Fig. 15.1
Pathophysiology of hypertension and heart failure. Schematic of the progression from hypertension to LVH to the clinical syndrome of heart failure. (a) The exact mechanisms that determine LV geometry are unclear; however, there are a variety of factors that may play a role in the progression to eccentric or concentric hypertrophy. (b) While the transition from concentric to eccentric hypertrophy can occur, most commonly the transition requires an interceding ischemic event. C: The subsequent maladaptive neurohormonal responses result in progression from LVH to overt heart failure symptoms. SBP systolic blood pressure, PVR peripheral vascular resistance, LV left ventricle, RAAS renin angiotensin aldosterone system, SNS sympathetic nervous system, LVH left ventricular hypertrophy
Arterial hypertension is the most common risk factor present prior to the development of heart failure. In the Framingham Heart Study, the vast majority (90 %) of patients diagnosed with heart failure had antecedent hypertension [6]. The same risk was seen in patients with asymptomatic LV dysfunction with 65 % of patients having a prior history of hypertension [7]. In the original Framingham cohort, the presence of hypertension doubled the risk of developing clinical heart failure.
The silver-lining to these bleak statistics comes from data demonstrating that the treatment and control of hypertension reduces the risk of developing heart failure by 50 % [8, 9], reduce the incidence of LVH by EKG, and slows the progression from Stage A / B to Stage C heart failure [10, 11]. In the SHEP Trial [10], the treatment of HTN resulted in a 50 % reduction in the incidence of HF with even greater benefit (80 % reduction) seen in patients with a history of myocardial infarction (MI). While this benefit has been seen most dramatically in the elderly, modification of risk is of the utmost importance to reduce the incidence of heart failure in the entire population. The benefit of BP control is seen irrespective of the anti-hypertensive strategy used, with the exception of alpha-blockers. The treatment of HTN with diuretics, angiotensin-converting enzyme [ACE] inhibitors, angiotensin II receptor blockers [ARBs], diuretics, Calcium Chanel Blockers [CCBs], β-blockers all reduce the incidence of heart failure. However, current data demonstrates that ACEIs and ARBs are most effective at improving the detrimental LV remodeling and improving outcomes in patients with reduced LV function [12], followed by beta-blockers to a lesser extent.
The Basics of ABPM in Heart Failure
The application of ABPM to congestive heart failure has been limited to date, with much of the data having been gathered prior to the current era of guideline-directed medical therapy (GDMT). The data that has been published has focused mainly on describing the 24-h, nocturnal blood pressure and blood pressure variability in heart failure; there is almost no data regarding the management and outcomes of patients treated by ABPM. There remains little basic data comparing the variation in BP readings between office, ambulatory and home blood pressure monitoring in heart failure, or the prevalence of white coat hypertension and masked hypertension. These basic analyses are required to aid in the application of ABPM to heart failure. Guidelines for ABPM have established lower definitions for hypertension and treatment goals compared to office and home blood pressure measurements. To date, there has been no similar studies to define appropriate blood pressure levels in heart failure by ABPM. Some limited data has described the prognostic benefits of ABPM in evaluating heart failure patients. The studies are small and limited in scope. The potential advantages of ABPM compared to traditional office blood pressure monitoring in congestive heart failure include: identification of White Coat Hypertension, Masked hypertension, nocturnal hypertension, describing dipping profiles, evaluation of possible orthostatic hypotension, monitoring of heart failure medication effectiveness, and titration of GDMT. Some small studies have suggested that ABPM, specifically nocturnal blood pressures, may be superior to office blood pressure measurements in predicting hospitalization for heart failure; large scale studies are needed to confirm these findings [13].
As with many chronic diseases, early identification and prevention are keys to reducing the incidence and decreasing the negative impact of the disease on society. The use of ABPM to assess patients at risk for heart failure may identify individuals that could potentially benefit from intensified therapy and life-style modifications to reduce their risk. ABPM correlates more strongly with LVH than office BP measurements [14] and has been inversely related to diastolic dysfunction [15]. LV afterload pressures, which can be detected during ambulatory blood pressure readings, may play a significant role in the development of LV dysfunction. 24-h ambulatory blood pressures have been strongly correlated to early left ventricular filling parameters [16], which can identify patients that may benefit from tighter blood pressure control and afterload reduction. In fact, the reduction in average BP recorded by ABPM has been shown to correlate with regression of LVH [17], demonstrating that early intervention may decrease the risk for developing heart failure. However, the limitation of much of this data is that long-term evaluation of heart failure outcomes has not been studied. While data has shown that ABPM may help identify patients with greater risk and that treatment has decreased surrogate markers (structural abnormalities) that may be precursors to heart failure, there are no studies that have shown a direct correlation between treatment and outcomes based on ABPM. While the use of GDMT has demonstrated significant benefit in the progression of heart failure and development of heart failure in patients at risk, the application of ABPM may provide further insight into therapeutic strategies and targets in the future.
