The advent of percutaneous therapies has revolutionized our approach to treatment of valvular heart disease. The ability to perform such interventions safely and effectively relies heavily on anatomical details. Fluoroscopic anatomy alone is inadequate to guide valvular interventions due to poor visualization of the complex 3-dimensional valve unit. More advanced imaging technology is thus required to support fluoroscopy in guiding valvular interventions. Understanding valvular anatomy and correlating it real time with presented multimodality imaging is critical for procedural success in the catheterization laboratory.
In this chapter, we describe the anatomy of the 4 cardiac valves, applying it to fluoroscopic and multimodality imaging, to guide percutaneous valvular interventions for the cardiac interventionalist.
The heart is normally situated obliquely in the chest such that the interventricular and interatrial septa are approximately 45° to the anteroposterior projection (Fig. 4-1). Therefore, a 45° left anterior oblique (LAO) projection will allow distinction of the left and right structures, whereas a 45° right anterior oblique (RAO) projection will discriminate anterior and posterior structures. The planes of the mitral and tricuspid valves are virtually at right angles to the septal plane with some inferior tilt with the mitral plane situated slightly posterior to the tricuspid valve plane. In other words, LAO 45° caudal 15° may visualize the mitral and tricuspid valves en face, whereas RAO 45° caudal 15° may provide one of the side views for these valves. The inflow and outflow of the left ventricle are aligned closely with an angle of 15° to 20° in a normal heart, whereas the inflow and outflow of the right ventricle are aligned almost at 90°.
The aortic valve plane is typically tilted caudally and leftward, with the end on view being either LAO 60° caudal 35° or RAO 30° cranial 45°. Side views can be obtained with either RAO 20° caudal 20° or LAO 40° cranial 30° projections. Pulmonary valve is tilted quite posteriorly and to the right, such that the end on view is RAO 10° caudal 50°, and the pulmonary valve plane ranges from RAO 30° cranial 20° to LAO 30° caudal 20°. The right ventricle wraps around the outflow of the left ventricle, being mostly anterior to the left ventricle with only the inferior margin to the right of the left ventricle; the outflow tract of the right ventricle is superior and to the left of the aortic valve. The left atrium forms most of the posterior aspect of the heart as appreciated on the lateral fluoroscopic views.
The fibrous skeleton (Fig. 4-2) formed by the valve annuli and the intervening right and left fibrous trigones defines the plane of the atrioventricular groove and houses all 4 of the cardiac valves. The aortic valve has a central location in the fibrous skeleton and is closely related to the other 3 valves. The mitral valve (posterior medial commissure) and the tricuspid valve (septal leaflet) are intimately connected to the base of the noncoronary cusp of aortic orifice by a condensed mass of fibrous tissue, the central fibrous body, also referred to as the right fibrous trigone, which continues inferiorly as the membranous interventricular septum. The left fibrous trigone is another condensed mass of fibrous tissue that connects the anterolateral commissure of the mitral valve and the base of the left coronary cusp of the aortic valve. The pulmonary valve is located anterior and to the left of the aortic valve and has a separate fibrous ring that is connected to the base of the right coronary cusp of the aortic valve by a fibrous band referred to as the conus ligament.
Away from the central body, the fibrous skeleton becomes less robust with less discrete organization. The part of the fibrous skeleton bounded by the left and right trigones connects the anterior mitral leaflet with the noncoronary cusps of the aortic valve and is commonly referred to as the aorto-mitral curtain, intervalvular fibrosa, or subaortic curtain. Calcifications of the fibrous skeleton of the mitral and aortic annuli (mitral annular calcification) are of critical importance to the outcome of transcatheter aortic valve implantation (TAVI) with respect to symmetric deployment of the valve and resultant perivalvular leak.
The aortic root is a complex and dynamic ensemble of the aortic annulus, aortic valve leaflets, sinuses of Valsalva, the sinotubular junction, and the interleaflet fibrous triangles that connects the left ventricle to the ascending aorta. It is often described as resembling a crown (Fig. 4-3) that is transected by 3 horizontal planes.1,2,3 The top of the crown, represented by an imaginary plane that transects the ring-shaped sinotubular junction in a horizontal fashion, is formed by the peripheral attachments of the aortic valve leaflets near the commissures. The base of the crown is a virtual plane formed by horizontal transection of the basal attachments of the aortic valve leaflets. Between these 2 planes exists the true anatomic aortoventricular junction marked by the area over which the fibrous wall of the arterial trunk is attached to the supporting ventricular structures, forming the third imaginary horizontal plane of the aortic root. It is important to note that these planes are not necessarily parallel to each other due to the fact that there is reflection of left and right coronary cusps over the muscular septum.
