Anatomy and Function of the Carotid and Vertebral Systems
THE BRANCHES OF THE AORTIC ARCH (SUPRAAORTIC TRUNKS)
![]() PLATE 1.1. Fragment of Las Dos Fridas, with Frieda Kahlo’s (1907 to 1954) interpretation of the branches of the aortic arch. |
Anatomy and Variations
The normal sequence (82%) for the takeoff of the branches of the aortic arch (Fig. 1.1) is for the innominate artery (IA) and left (Lt) common carotid artery (CCA) to exit first in the anterior mediastinum and for the Lt subclavian artery (SA) to arise from the distal arch in the posterior mediastinum. The distance between the origin of the Lt CCA and Lt SA (1 to 2 cm) is usually greater than that between the IA and the Lt CCA.
The most common variation (10%) of the aortic arch branches is for the Lt CCA to take origin more proximally in the arch, either sharing the ostium with the IA or arising from the IA itself. The incidence of this variant is higher among blacks than in whites. This variant is relevant to the surgeon because clamping or doing an angioplasty of such a configuration will involve necessarily the blood supply to both hemispheres.
Another variation (10%) is a V-shaped origin of both CCAs from a single ostium, or from a short common bi-carotid trunk, before they separate to go to each side of the neck. This variation, present in patients who have an anomalous right (Rt) retroesophageal subclavian artery (rSA), is incorrectly designated as a “bovine” pattern.1,i
A third variation is the Lt vertebral artery (VA) arising from the aortic arch between the origins of the Lt SA and Lt CCA. This occurs in 7% of the people and, as we will see later (see Segment V1: The extraforaminal course p.19), this variation requires specific considerations during operations in the Lt SA and Lt VA.
When the IA takes off from the aorta more distally, to the left of the trachea, it must cross over the latter to reach its destination in the right side of the neck. The compression of the IA over the trachea results in different problems depending on which of the two elements is stiffer. Newborns with their soft, collapsible tracheas may experience severe respiratory distress if the IA compresses them. In most newborns, the IA, or some part of it, exits the arch of the aorta to the left of the trachea. By the age of 3 years, the growth of the aortic arch will have situated the origin of the IA to the right of the trachea and so it does not cross the latter. Babies with bouts of apnea or recurrent tracheobronchitis that do not respond to medical management will need surgical correction, usually transposition of the origin of the IA to a more proximal site in the arch. Conversely, patients that have a permanent tracheostomy cannula, or are undergoing long-term endotracheal intubation, may experience catastrophic bleeding when the posterior wall of the IA is eroded by the stiffened, cannulated trachea (aorto-IA fistula).
Clinical Implications of Aortic Arch Anomalies
A Rt rSA is present in 0.8% of the population (Fig. 1.1). Individuals with this anomalous artery have all or some of the following associated anomalies: a right-sided thoracic duct, a non-recurrent right inferior laryngeal nerve, and a common origin for both CCAs (Fig. 1.3). The Rt VA may arise in these patients from the Rt SA, the Rt CCA, or the IA.
The Rt rSA has an abnormal architecture and, like other persistent embryonic arteries (such as the sciatic, trigeminal, and so forth), has a propensity to degenerate early into atherosclerotic or aneurysmal disease. Aneurysms of the rSA can reach enormous size (Fig. 1.4) and may rupture into the esophagus. A Rt rSA may originate
from a normal appearing descending aorta or, more frequently, from an outpouching of the aortic wall called a diverticulum of Kommerell. The aortic wall around the opening of the Kommerell’s diverticulum also has an abnormal structure and is often dilated above and below the opening of the diverticulum. This dilation may extend to the neighboring Lt SA (Fig. 1.5).
from a normal appearing descending aorta or, more frequently, from an outpouching of the aortic wall called a diverticulum of Kommerell. The aortic wall around the opening of the Kommerell’s diverticulum also has an abnormal structure and is often dilated above and below the opening of the diverticulum. This dilation may extend to the neighboring Lt SA (Fig. 1.5).
In a previous publication,2 I had described the direct closure of the Kommerell diverticulum by an intralumenal patch sawn through an anterior aortotomy. This turned out to be a bad surgical solution. Over the years, patients show dilation of the aortic wall around the patch that closed the origin of the diverticulum. The current treatment of a diverticulum of Kommerell, covering its opening as well as the adjacent aortic wall with an endograft, is a far better solution, one that not only obliterates the opening of the diverticulum but also protects the neighboring aorta from further dilation. The coverage of the Kommerell opening is complemented by the transposition of the Rt SA to the Rt CCA through a supraclavicular approach.
