The purpose of this study was to establish the role of hormonal anabolic deficiencies in exercise intolerance in patients with chronic heart failure One hundred four consecutive men (mean age 53.1 ± 10.6 years) with established diagnoses of chronic heart failure were included. At enrollment, blood samples were taken, and echocardiography and cardiopulmonary exercise testing were carried out. Exercise capacity was expressed as peak oxygen consumption (V o 2 ), predicted peak V o 2 , and the ventilatory response to exercise (VE/V co 2 ) slope. The mean left ventricular ejection fraction was 29.7 ± 11.9%, and most patients (86%) were in New York Heart Association class I or II, with a mean peak V o 2 of 18 ml/min/kg. According to the age-adjusted reference values, hormonal deficiencies were present in 29% for total testosterone, 39% for estimated free testosterone, 34% for insulin-like growth factor–1, and 61% for dehydroepiandrosterone sulfate. Dehydroepiandrosterone sulfate showed a significant correlation with peak V o 2 (r = 0.29, p = 0.007), predicted peak V o 2 (r = 0.28, p = 0.006), and VE/V co 2 slope (r = −0.39, p <0.001), whereas total testosterone, estimated free testosterone, and insulin-like growth factor–1 were not significantly correlated. After adjusting in a multivariable model, dehydroepiandrosterone sulfate remained an independent predictor of each exercise parameter. In conclusion, in a cohort of patients with mild chronic heart failure, exercise capacity objectively measured using cardiopulmonary exercise testing was related to anabolic impairment of the adrenal rather than the somatotropic or peripheral axis.
Chronic heart failure (CHF) is a multiple metabolic and hormonal deficiency syndrome with anabolic-catabolic imbalance. The impairment of the 3 major anabolic axes (adrenal, gonadal, and somatotropic) is widely present in men with CHF and is associated with greater severity of symptoms, and more important, it represents a powerful predictor of poor outcome. Functional impairment is a hallmark in the severity of heart failure and has long been recognized as a prognostic marker of the disease. Cardiopulmonary exercise testing has become an important clinical tool to objectively evaluate exercise capacity and predict outcomes in patients with CHF. Recently, Jankowska et al demonstrated that the gonadal axis, reflected by a reduction of circulating testosterone, is related to cardiopulmonary exercise capacity in men with CHF. However, these findings have not been widely confirmed in other studies. Therefore, we aimed to establish the clinical determinants and hormonal anabolic deficiencies that could explain exercise intolerance in a cohort of patients with CHF.
Methods
The study group consisted of 104 consecutive patients with established diagnoses of CHF who were followed up in an outpatient specialized clinic. The selection criteria were a left ventricular ejection fraction (LVEF) <40%, clinically stable condition (defined as the absence of hospitalization or signs of acute cardiac decompensation in the previous 4 weeks), and optimized medical therapy for CHF, which were all unchanged for ≥4 weeks (100% angiotensin-converting enzyme inhibitors or angiotensin receptor blockers, 100% β blockers, 84% loop diuretics, 58% digoxin, 55% spironolactone). For each patient, all procedures of the study were performed in the same morning. Blood samples were taken after a fasting period of ≥12 hours and a rest period of 20 minutes. We prospectively recorded all clinical variables, and transthoracic echocardiography (Sonos 5500; Philips Medical Systems, Andover, Massachusetts) was carried out. The LVEF was calculated using the modified Simpson’s rule using second-harmonic imaging. Finally, symptom-limited cardiopulmonary exercise testing was performed. The study protocol was approved by the local ethics committee, and informed consent was obtained from each patient.