The term annulus came into vogue to express a reference plane for valve sizing and deployment during surgical aortic valve replacement. However, despite the widespread use of this term, there is no distinct anatomic or histologic circular structure that defines it. Surgically, the annulus is crown shaped and is represented by the semilunar lines of attachment of the aortic valve leaflets to the aortic root.1 Echocardiographers, however, measure the annulus along the virtual basal plane of the crown in the left ventricular outflow tract at the inferior-most aspect of the aortic valve leaflet hinge points.2
Annular measurements are routinely performed in the parasternal long-axis view obtained on 2-dimensional (2D) transthoracic echocardiography (TTE) and rarely in the apical 5-chamber view (Fig. 4-4). The aortic annulus on transesophageal echocardiography (TEE) is measured in the 125° to 140° long-axis view of the left ventricle (see Fig. 4-4). This measurement is taken from the inner edge of the septal endocardium to the inner edge of the anterior leaflet of the mitral valve at the hinge point of the aortic valve leaflets in mid-systole.4 More recently, multidetector computed tomography (MDCT) has become the gold standard for annular assessment.5,6 Annular measurements using MDCT can be obtained as either a hinge-to-hinge measurement on a single oblique view as measured on TTE/TEE or can be derived from the area or the circumference of the virtual basal ring (Fig. 4-5). There are several important points about the measurement of the “annulus.” The annulus is not circular but elliptical, and therefore, different modalities that measure different planes of the annulus do not necessarily yield same results.5 The annular size obtained by MDCT is typically larger than when measured by 2D TTE/TEE. When the diameter of the annulus is measured solely by 2D methods (typical for TEE or TTE), special care has to be taken to get the maximal diameter because symmetric opening of the leaflets (where typically the views are obtained) does not necessarily provide the largest diameter.2
FIGURE 4-5
Aortic annular measurement on cardiac computed tomography. Hinge points of all 3 cusps are identified in both the Sagittal oblique plane (top row) and Coronal oblique plane (middle row). Reconstructed double-obliqued axial plane or transverse plane (bottom row) is then used to measure the maximal, minimal, and mean annular diameter and the annular area and perimeter.

The aortic valve is normally comprised of 3 semilunar leaflets or cusps of nonuniform size (Fig. 4-6). The leaflets derive their name from the associated coronary artery (right coronary cusp, left coronary cusp, and noncoronary cusp) that arises near the cusp. Each leaflet has a free margin and a margin that is attached peripherally to the aortic root in a semilunar fashion. Each leaflet also has a ventricular surface that is smooth and an arterial surface that is rougher in nature. At the center of the free margin of the leaflets is a thickened nodule called the nodule of Arantius.7 The remainder of the free margin extending from the nodule of Arantius to the aortic attachment is called the lunule. Coaptation of the leaflets occurs at the nodule of Arantius and a thicker closing surface 1 to 2 mm below the lunule. Therefore, fenestrations, although common in the lunule, are not associated with valvular insufficiency in the normal aortic valve.7 However, if dilation of the aortic root moves the line of valve closure toward the lunule, aortic insufficiency can result from these lunular fenestrations. The lunules of adjacent leaflets are attached to each other for a very short segment at their bases to form apposition lines referred to as commissures.
Functionally, the leaflets separate the aorta from the left ventricle during ventricular diastole and represent the hemodynamic ventriculoarterial junction.
The sinotubular junction is a circular ridge of fibroelastic tissue at the apices of leaflet attachment to the aortic wall.2 Anatomically, the sinotubular junction marks the transition point from the aortic root to the ascending aorta. Dilation of the sinotubular junction leads to aortic insufficiency. Severe calcification of the sinotubular junction can be problematic for balloon-expandable TAVI. If the calcification is circumferential and the sinotubular junction diameter is smaller or comparable to the diameter of the annulus, there is some risk of “watermelon seeding” of the balloon in the ventricular direction at the time of valve deployment.8 Precariously, this can lead to severe aortic insufficiency or even valve embolization into the left ventricle.