One of the earliest signs of progression of heart failure is hypotension and the inability to tolerate GDMT. The need to withdraw or reduce heart failure medications portends poor prognosis in patients. In several small clinical trials, patients with higher blood pressure [18] had better outcomes and patients with NYHA Class IV heart failure with higher mean 24-h, awake and sleeping blood pressures had better prognosis compared to those with lower blood pressures [19]. Furthermore many heart failure symptoms, fatigue, dizziness, lightheadedness, and decreased exercise tolerance, can also be symptoms of hypotension. The presence of persistent or prolonged periods of hypotension, which may result from heart failure therapies, may predispose patients to increased risk of target organ dysfunction. The combination of low cardiac output and significant hypotension may increase the risk of renal dysfunction, pre-syncope, syncope, and altered mental status. The application of ABPM could possibly aid in determining optimal dosing and timing of GDMT to reduce potential side-effects and maintain optimal therapies. Currently, there remains a paucity of large randomized data, demonstrating the potential benefits of utilizing ABPM to optimize treatment in heart failure.
Circadian Variation in Heart Failure
The importance of circadian variations in blood pressure was first described almost four decades ago and the literature supporting prognostic impact of ABPM in cardiovascular disease continues to grow. In healthy subjects, BP is highest in the early morning hours and declines to its lowest levels at night. Normal circadian rhythms are dictated by various mechanisms including the sympathetic nervous system, postural position, baroreflexes, physical activity, tobacco use, sodium intake, alcohol use, and neurohormones. Over the ensuing decades, the superiority of circadian blood pressures, specifically nocturnal BPs, has been repeatedly demonstrated for cardiovascular outcomes in many disease states including hypertension, diabetes, stroke, and kidney disease.
The two most influential circadian patterns for cardiovascular disease are early morning surge BP and the nocturnal dipping profile. As with the majority of ABPM data, there is a relative paucity of information regarding the risk and benefits of ambulatory circadian variation in heart failure, and much of the data that exists were obtained prior to the current era of GDMT. Current guidelines do not discuss the potential role of circadian variation in heart failure management [20].
Early studies that assessed the circadian variation in heart failure have demonstrated a correlation between circadian BP and left ventricular dysfunction; however, the risk or outcomes attributed to ambulatory readings were not assessed [21]. The c urrent literature suggests that heart failure patients have depressed circadian variation compared to normal controls (Fig. 15.2). In heart failure up-regulated neurohormones, increased sympathetic activity, salt and water retention, and impaired baroreceptors may impact the normal circadian rhythm. Heart failure pharmacologic therapies that modulate the neurohormonal milieu, such as beta-blockers and ACEI, may also play a role in the altered circadian rhythms . The findings regarding average 24-h blood pressure in heart failure patients have been conflicted, which impacts the assessment of ambulatory circadian variation. Several small studies have demonstrated different average daytime blood pressures ranging from 108/72 mmHg [19] to 131/77 mmHg [22]. The data obtained by Borne et al. [21] demonstrated even lower ambulatory daytime and nocturnal blood pressures in NYHA Class III-IV patients. These conflicting data highlight the need for large studies to assess the circadian blood pressure patterns in the heart failure population, especially in the current era of GDMT. The effects of neurohormonal activity, LV function, gender, etiology of cardiomyopathy, functional class, and medication need to be better understood to appropriately apply ABPM data in heart failure. While much is still poorly understood, some data exists as a starting point, demonstrating that neurohormonal activity was indirectly related to circadian variations while ventricular function was directly associated with greater variability [22]. Furthermore, the severity of HF has been linked to decreased circadian variation, as heart failure progresses the degree of circadian variation in BP also declines.