All patients underwent symptom-limited cardiopulmonary exercise testing using the modified Bruce protocol on a Marquette treadmill (GE Medical Systems, Milwaukee, Wisconsin). Pulmonary gas exchange and ventilatory variables were obtained from calibrated signals derived from rapidly responding gas analyzers and a pneumotachograph (CPX System; Medical Graphics Corporation, St. Paul, Minnesota) with the assessment of minute ventilation (VE), oxygen consumption (V o 2 ), and carbon dioxide production (V co 2 ) every 10 seconds using a mass spectrometer (Amis 2000, MedGraphics Cardio2 System; Innovision A/S, Odense, Denmark). Patients were encouraged to exercise to exhaustion, and all patients reached the anaerobic threshold and a respiratory ratio (V co 2 /V o 2 ) >1.05. Continuous 12-lead electrocardiographic monitoring was used. Blood pressure was recorded every minute using a sphygmomanometer cuff. All participants stopped exercise because of breathlessness and/or fatigue. None experienced chest pain or developed ST-segment deviation. Peak V o 2 was established as the highest value in the terminal phase of exercise. Peak V o 2 was expressed in milliliters per kilogram per minute and also as a percentage of the normal predicted peak V o 2 . Predicted peak V o 2 was calculated on the basis of gender, age, and weight using the formulas proposed by Oudiz et al. The slope of the relation between VE and V co 2 (VE/V co 2 ) was calculated as the ventilatory response to exercise. Platelet-poor plasma and serum fraction were obtained by centrifugation at 2,200g for 20 minutes. Aliquots were stored at −80°C for bath analysis. Total testosterone (TT) was measured using electrochemiluminescence immunoassay kits in a Modular Analytics E 170 module (Roche Diagnostics GmbH, Mannheim, Germany) (range 0.02 to 15.0 ng/ml). Estimated free testosterone (eFT) was calculated using the equation of Vermeulen et al, taking into account TT, albumin, and sex hormone–binding globulin. Sex hormone–binding globulin was measured using immunoradiometric assay kits (Orion Diagnostica, Espoo, Finland) in an LKB luminometer (Wallac, Turku, Finland) (range 6.25 to 200 nmol/L). Dehydroepiandrosterone sulfate (DHEA-S) was determined by immunometric assay (Immunotech, Marseille, France) in an LKB luminometer (range 0 to 1,000 μg/100 ml). Insulin-like growth factor–1 (IGF-I) was determined using immunoradiometric assay kits (Immunotech) in an LKB luminometer (range 0 to 1,200 ng/ml). N-terminal pro–B-type natriuretic peptide (NT–pro-BNP) was measured using electrochemiluminescence immunoassay using a Modular Analytics E 170 module (range 5 to 35,000 pg/ml). C-reactive protein was measured using immunoturbidimetric assay in a Modular P 800 analyzer (Roche Diagnostics GmbH) (range 0.1 to 25.8 mg/dl). For each hormone, the presence of hormone deficiency was defined using the normal limit for the gender- and age-adjusted value provided by the manufacturer. The estimated glomerular filtration rate was calculated using the simplified Modification of Diet in Renal Disease (MDRD) equation (186.3 × plasma creatinine − 1.154 × age − 0.203; the correction factor for women was 0.742).
Continuous variables were tested for a normal distribution using the Kolmogorov-Smirnov test. Normally distributed data are presented as mean ± SD and non-normally distributed variables as medians with interquartile ranges; the latter were log transformed, which made it possible to normalize their distribution for statistical analysis. Categorical variables are expressed as percentages. Single-predictor and multivariate regression analysis were applied to establish variables determining hormone levels, peak V o 2 , predicted peak V o 2 , and VE/V co 2 slope. In the single-predictor analysis, we included clinical parameters (age, body mass index, LVEF, and CHF duration), major co-morbidities (renal function assessed by the estimated glomerular filtration rate, anemia assessed by hemoglobin level, and the presence of diabetes mellitus), and serum levels of uric acid, C-reactive protein, and NT–pro-BNP. We also included sex hormone–binding globulin and anabolic hormones (TT, eFT, DHEA-S, and IGF-1) to determine cardiopulmonary exercise test parameters. During the construction of multivariate models, we included all the variables that had been shown to be significant determinants in single-predictor models. Forward and backward stepwise multivariate analysis was applied, with p = 0.10 for the inclusion and exclusion of variables in the model. A p value <0.05 was considered statistically significant. The statistical analysis was performed using SPSS version 15.0 for Windows (SPSS, Inc., Chicago, Illinois).
Results
A total of 104 men with CHF were included in this study. Table 1 lists the clinical characteristics and the nonhormonal laboratory parameters. Anabolic hormonal levels were as follows: TT 4.35 ± 2.54 ng/ml, eFT 75.2 pg/ml (interquartile range 52.0 to 101.3), DHEA-S 1,045 ng/ml (interquartile range 560 to 1,707), and IGF-1 127.5 ± 52 ng/ml. According to the age-adjusted reference values, hormonal deficiencies were present in 29% for TT, 39% for eFT, 34% for IGF-1, and 61% for DHEA-S. At least 1 anabolic deficiency was present in 74% of patients: 39% had 1, 15% had 2, and 19% had 3 anabolic deficiencies.