The expanded portion of the aortic root on the arterial side of the aortic valve leaflets is called the sinus of Valsalva. It is bound distally by the sinotubular ridge and proximally by the lower most attachment of the aortic valve leaflets. There are 3 sinuses, each named for the coronary artery to which it is associated (right coronary, left coronary, and noncoronary). The sinuses prevent coronary obstruction by housing the valve leaflets and generating eddy currents that further hold the leaflets away from the aortic valve during systole.9 The eddy currents also play a role in valve closure and stress reduction of the aortic valve leaflets during diastole.9
The geometry of the aortic sinus has important implications for valve choice and size during transcatheter aortic valve replacement (TAVR). Important measurements at the aortic root include height and width of the sinus of Valsalva, take off height of the coronary ostia, and ascending aortic diameter (Fig. 4-7). These measurements are crucial to prevent coronary ostial obstruction following TAVR.10,11
A unique aspect of the noncoronary sinus is that it rests on the interatrial septum. When doing a transseptal puncture, visualization of this sinus by contrast injection or catheter placement in the sinus is critical to avoid puncturing the aorta.
Semilunar attachment of the aortic leaflets creates 3 triangular areas under each commissure called the interleaflet fibrous triangles.9 They are an extension of the left ventricular outflow tract to the sinotubular junction. The triangle between the left coronary sinus and noncoronary sinus is continuous with the aortomitral curtain. The triangle between the noncoronary and the right cusp is in continuity with the membranous septum. The third triangle between the left and right coronary cusps attaches to the muscular septum.
Familiarity with anatomic relationships of the aortic valve to the 4 cardiac chambers and to the vascular and conduction system is important to the cardiac interventionalist in the current era of transcatheter therapies for structural heart interventions.
As described earlier, the noncoronary sinus rests on the interatrial septum. Visualization of this sinus by contrast injection or catheter placement in the sinus is critical to avoid puncturing the aorta during transseptal puncture. When using fluoroscopy for transseptal puncture, the location of the fossa ovalis is “guesstimated” based on the location of the posterior boundary of the aortic root as marked by a pigtail catheter positioned in the noncoronary aortic cusp combined with bony landmarks. The classic fluoroscopic landmark for the puncture site is in the center of the spine, at the level of the aortic root.12 To avoid aortic perforation during transseptal puncture, the dilator tip should be visualized below the pigtail catheter placed in the noncoronary cusp in the 30° LAO view and posterior to the pigtail catheter in the 30° RAO view.
Coronary obstruction after TAVR is a rare but serious procedural complication that is potentially avoidable with meticulous preprocedural aortic root analysis. A low coronary ostial takeoff or, more commonly, a low takeoff in combination with a narrow aortic root and bulky valve calcification predisposes to coronary ostial obstruction by displacement of the calcified native cusp over the coronary ostium.10,11,13 In the setting of a narrow sinus of Valsalva, the bulky calcified leaflets cannot be accommodated and invariably get pushed over the ostia of the coronary arteries and have been observed to cause coronary obstruction. A displaced calcified native leaflet is more likely to close the origin of the left main coronary artery than the right coronary artery due to a normal higher right coronary artery ostia takeoff. Furthermore, aorto-mitral continuity via the intervalvular fibrous skeleton allows complete straightening of the left coronary leaflet, placing the left coronary ostium at a higher risk. The interventricular septum, however, anchors the right coronary leaflet and limits its upward movement, an important factor that reduces the risk of right coronary artery ostial occlusion. A sinus of Valsalva width of 27 mm (for the 26-mm CoreValve) or 28 mm (for the 29-mm CoreValve) and a coronary height of 14 mm are recommended by the manufacturer for the implantation of the CoreValve system (Medtronic, Edgewater, MD).10 There are no specific manufacturer recommendations for the Edwards valve (Edwards Lifesciences, Irvine, CA), but a cutoff of 10 mm for the coronary height is widely practiced.
Careful consideration should be given prior to stenting the left main trunk in patients intended to have a TAVI. In the absence of beveled stents, the inferior aspect of the left main stent often extrudes into the aortic lumen. It is conceivable that a percutaneously placed valve may crush the native valve leaflets against a protruding left main stent. If left main percutaneous intervention is undertaken in such patients, precise stent placement to avoid “hanging out” into the aorta is paramount. Shallow LAO or anteroposterior (AP) cranial projections (instead of the typical LAO caudal or AP caudal views) may be useful to allow conservative stent positioning.