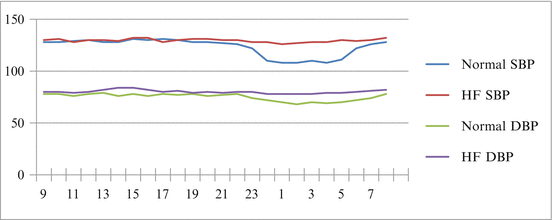
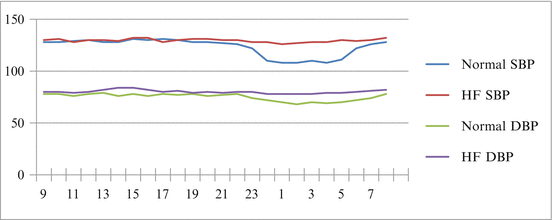
Fig. 15.2
24-h Blood pressure curve in normal controls compared to heart Failure. An illustration of the blunted circadian variation in both systolic and diastolic blood pressure in patients with Heart Failure compared to normotensive controls. SBP systolic blood pressure, DBP diastolic blood pressure, HF heart failure
Dipping Profile in Heart Failure
In healthy controls, the normal circadian pattern is one of nocturnal dipping or a decline in BP from ambulatory daytime BPs. Typically, this decline is approximately 20 % compared with awake readings; however, the general consensus is that a decline or a “dip” of <10 % from day to night BP readings is considered abnormal and is associated with poor CV outcomes [20]. Current literature classifies patients based on their nocturnal dipping profile: (1) Dippers, a normal decline in BP from day to night, (2) Non-Dippers, a 0–10 % decline in nocturnal BP, (3) Extreme dippers, those with >20 % decline in BP, and (4) Risers, an increase in nighttime BP from daytime readings. Dippers and extreme dippers have the lowest cardiovascular risk, while non-dippers and risers have the highest, incrementally.
While these definitions have been applied to heart failure patients, there is no consensus on what constitutes a normal dipping profile in heart failure. In a large cohort of NYHA Class II–III heart failure patients, the majority had an abnormal dipping profile using the standard definitions. In the same cohort, the presence of an abnormal dipping profile was an independent predictor HF outcomes. Non-dippers and risers had a 1.6 and 2.7 times increased risk of death or hospitalization compared to dippers, respectively [13]. Interestingly, in this cohort the majority of patients were on GDMT, which included metoprolol as a beta-blocker. If up-regulation of the sympathetic nervous system indeed plays a role in the altered circadian rhythm in heart failure, the use of alpha-adrenergic blockade along with beta-blockade (such as carvedilol) may help to normalize the dipping profile in these patients. There is, however, no data demonstrating benefit in restoration of a normal dipping pattern in HF. In fact, there is some data that suggests a normal dipping profile may be detrimental in heart failure. Canesin MF et al. [19] studied the effect of dipping profile on survival in 38 NYHA Class IV HF patients. Patients who had ≤6 mmHg decline in mean nighttime BP or nocturnal systolic BP had better prognosis at 6 months. More importantly, patients with a sleep SBP <105 mmHg were almost eight times more likely to die. While these findings cannot be extrapolated to patients with less severe heart failure (NYHA Class I–III), they do raise considerable questions regarding the normal dipping profile in HF patients. Large-scale assessment of dipping profiles in HF patients with varying severity is required. Establishing values to define dippers and non-dippers in HF is essential; it is possible that a dip of <10 % is beneficial in HF. Establishing these definitions are important in ultimately determining if pharmacotherapy can be used to normalize the dipping profile and improve outcome. Chronotherapy may play a significant role in the future of heart failure management. The administration of ACEI at bedtime has been shown to normalize dipping profiles in refractory hypertensives, and while it has become widely accepted to dose ACEI twice daily in heart failure therapy, adjusting the dosing between morning and evening doses may provide further benefit in the dipping profile in this population.
Morning Surge BP and Heart Failure
After a normal decline in blood pressure during the quiescence of sleep, a physiologic increase in BP upon wakening should occur. The physiologic increase in neurohormones, cortisol, and heart rate are likely triggers for the increase in BP. An increase in cardiovascular events, including stroke and MI, has been associated with this rise in morning blood pressure. Morning surge BP is an exaggerated rise in morning blood pressures to levels significantly higher than mean daytime values and may add incremental risk beyond the normal morning rise. There is limited recommendation on the application of morning surge BP in clinical practice.
The prevalence and degree of morning surge BP is undefined in heart failure. The use of GDMT that inhibit the SNS and regulate neurohormonal activity may blunt the morning rise; however, the altered baroreceptor reflex and chronic adrenergic up-regulation may result in significantly higher morning BP elevations.