Individuals with a right-sided aortic arch display mirror images of the arrangements seen in normal left-sided arches. If they have a retroesophageal artery, it would be the left one (Fig. 1.6). Rt-sided arches may coexist with agenesis of the Lt-sided IA (Fig. 1.7A) that will result in a Lt subclavian steal (Fig. 1.7B). A similar steal is present in patients with an isolated Lt SA that is not connected to the aorta (Fig. 1.8). Including the more frequent variations described above, a total of 25 different arrangements have been reported for the branches of the arch.
![]() FIGURE 1.7. (A) Early injection phase of a Rt-sided arch with agenesis of the IA. (B) Late phase showing a vertebral and common carotid steal. |
The Phrenic Nerve and the Subclavian Artery
The following considerations regarding the anatomy of the phrenic and accessory phrenic nerves (aPNs) are pertinent to the finding of an elevated hemidiaphragm following operations on the SA. An aPN exists in 68% of individuals and its trajectory varies widely. An aPN may originate from the nerve to the subclavius muscle (containing fibers from C5 and C6) or from the ansa cervicalis.3 aPNs are more frequent on the right side (Fig. 1.9) and 90% of them are lateral to the PN.
Injury to the PN or to the aPN results in paralysis of a hemidiaphragm. Injury to the aPN is one of the reasons for the surprisingly high incidence (between 10% and 85%) of hemidiaphragmatic palsy following cardiac surgeryii and for those cases where the palsy is found after subclavian venipuncture or supraclavicular nerve block.4, 5, 6
There are nerve loops around the subclavian vein and the internal mammary artery linking the PN and the aPN that may be injured during venipuncture and during high dissection of the internal mammary artery for coronary revascularization.
This variable topography of the aPN is the likely explanation for the finding of hemidiaphragmatic palsy following operations on the SA even when the integrity of the main PN was noted by the surgeon. The corollary is that any nerve that is seen joining the PN in the surgical field should be preserved.
THE CAROTID ARTERY
Trajectory and Histology
Arteries and veins do not have midline symmetry. The branches of the arch are asymmetric, the left and the right carotid may bifurcate at different levels, and carotid atheroma does not develop at the same rate bilaterally. The detailed layout of our blood vessels is not encoded in our genomeiii but is believed to result from the interaction of vessel precursors with local factors.
The tortuosity of the CCA, internal carotid artery (ICA), and external carotid artery (ECA) has been attributed to age and hypertension although some observers that have noted ICA tortuosity in newborns have questioned this explanation. There is no denying, however, that the neck arteries we image in young people are straighter than those of older folks.
The CCA diameter increases with age and with atherosclerotic risk factors. As the CCA dilates with age, it must also elongate.iv Furthermore, the distance between the aortic arch and the skull base bridged by the CCA and ICA shortens with age due to shrinking of the intervertebral disks and increased lordosis of the cervical spine. The carotid arteries accommodate to this shorter distance by becoming tortuous.
Carotid coiling on the other hand (Fig. 1.10) is an embryological development and is not a consequence of vascular remodeling secondary to aging, hypertension, or atherosclerosis. Beigelman7 showed in an extensive survey of 885 participants (from infants to nonagenarians) that coiling is not correlated with increasing age.
The carotid bifurcation is a junction of arteries with different mechanical properties the result of the different composition of their tunica media. The branches of the arch (IA, CCA, and SA) are elastic arteries. The CCA media is mostly elastin and collagen, as is the case with most conduction arteries. Conversely, the media of the ECA and ICA, both muscular arteries, is mostly smooth muscle with little elastin or collagen network. This gives the ECA and ICA the capacity to contract into spasm, as we see in the operating room when dissecting or stretching them.
The transition zone between the elastic CCA and the muscular ICA is at the origin of the ICA and extends distally for a length of 10 mm. The carotid baroreceptors,
located in this transitional zone, sense blood pressure by transducing their stretch. Most carotid atheromas develop in this transitional zone.
located in this transitional zone, sense blood pressure by transducing their stretch. Most carotid atheromas develop in this transitional zone.
The bulb itself has a thick adventitia8 (0.6 mm) and a thin media (0.2 mm) composed mostly of elastic fibers. At about 8 mm from the flow divider, the media doubles its thickness as layers of smooth muscle begin to appear. Sympathetic fibers run in the outer layer of the ICA and may be stretched and disrupted by the intramural hematoma of a dissection. This accounts for the high incidence (25% to 40%) of Horner’s syndrome that is seen in patients with ICA dissection.
Internal Carotid Artery Aplasia
The entire ICA may be missing (agenesis) (Fig. 1.11) or there may be a normal size bulb and a thread-like or absent (aplasia) ICA distal to it. The opposite carotid or the VA that supply the territory of the absent ICA will be consequently hypertrophic.
Since the embryo’s skull base develops 2 weeks after the ICA forms, patients with carotid aplasia do not have a carotid canal in the temporal bone (Fig. 1.12) and individuals with carotid hypoplasia have a correspondingly smaller carotid canal.