Variable | Value |
---|---|
Age (years) | 53.1 ± 10.6 |
Body mass index (kg/m 2 ) | 27.6 ± 3.9 |
Nonischemic cause | 86 (83%) |
Ischemic cause | 18 (17%) |
CHF duration (months) | 53 (18–97) |
New York Heart Association class | 2.0 ± 0.6 |
I | 14 |
II | 72 |
III | 14 |
IV | 0 |
Sinus rhythm | 79 (77%) |
Bundle branch block | 51 (49%) |
Echocardiography | |
LVEF (%) | 29.7 ± 11.9 |
Left atria (mm) | 47.8 ± 9.5 |
Left ventricular end-diastolic volume (ml) | 206 (65–284) |
Left ventricular end-diastolic diameter (mm) | 65.5 (58–71) |
Biochemistry | |
Estimated glomerular filtration rate (ml/min/1.73 m 2 ) | 72.6 ± 24.8 |
Hemoglobin (g/dl) | 14.8 ± 3.3 |
Sodium (mg/dl) | 139 ± 3.4 |
Potassium (mg/dl) | 4.7 ± 0.5 |
NT–pro-BNP (pg/ml) | 668 (183–1,745) |
Uric acid (mg/dl) | 7.1 ± 2.0 |
Total cholesterol (mg/dl) | 184 ± 40 |
C-reactive protein (mg/dl) | 0.30 (0.10–0.50) |
Cardiopulmonary exercise test | |
Peak V o 2 (ml/min/kg) | 18.6 ± 5.1 |
Predicted peak V o 2 (%) | 55.3 ± 15.0 |
VE/V co 2 | 33 (30–37) |
DHEA-S levels were correlated inversely with age (r = −0.37, p <0.001), CHF duration (r = −0.26, p = 0.011), NT–pro-BNP (r = −0.43, p <0.001; Figure 1 ) and uric acid (r = −0.23, p = 0.021). After adjusting in a multiple linear regression analysis, only NT–pro-BNP remained an independent predictor of DHEA-S levels (β = 0.30, p = 0.01). TT showed negative correlations with body mass index (r = −0.22, p = 0.021) and LVEF (r = −0.2, p = 0.045) and borderline correlations with hemoglobin (r = 0.18, p = 0.07) and NT−pro-BNP (r = 0.17, p = 0.08). However, only hemoglobin (β = 0.22, p = 0.029) remained an independent predictor after multiple linear regression analysis. IGF-1 and eFT did not show any significant correlations with variables at baseline (p >0.05 for all analyses).