In a sub-study of the PRAISE trial [23], the authors evaluated the timing of sudden cardiac death (SCD) in NYHA Class III–IV patients. The analysis of the data revealed a non-uniform distribution of SCD with a peak occurring in the evening not in the early morning. The evening peak was seen only in those with an ischemic etiology. The data suggests that the chronically elevated neurohormones resulted in a more uniform distribution. The study did not assess for heart failure admissions or cardiovascular mortality. The presence of an early morning surge may suggest suboptimal neurohormonal blockade and the spike in catecholamines may result in more rapid progression of heart failure.
Studies evaluating the impact of early morning BP surge on heart failure outcomes and long-term progression of the disease process may provide important clinical information and offer a potential target for future therapies. Chronotherapy aimed at reducing the morning surge may prove beneficial in the management of chronic heart failure.
White Coat Hypertension and White Coat Effect
White coat hypertension (WCH) is the presence of elevated BPs during contact with medical professionals, with otherwise normotensive BPs on ABPM in patients not currently on anti-hypertensive therapies. While it remains controversial, the general consensus is that white coat hypertension places patients at increased cardiovascular risk compared to the general population. Given that the majority of heart failure patients, including Stage A HF, are likely to be on some anti-hypertensive therapy, establishing guidelines for WCH in heart failure may be limited. However, in the rare patient with risk factors not actively on therapy, the presence of WHC may prompt closer monitoring of blood pressure and reemphasis on the importance of life-style modifications for preventing progression of the disease. Current data do not support treating WCH with pharmacologic therapies.
White coat effect (WCE) is the presence of higher BPs during clinic visits with lower, although still elevated, BPs on ambulatory readings in patients on therapy. Current consensus is that WCE >20 mmHg systolic and >10 mmHg diastolic are clinically relevant [20]. To the authors knowledge, there is no evidence demonstrating the presence of WCE in heart failure patients, nor demonstrating any increased risk from its presence. The lack of evidence highlights the gaps in knowledge regarding ABPM and HF. The importance of identifying patients with WCE would be to prevent unnecessary titration of medications, which may place patients at increased risk for adverse events. Patients identified to have WCE may require closer monitoring of BPs with more frequent home BP monitoring or repeat ABPM. In the treatment of Stage C heart failure, current guidelines recommend titrating GDMT to maximum tolerated doses, which should be performed regardless of the presence or absence of WCE. Therefore, defining WCE may be of limited benefit in these patients.
Masked Hypertension
Masked hypertension (MH) is traditionally defined as having normal office blood pressure (<140/90 mmHg) in the presence of elevated daytime ambulatory BPs (>135/85 mmHg) [20]. While current hypertension guidelines have recommended values for the diagnosis of MH in the general population, there are no data specifically in heart failure patients. Patients with heart failure, diabetes, LVH, obesity, and other comorbid conditions are more likely to have MH; the exact prevalence of MH in the heart failure population requires defining.
This definition is traditionally applied to those not currently receiving therapy, as HTN clearly cannot be defined as “masked” if indeed it is being treated. However, in the heart failure population using the term “masked” hypertension, or some derivation thereof, may play an important role. If patients with Stage A or B heart failure only have blood pressure readings in the office, the presence of elevated ambulatory BPs may result in undertreatment of an important risk factor for the progression of the disease. The use of ambulatory monitoring may identify those patients who would benefit from intensified pharmacologic and life-style therapies. In patients with Stage C HF, the guidelines clearly recommend forced titration of GDMT to maximum tolerable doses [2]; however, clinicians may not be comfortable pushing the doses of many of the medications if office BP readings are controlled. Elevated daytime ambulatory BPs in the presence of controlled office readings would reassure the clinician that continued dose titration will be tolerated and repeated ABPM could demonstrate appropriate response to the therapies. Without data demonstrating the presence of “masked” hypertension or the benefit of treatment on heart failure outcomes, recommendations cannot be made. Future investigations should establish the prevalence of MH, the incremental risk associated with MH, and determine if normalization of ABPM readings in the presence of lower office BPs would confer benefit.
Ambulatory Hypotension
ABPM can provide important information regarding the presence of daytime ambulatory hypotension. While short/intermittent episodes of hypotension or orthostatic changes may not be captured during ambulatory monitoring, the identification of patients with prolonged periods of hypotension is possible. Heart failure is common in the elderly who are at risk for hypotension due to baroreceptor and autonomic dysfunction. The symptoms of hypotension, lightheadedness or presyncope from decreased cerebral perfusion and decreased exercise tolerance, may also be symptoms of worsening heart failure. Identification of patients with ambulatory hypotension could aid in the titration of GDMT and prevent target organ dysfunction.