ICA aplasia (est. incidence 0.01%) is usually found in patients who are undergoing angiography for the workup of intracranial aneurysm: the incidence of intracranial aneurysm is 30% higher in patients who have a hypoplastic/aplastic ICA.9
The high incidence of intracranial aneurysm in patients with ICA aplasia has been attributed to (1) increased circulatory load in the contralateral ICA and basilar artery (BA)
or (2) a synchronous error in development occurring at the fourth to fifth week of embryonic stage. But the fact that the associated intracranial aneurysms present more frequently on the same side as the aplastic ICA, in intracranial arteries that are not subjected to abnormally increased flow, makes the second explanation the likely one to be true. This explanation is further supported by the fact that patients with ICA aplasia often show other concurrent abnormalities (ventricular septal defect, hemangiomas) that are also believed to have occurred at about the same time (fourth to fifth week) that the ICA develops.
or (2) a synchronous error in development occurring at the fourth to fifth week of embryonic stage. But the fact that the associated intracranial aneurysms present more frequently on the same side as the aplastic ICA, in intracranial arteries that are not subjected to abnormally increased flow, makes the second explanation the likely one to be true. This explanation is further supported by the fact that patients with ICA aplasia often show other concurrent abnormalities (ventricular septal defect, hemangiomas) that are also believed to have occurred at about the same time (fourth to fifth week) that the ICA develops.
The Carotid Bifurcation
Carotid bifurcation atheroma is often asymmetric. Given that the arterial wall and its cellular components are exposed to the same humoral elements in both sides, it must be that differences in geometry determine the appearance of atheroma on one side or its absence on the other. The interaction of the flow stream with the geometry of the walls of the bifurcation determines wall shear stress, wall metabolism, the rate of local platelet aggregation, and factors that may enhance or inhibit the development of an atheromatous plaque. Because of this, some considerations are in order regarding the geometry of the bifurcation and what effects it has on these variables.
The CCA bifurcates at the same level only in 28% of individuals. The bifurcation is higher on the right side in 22% of individuals and in 50% is higher on the left. The CCA bifurcation may occur low in the neck, in rare cases as low as the upper mediastinum.
Measurements taken in the angiograms of a large cohort of patients show large inter- and intra-individual variation differences in the geometry of the carotid bifurcation.10 The area ratio (β)v of the carotid bifurcation is 1.14 in newborns,11
remarkably close to the ideal area ratio (1.15) that the physics of flow predict for an ideal bifurcation that would minimize the development of pressure gradients and result in minimal wave reflection. The area ratio of the carotid bifurcation determines what pressure drops will take place as the single flow stream of the CCA branches out (ECA and ICA) and how much of the pressure wave will reflect and bounce back upstream. This area ratio of the carotid bifurcation may vary as much as fourfold between individuals. In addition, there is also considerable intra-individual variation in geometry between the right and left sides.10
remarkably close to the ideal area ratio (1.15) that the physics of flow predict for an ideal bifurcation that would minimize the development of pressure gradients and result in minimal wave reflection. The area ratio of the carotid bifurcation determines what pressure drops will take place as the single flow stream of the CCA branches out (ECA and ICA) and how much of the pressure wave will reflect and bounce back upstream. This area ratio of the carotid bifurcation may vary as much as fourfold between individuals. In addition, there is also considerable intra-individual variation in geometry between the right and left sides.10
The peculiar geometry of the carotid bifurcation, with the bulbous origin of the ICA, has been a subject of interest to those who study the relationship between the flow mechanics of the bifurcation and the tendency for development of atheroma in it. It is assumed that a lower wall shear stress on the sinus wall creates the physical conditions that enhance the risk of plaque development. Mathematical models suggest that the larger the angle formed by the bifurcation, the lower will be the shear stress on the sinus wall and hence the higher the risk of atheroma formation.12 The bifurcation angle is larger in older people than in youngsters, perhaps a factor in the preferential development of atheroma in older individuals. Other studies using computational flow dynamics (with data derived from magnetic resonance [MR]13) showed that some simple geometric features of the carotid bifurcation, such as the area ratio and degree of tortuosity, are good predictors of the site and rate of deposition of atheroma in the bifurcation. The numerical flow studies also show that turbulence and wall sheer stress gradients are greatly reduced when the origin of the ICA tapers its diameter gradually and does not have a bulbous dilatation,14 which is, incidentally, the geometry achieved by a Type 1 eversion carotid endarterectomy (CEA), when some length of ICA is removed to correct preexisting elongation.
The first reference to the bulbous dilatation of the first segment of the ICA is from Allan Burns15 who, in 1811, noted the presence of this dilatation “independently of any organic disease of the coats [of the artery].” Boyd16 correctly concluded in 1937 that “changes in pressure of the general circulation are magnified at the dilatation and, consequently, the nerve-endings of the afferent nerves [in its wall] are able to register these changes in pressure more readily.”