The His bundle and the fascicles of the left bundle are closely related to the aortic root. Conduction system disturbances and permanent pacemaker requirements are thus common following TAVR. The His bundle, a continuation of the atrioventricular node, penetrates the central fibrous body, runs between the membranous septum and the muscular septum, and bifurcates at the crest of the muscular ventricular septum into the left and right bundles. At this location, the left bundle is intimately related to the base of the interleaflet triangle between the right and noncoronary cusp of the aortic valve. In autopsy specimens, the exit of the left bundle has been identified to be 6.3 ± 2.4 mm from the noncoronary cusp of the aortic valve, making it particularly vulnerable to disruption with a lower placement of a percutaneous aortic valve.14 The superficial course of the left bundle as it descends along the left interventricular septum further increases its susceptibility to injury with even minor trauma. Conduction disturbances are more common after the CoreValve compared to the Edwards valve and are likely related to the frame characteristics and mechanism of implantation.15
Three basic types of aortic stenosis are recognized: (1) degenerative calcific aortic stenosis; (2) congenitally bicuspid aortic stenosis; and (3) rheumatic aortic stenosis (Fig. 4-8). Degenerative calcific aortic stenosis is present in approximately 2% to 3% of the population over the age of 65 and approximately 4% of patients over the age of 85.16 Nearly one-third (29%) of patients have calcific aortic sclerosis without stenosis.16 Degenerative calcific aortic stenosis represents the majority of patients over the age of 70 undergoing traditional aortic valve replacement. Congenitally bicuspid aortic stenosis is the most common etiology of aortic stenosis in younger patients and has been reported to occur in 1% to 2% of the population. Adjacent cusps fuse to form a single aberrant cusp, larger than its counterpart yet smaller than 2 normal cusps combined.3 The hemodynamic perturbations associated with the bicuspid leaflets in combination with fibrillin-1–deficient microfibrils are responsible for the premature loss of the structural integrity of the valve as well as premature degeneration of the aortic media.3 As such, bicuspid aortic stenosis is often associated with dilatation of the aortic root and aneurysm formation. These patients typically present in their fifth and sixth decades of life with symptomatic aortic stenosis with or without a dilated aortic root and present a unique challenge for percutaneous valve therapy. Large annular diameter, dilated aortic sinuses, and eccentric leaflet calcification often translate into suboptimal candidacy for TAVI. Even crossing the bicuspid valve can be difficult in these patients; an AL-1 catheter and a straight 0.35-inch wire can be used to accomplish this occasionally arduous task. If the left coronary leaflet is involved in the fusion (left-right or left-non), an RAO projection is often helpful in crossing these valves, whereas a traditional LAO projection is most useful for crossing valves with fusion of the right and noncoronary leaflets. Classical rheumatic aortic valve stenosis is associated with an abnormal mitral valve (diffuse leaflet fibrosis, usually stenotic) and an aortic valve characterized by commissural fusion of at least 1 and generally 2 or 3 commissures.
Three basic types of aortic regurgitation have been recognized17 (see Fig. 4-8): type I, normal leaflets with aortic root dilation or leaflet perforation; type II, leaflet prolapse or fenestration; and type III, leaflet restriction or extensive leaflet calcification. Primary aortic regurgitation with noncalcified aortic root is typically considered a contraindication for TAVR due to the risk of valve dislocation as a consequence of insufficient anchoring. However, recent advances in valve technology have expanded percutaneous catheter-based therapies to pure aortic regurgitation.18
Pivotal to performing TAVI and other structural heart interventions is a fundamental understanding of fluoroscopic anatomy. Two basic views commonly used for fluoroscopic imaging of the aortic valve are RAO 30° and LAO 60°.19 In the RAO view, the right coronary cusp is the most anterior located cusp (closest to the anterior wall of the left ventricle); conversely, the noncoronary cusp is the most posterior located cusp (closest to the inferior wall of the left ventricle) (Fig. 4-9). The left coronary cusp lies between the noncoronary cusp and right coronary cusp in the RAO projection. Conversely, in the LAO projection, the left coronary cusp is well visualized (closest to the lateral wall of the ventricle), whereas the noncoronary cusp and right coronary cusp are superimposed on one another, with the noncoronary cusp lying slightly below the right coronary cusp (see Fig. 4-9). A pigtail catheter in the noncoronary cusp can be clocked to enter the right coronary cusp and counterclocked to enter the left coronary cusp.