Postprandial hypotension is the decline in systolic blood pressure within 90 min of ingesting a meal. The current literature does not specify a clear clinical definition of Postprandial hypotension, yet in certain populations it is more common than orthostatic hypotension. It has been associated with syncope, falls and cardiovascular events. Postprandial hypotension is common in the elderly, with one study demonstrating it in nearly 70 % of a geriatric population [24]; its prevalence in the heart failure population in unclear. In healthy subjects, cardiac output can increase by as much as 20 % after a meal; in patients with impaired ventricular function and reduced cardiac reserve, cardiac output may not be able to increase sufficiently. Typically, in heart failure this manifests as early satiety and decreased appetite; however, it may also result in postprandial hypotension. The prevalence and effect of postprandial hypotension should be evaluated in the heart failure population. Recommendations such as smaller more frequent meals may reduce the incidence and decrease the risk of adverse events.
GDMT may precipitate prolonged periods of hypotension which may not be recognized in the clinic depending on timing of the medications. Many patients take all of their medications in the morning and the onset of action of multiple anti-hypertensive medications may result in periods of hypotension. If discovered during ambulatory monitoring, the clinician could adjust the timing of medications to avoid unnecessary periods of low blood pressure while maintaining appropriate GDMT. Volume depletion from diuretic therapies could also increase the risk of ambulatory hypotension, which could prompt adjustment of the timing or dose of diuretic therapies. Little data exist regarding ambulatory hypotension in the heart failure population and studies should focus on defining the prevalence and risk associated with daytime hypotension.
Hypertension and Ischemic Heart Disease
Ischemic Heart Disease: Morbidity and Mortality
Ischemic heart disease (IHD) remains one of the most common chronic fatal illnesses with death rates of nearly 530,000 per year in United States of America [25]. According to National Hospital Ambulatory Medical care Survey, about 11,883,000 people visited a physician’s office for ischemic heart disease in the US 2001. IHD is an all-too-common initial step on a pathway that leads to various detrimental cardiovascular outcomes that negatively impact both the longevity and quality of life. IHD, or coronary artery disease (CAD) , is a condition which results from atherosclerosis and resultant plaque build-up in the coronary arteries. This plaque narrows the arteries with time and reduces coronary blood flow which presents as chest pain, resulting from myocardial ischemia. Eventually, an area of plaque can rupture causing thrombosis formation which itself can block local coronary blood flow or can dislodge to distant coronary artery resulting in embolic obstruction, with resultant myocardial infarction.
Risk Factors of IHD
There are several modifiable and some non-modifiable risk factors that predispose to the development of ischemic heart disease. Important risk factors include, but are not limited to, age, serum cholesterol, hypertension, tobacco use, sedentary life-style, diabetes mellitus, stress, obesity, family history, left ventricular hypertrophy, and various genetic factors [26–29]. These predisposing factors are advantageous in identifying people who are at increased risk of ischemic heart disease and who should be targeted for specific life-style and pharmacologic interventions aimed at prevention and treatment to reduce the incidence of IHD. The non-modifiable risk factors are age, gender, and family history of IHD. Among many, one of the most well-documented and important modifiable risk factors is arterial hypertension [30]. There are several epidemiological studies that have documented the strong correlation between hypertension and ischemic heart disease [31–33].
Impact of Hypertension
Hypertension is one of the most common causes of premature death worldwide and the problem continues to grow; in 2025, an estimated 1.56 billion adults will be living with hypertension [34]. The trend towards increasing obesity, sedentary life-styles, and an aging population contribute to the increased incidence of arterial hypertension. The overall prevalence of hypertension is similar in women and men based on recent NHANES data, though the prevalence varies by age [35]. Over 65 million Americans age 20 or older have hypertension, which translates into one in every three adults [36]. The prevalence of hypertension also varies with ethnicity and is several fold higher in the African American population [37]. In recently published US population data, the prevalence of hypertension after age 60 years is 60 % in Caucasians and 70 % in African Americans. According to the Framingham study on risk stratification, hypertension was the most significant risk factor leading to 40 % of coronary events in men and 68 % in women [38]. The relationship between hypertension and risk of cardiovascular events is continuous, consistent, and independent of other risk factors [46]. The higher the blood pressure, the greater the chance of ischemic heart disease, heart failure, and stroke. High blood pressure was a primary or contributing cause of death for more than 360,000 Americans in 2013—which is nearly 1000 deaths each day [36]. Of greater concern is the overall poor awareness and control of hypertension; recent data from a large multinational study consisting of 142,042 participants demonstrated that 46.5 % of the participants with hypertension were aware of the diagnosis, but only 32.5 % of those were being treated [39]. In U.S. only, about half (52 %) of people with high blood pressure have their condition under control [40]. These findings suggest substantial room for improvement in hypertension diagnosis and treatment. Hypertension, often referred to as the “silent killer,” results in progressive and unrecognized vascular damage increasing the risk of cardiovascular morbidity and mortality. Consistent and regular monitoring of blood pressure in patients with risk factors for CV disease allows for early identification of patients who may benefit from life-style modification and pharmacologic therapy that may prevent the development of chronic morbidity and mortality.