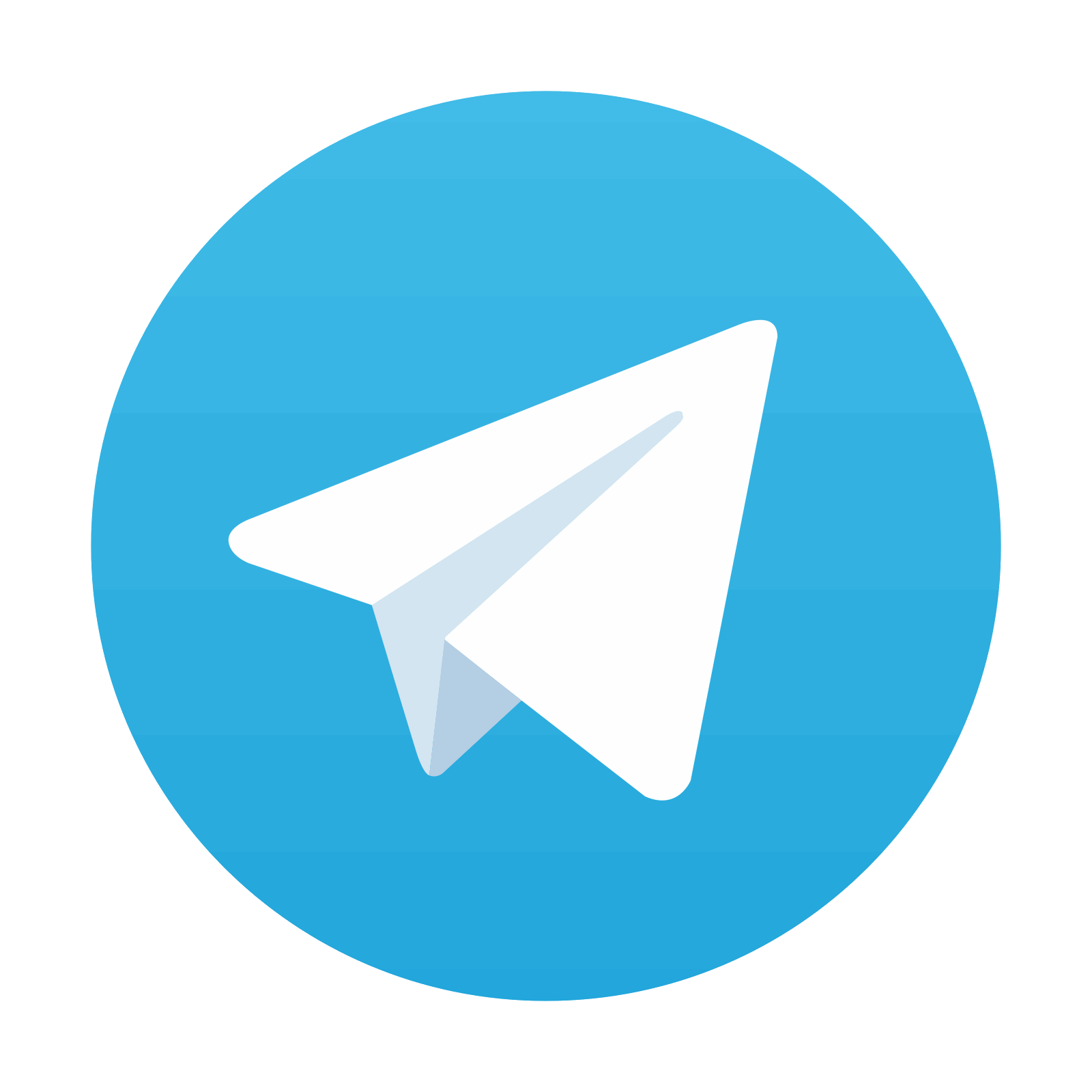
Stay updated, free articles. Join our Telegram channel

Full access? Get Clinical Tree
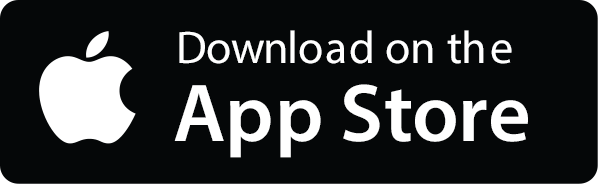
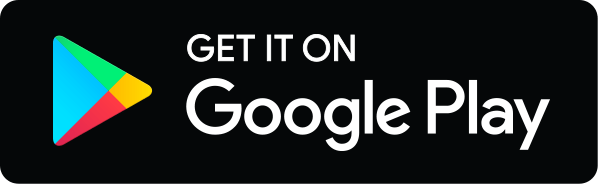