FIGURE 4-9
Fluoroscopic image of the heart and corresponding transesophageal electrocardiography images are shown. Mitral and aortic valve details are outlined. A1, first scallop of the anterior mitral leaflet; A2, second scallop of the anterior mitral leaflet; A3, third scallop of the anterior mitral leaflet; AML, anterior mitral leaflet; AO, aorta; IAS, intra-atrial septum; LA, left atrium; LAA, left atrial appendage; LCC, left coronary cusp; LMT, left main trunk; LV, left ventricle; NCC, noncoronary cusp; P1, first scallop of the posterior mitral leaflet; P2, second scallop of the posterior mitral leaflet; P3, third scallop of the posterior mitral leaflet; PA, pulmonary artery; PML, posterior mitral leaflet; PV, pulmonic valve; RA, right atrium; RCC, right coronary cusp; RV, right ventricle; TV, tricuspid valve. (Reprinted from Kapadia SR, Schoenhagen P, Stewart W, et al. Imaging for transcatheter valve procedures. Curr Probl Cardiol. 2010;35:228-276, with permission from Elsevier.)

Achieving a uniform alignment of the coronary cusps for TAVI typically requires slight caudal angulation for the RAO view and slight cranial angulation for the LAO view. Kasel and colleagues20 have a proposed a rule—“follow the right cusp”—to obtain the perpendicular implantation view on fluoroscopy. After identification of the right coronary cusp, the implantation angle can be obtained by rotating the C-arm to an RAO or LAO angulation if the right cusp is placed behind the noncoronary cusp or the left cusp, respectively, and/or rotating the C-arm cranially or caudally if the right cusp appears higher or lower than the ideal plane intercepting other cusps, respectively.
Fluoroscopy is also important for recognizing other important anatomic considerations for TAVI such as curvature of the aortic arch, aortic valve calcification, calcification of the sinotubular junction, aortoiliac calcification and diameter, and movement of equipment through the aortoiliac system.
At times, echocardiographic visualization of the aortic valve is difficult, and MDCT, with its higher spatial resolution, allows for detailed evaluation and recognition of the valvular architecture. Several anatomic issues can be addressed by MDCT including anatomy of the aortic valve (eg, bicuspid), aortic valve area, severity and location of aortic root calcification, annular measurements, sinus of Valsalva measurements, and location of the coronary ostia, sinotubular junction assessment, and aortic root orientation in relation to the body axis.21 Anatomic assessment of the aortic root and iliofemoral arteries using MDCT has thus become a standard practice prior to TAVI.5,22
Bicuspid aortic valves are present in 1% to 2% of the population and are the second most common etiology of aortic stenosis. They can be congenital or acquired. In the congenital bicuspid aortic valve, unlike the acquired variant, the cusp containing the false commissure (raphe) (conjoined cusp) is of similar length to that of the other cusp. The bicuspid aortic valve exists in 2 major forms: a right-left (fusion of the noncoronary cusp and either the left or right cusp) and an AP (fusion of the right and left cusp) orientation of the 2 cusps. These structural changes in the bicuspid aortic valve straighten the line of lateral attachment, limiting the mobility of the valve and subjecting the valve to hemodynamic perturbations that facilitate premature degeneration and calcification of the valve. Bicuspid aortic valve is generally considered a contraindication to TAVR because of the theoretical risk of poor seating, coronary ostial obstruction, or paravalvular regurgitation from the distorted geometry of the aortic root. However, clinical experience with TAVR, although limited in this group of patients, has been promising.
Using planimetry, the aortic valve area can be measured on MDCT. Aortic valve area assessment by TTE, however, uses the continuity equation. Therefore, MDCT evaluates anatomic valve area instead of the effective area, which is evaluated by TTE. Therefore, anatomic valve area measured by MDCT is larger than that measured by TTE.23,24,25,26 Incorporation of computed tomography (CT)-derived left ventricular outflow tract measurement into the continuity equation has been shown to improve TTE aortic stenosis severity assessment.