Correlation of IHD with Systolic and Diastolic Blood Pressures
Population-based observational data has consistently demonstrated a strong correlation between increased systolic blood pressure (SBP) and diastolic blood pressure (DBP) with ischemic heart disease [41, 42]. Data from the Framingham Heart Study [43] supported by more recent data [44, 45] indicates that SBP increases continuously across all age groups, whereas DBP increases until age 60 years and then begins to decrease steadily. We know that coronary arteries are perfused predominantly during diastole and significant variations in DBP affect coronary circulation directly. During systole, the contracting LV myocardium compresses intra-myocardial vessels and obstructs its own blood flow. There is sufficient data demonstrating a J-curve relationship between IHD and mortality when DBP was either too high or too low [46]. This simply extrapolates to an increased mortality from myocardial infarctions in patient on either side of range of optimum DBP [47]. As the prevalence of hypertension increases with age, adequate control of both SBP and pulse pressure rather than DBP in the elderly has become the dominant public health imperative. A large study including 902 patients with moderate to severe HTN reported the nadir of the J-Curve in DBP to be 85–90 mmHg, while no such J-curve relationship has been found between SBP and IHD; the risk of IHD increases steeply with increasing SBP [47]. In the Chicago Heart Association Detection Project in Industry, men 18 to 39 years of age at baseline with a BP of 130–139/85–89 mmHg or with stage 1 hypertension (SBP 140–159/ 90 = 99 mmHg) accounted for nearly 60 % of all excess IHD, overall cardiovascular disease, or all-cause mortality [33]. Effective control of both systolic and diastolic blood pressure with the use of life-style modification and antihypertensive therapies has resulted in major reductions in cardiovascular morbidity and mortality over the past 50 years [48]. Randomized trials have shown that lowering BP results in rapid reductions in cardiovascular risks [49]. As an illustration, a 10-mmHg–lower usual SBP (or a 5-mmHg–lower usual DBP) would predict a 50–60 % lower risk of fatal stroke and an approximately 40–50 % lower risk of death due to ischemic heart disease or other vascular causes at middle age [50].
Pathophysiology of IHD: Role of Hypertension
Pathophysiology relating to the development of ischemic heart disease in hypertension involves increased myocardial oxygen demand and decreased coronary blood flow. When SBP is elevated, there is an increase in both LV output impedance and intra-myocardial wall tension, which increases LV pressure load and cardiac work—leading to LV hypertrophy (Fig. 15.3). Arterial hypertension exerts strain on the arterial wall leading to fragmentation, thinning, and fracture of elastin fibers, as well as increased collagen deposition in arteries, which results in decreased compliance of these vessels and overtime development of atherosclerosis [51, 52]. Atherosclerosis leads to diminished coronary blood flow or, at least, a diminished coronary flow reserve. In addition to these structural abnormalities, endothelial dysfunction, which develops over time as a consequence of both aging and hypertension, contributes functionally to increased rigidity of arterial wall [53]. Many of the mechanisms of the initiation and maintenance of hypertension are also those that mediate damage to target organs, including the coronary vessels and the myocardium. These mechanisms include increased sympathetic nervous system and renin-angiotensin-aldosterone system (RAAS) activity; deficiencies in release and/or activity of vasodilators, for example, nitric oxide, prostacyclin, and the natriuretic peptides; structural and functional abnormalities in conductance and resistance arteries, particularly endothelial dysfunction; and increased expression of growth factors and inflammatory cytokines in the arterial tree [54]. All of these factors either increase myocardial demand or decrease oxygen supply to myocardium, leading to chronic stable angina at one end of the spectrum and acute myocardial infarction at the other end depending on the time lapse for which myocardium is deprived of oxygen.