In the single-predictor models ( Table 2 ), peak V o 2 was positively correlated with age, body mass index, NT–pro-BNP, estimated glomerular filtration rate, and uric acid. Predicted V o 2 was correlated with LVEF, duration of CHF, NT–pro-BNP, hemoglobin, and estimated glomerular filtration rate, whereas VE/V co 2 slope was correlated with age, LVEF, CHF duration, NT–pro-BNP, uric acid, and the presence of diabetes mellitus. Among hormonal parameters, DHEA-S showed a significant correlation with peak V o 2 (r = 0.29, p = 0.007), predicted peak V o 2 (r = 0.28, p = 0.006), and VE/V co 2 slope (r = −0.39, p <0.001), but TT, eFT, and IGF-1 showed no significant correlations with any of these variables. After adjusting in the multivariate model ( Table 3 ), DHEA-S remained an independent predictor of each exercise parameter. Significant impairment was found across tertiles of DHEA-S in terms of peak V o 2 ( Figure 2 ), predicted peak V o 2 , and VE/V co 2 slope (p <0.003 for all parameters), but we did not find any significant differences across tertiles of TT, eFT, and IGF-1.
Determinant | Peak V o 2 | Peak V o 2 Predicted | VE/V co 2 Slope | |||
---|---|---|---|---|---|---|
r | p Value | r | p Value | r | p Value | |
Age | −0.50 | <0.001 | — | — | 0.31 | 0.007 |
Body mass index | −0.27 | 0.011 | 0.07 | 0.535 | −0.15 | 0.186 |
LVEF | 0.01 | 0.365 | 0.26 | 0.015 | −0.25 | 0.034 |
CHF duration | −0.15 | 0.193 | −0.43 | <0.001 | 0.39 | 0.01 |
Log 10 (NT–pro-BNP) | −0.27 | 0.010 | 0.42 | <0.001 | 0.43 | <0.001 |
Log 10 (hemoglobin) | 0.18 | 0.100 | 0.29 | 0.012 | −0.14 | 0.223 |
Estimated glomerular filtration rate | 0.27 | 0.014 | 0.24 | 0.029 | −0.16 | 0.179 |
Uric acid | −0.26 | 0.017 | −0.17 | 0.139 | 0.35 | 0.003 |
Diabetes mellitus | t = −0.17 | 0.125 | t = −0.10 | 0.373 | t = 0.29 | 0.010 |
TT | 0.06 | 0.572 | 0.05 | 0.652 | 0.11 | 0.350 |
Log 10 (eFT) | 0.07 | 0.514 | 0.09 | 0.425 | 0.10 | 0.938 |
Log 10 (DHEA-S) | 0.29 | 0.007 | 0.29 | 0.008 | −0.39 | <0.001 |
IGF-1 | −0.03 | 0.852 | −0.15 | 0.300 | −0.04 | 0.817 |
Sex hormone–binding globulin | 0.03 | 0.793 | −0.03 | 0.763 | −0.05 | 0.639 |
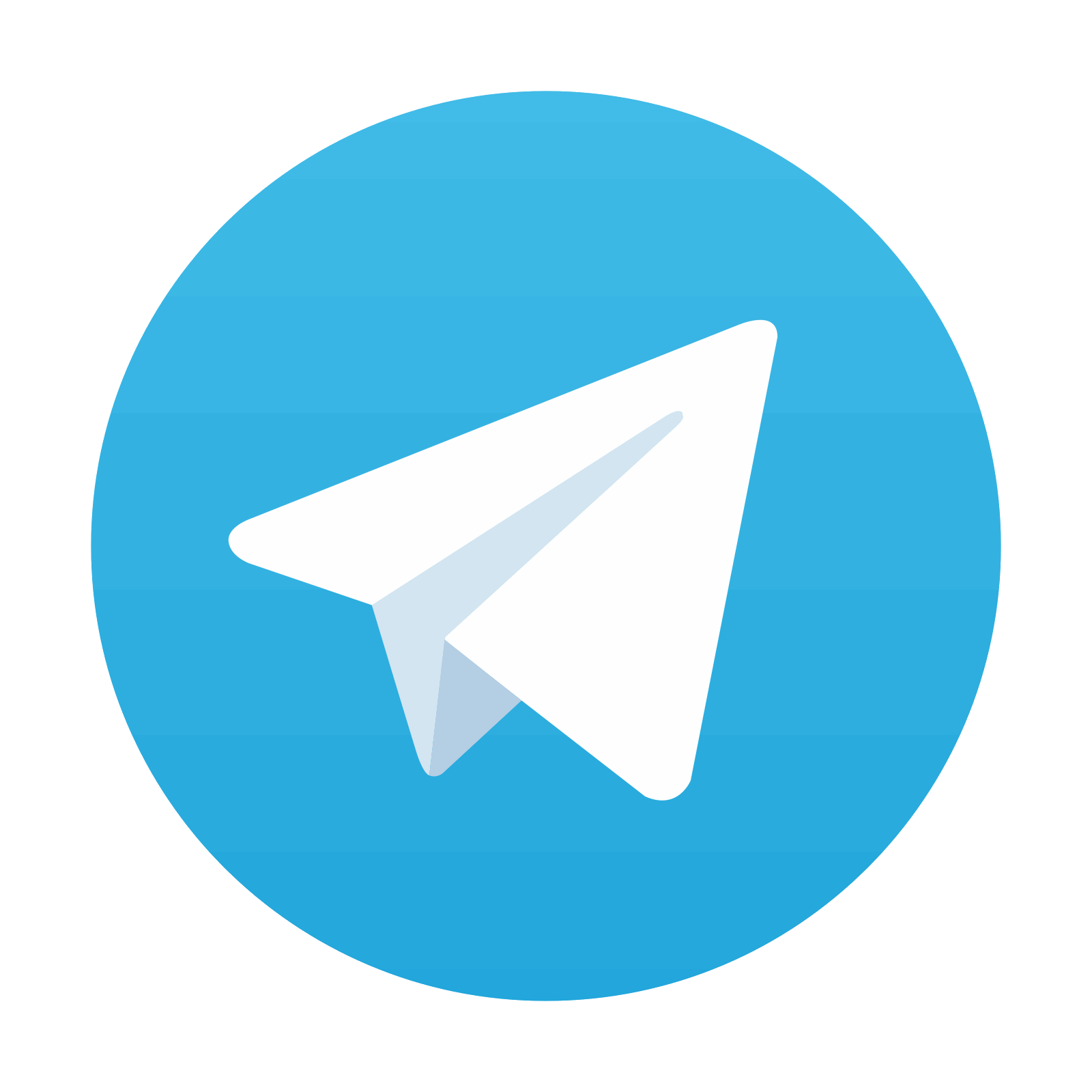
Stay updated, free articles. Join our Telegram channel

Full access? Get Clinical Tree
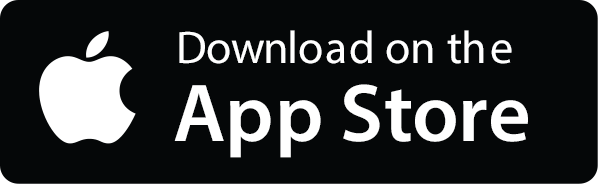
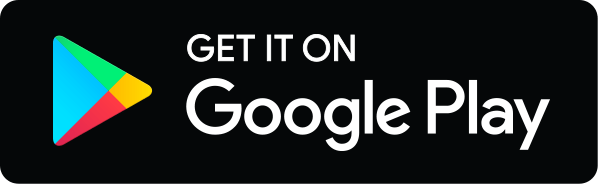