Age-related degenerative changes in the aortic valve are those of progressive calcification and fibrosis27 (intrinsic and extrinsic cusp calcification). Two patterns of calcification have been described: (1) the coaptation pattern, where calcification occurs along the line of cusp coaptation; and (2) the radial pattern, where calcification occurs as spokes of a wheel, spreading from the peripheral cusp attachments toward the center. However, in advanced stages, the calcification pattern becomes unidentifiable.28 Aortic valve calcification can be scored both qualitatively and quantitatively by cardiac CT. Aortic valve calcification is qualitatively assessed as follows: grade 1, no calcification; grade 2, mildly calcified (small isolated spots); grade 3, moderately calcified (multiple larger spots); and grade 4, heavily calcified (extensive calcifications of all cusps) (Fig. 4-10). Extensive aortic root calcium precludes optimal valve implantation and identifies patients at risk for significant paravalvular leak after TAVR.29
MDCT is regarded as the gold standard for aortic annulus measurement.30,31,32 In the reconstructed axial images of the aortic root, the following 3 measurements are made immediately below the most caudal attachment of the 3 aortic leaflets to measure the annulus: (1) measurement of the long and short diameters of the oval aortic annulus to calculate the mean diameter; (2) area-derived diameter (planimetry of the area of the aortic annulus and calculation of the diameter that corresponds to this area under the assumption of full circularity); and (3) perimeter-derived diameter (measurement of the circumference of the aortic annulus and calculation of the diameter that corresponds to this length again under the assumption of full circularity).
In addition to evaluation of the aortic valve and annulus, many other parameters are ascertained during the acquisition of cardiac CT that are routinely used in planning of TAVR, including aortic dimensions, coronary ostial height, sinus of Valsalva, and sinotubular junction diameters.33
Echocardiography provides excellent temporal and spatial resolution for investigating not only the anatomy but also the function of the aortic valve. Identification of various anatomic relationships in different transthoracic and transesophageal views is critical for planning and guidance of structural interventions (Fig. 4-11). Two-dimensional Doppler TTE is the most commonly used tool for assessing the severity of aortic stenosis. This can be accomplished by estimating the valve area by planimetry or using the continuity equation, measuring the peak transvalvular velocity and the peak and mean pressure gradients across the valve.34 Peak pressure gradient can be calculated from the peak velocity using the Bernoulli equation (ΔP = 4V2, where ΔP = pressure gradient and V = flow velocity in m/s at the site of obstruction). Aortic valve area is estimated by the continuity equation using Doppler-derived transvalvular velocities (TVIs) in conjunction with anatomic measurements of the left ventricular outflow tract (LVOT; Aortic valve area = LVOT × LVOT VTI/aortic valve VTI).35
In the presence of aortic valve regurgitation or significant left ventricular (LV) dysfunction, measuring aortic valve gradient alone is inadequate to determine the severity of aortic stenosis. In these situations, aortic valve area should also be measured because the continuity equation is reliable even in the presence of aortic and mitral insufficiency and independent from transaortic flow.36
Many indices have been investigated for determining the severity of AS, namely, dimensionless index, jet width at the aortic valve level, valve resistance, percent LV stroke work loss, and modified ventricular-vascular coupling. However, none of these adjunctive measures have proven to more reliable than transvalvular gradient, aortic valve area, and jet velocity. As such, current guidelines define the severity of aortic stenosis based on these parameters.35
Dimensionless index is the ratio of the systolic velocity flow integral in the LVOT to the systolic velocity integral in the aortic jet and may be useful in the setting of severe LV dysfunction. Dimensionless index <0.3 is consistent with severe aortic stenosis and has a high sensitivity and specificity for diagnosis of severe aortic stenosis.37 Not surprisingly, TTE has limitations; poor acoustic windows and severe calcification of mitral and aortic annulus may lead to inaccurate measurement of LVOT diameter and aortic valve gradients translating to erroneous calculations of aortic valve area.38 Nonparallel interrogations of jet velocity may lead to underestimation of the severity of stenosis, due to the higher central velocity of aortic flow through the valve.39 In the presence of mitral regurgitation, irregular heart rhythms, or subvalvular or supravalvular stenosis, transvalvular velocities can be overestimated.40 Low cardiac output or significant LV dysfunction may lead to underestimation of aortic stenosis severity.