< div class='tao-gold-member'>
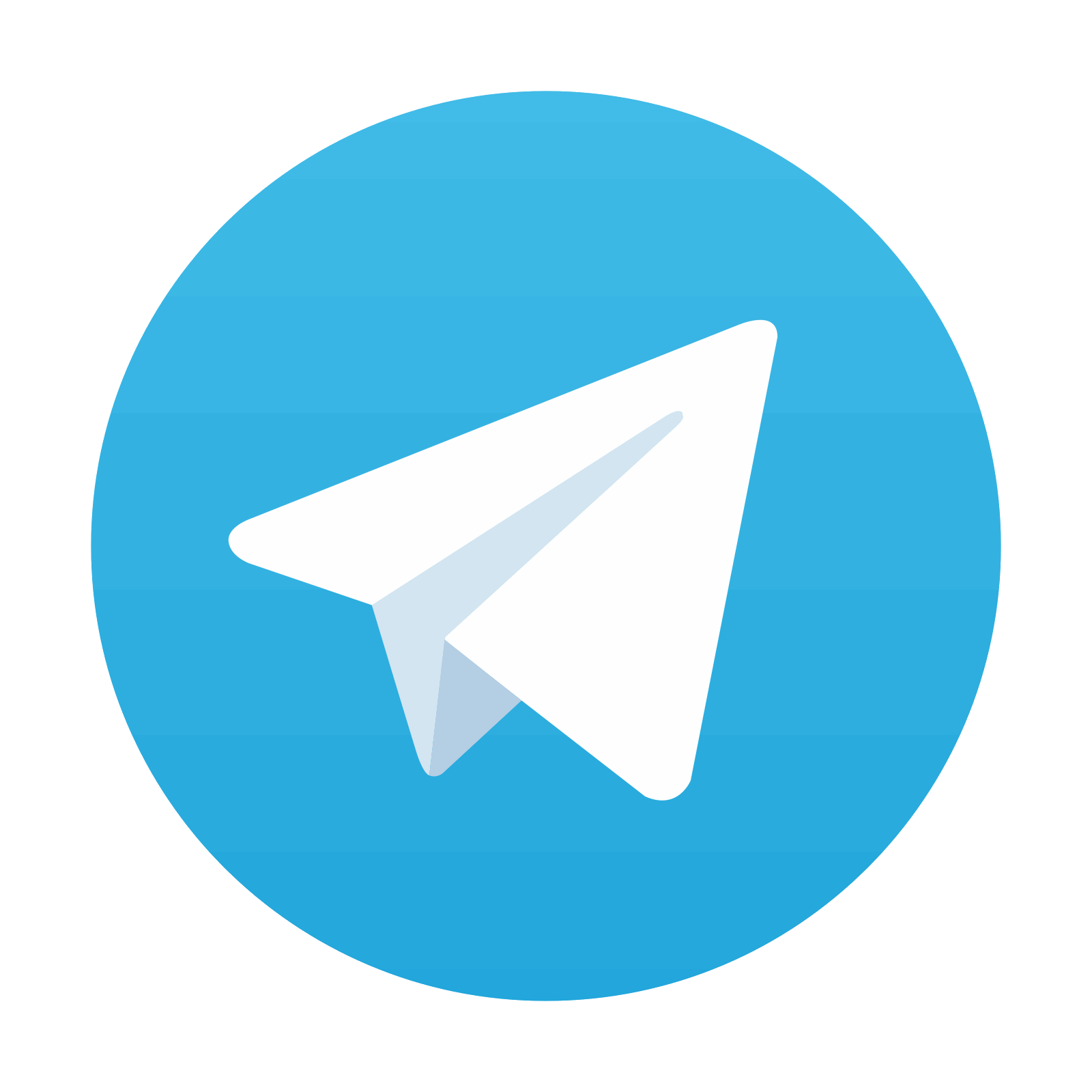
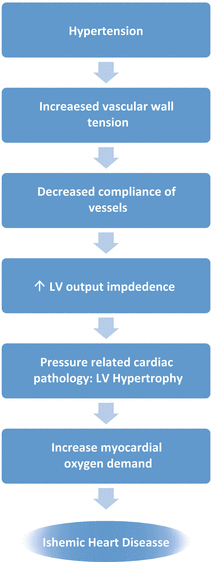
Only gold members can continue reading. Log In or Register a > to continue
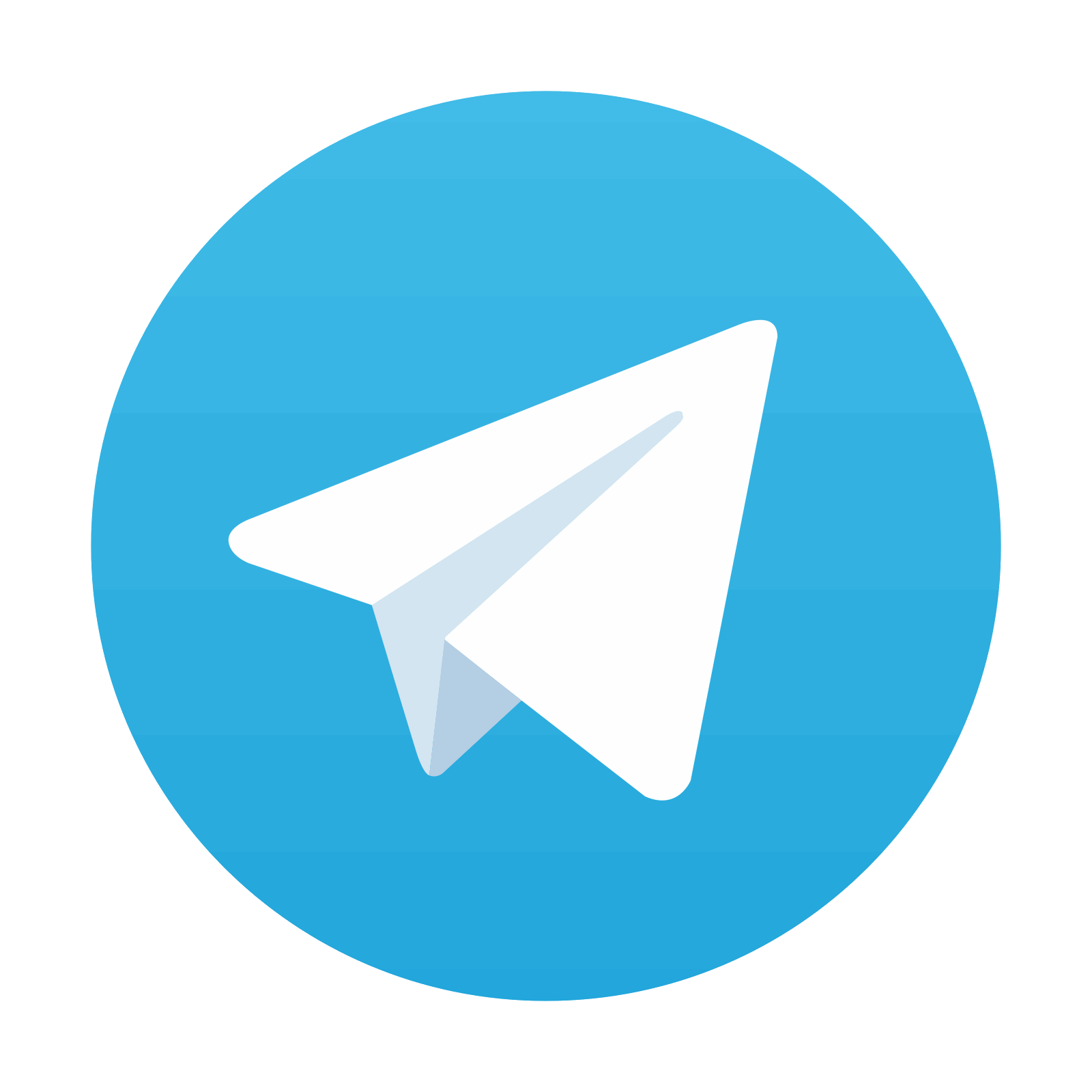
Stay updated, free articles. Join our Telegram channel

Full access? Get Clinical Tree
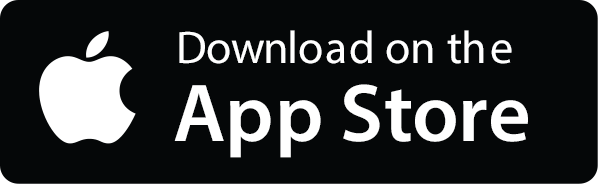
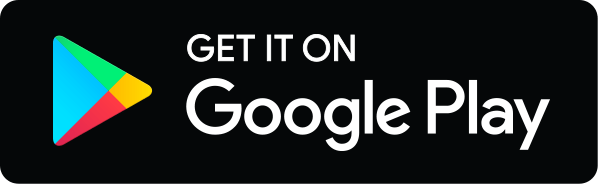