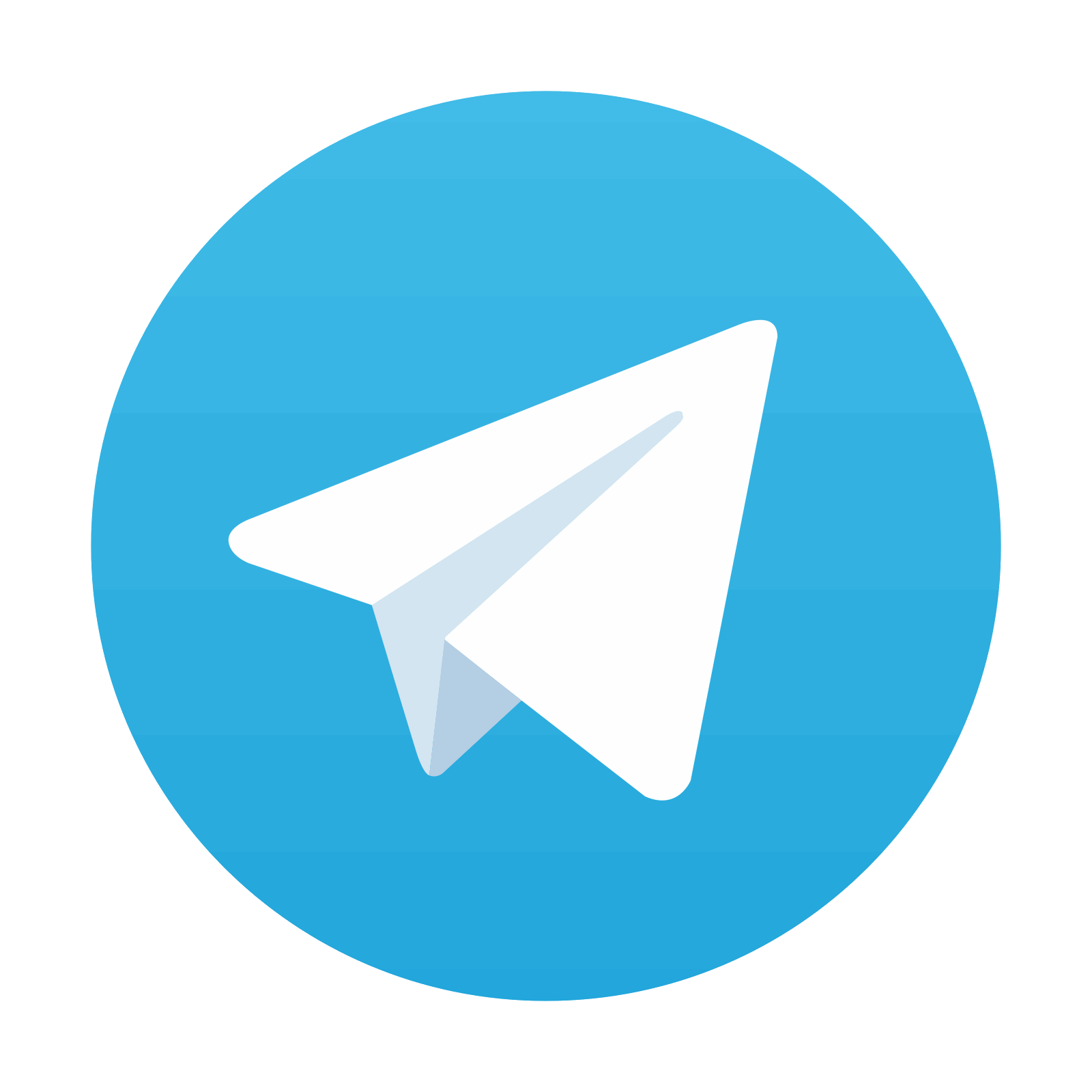
Stay updated, free articles. Join our Telegram channel

Full access? Get Clinical Tree
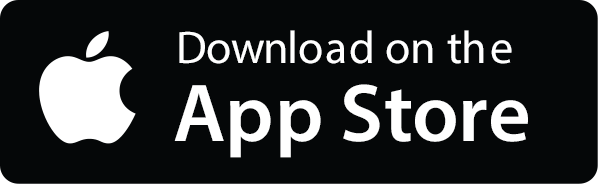
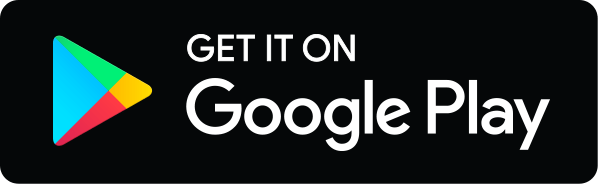