Thermal ablation involves the application of heat or cold energy to the lung under image guidance to eradicate tumors. It is indicated for treatment of early-stage non–small cell lung cancer in nonsurgical patients. Ablation technologies have advanced, such that nearly all small tumors can now be treated safely and effectively. Ablation does not cause a lasting decline in pulmonary function tests and may therefore be used to treat multiple synchronous and metachronous lung tumors, a chief advantage over other treatments. Large series with intermediate- and long-term data have been reported showing favorable overall survival, similar to radiation therapy.
Key points
- •
Thermal ablation is an established, validated treatment for early-stage non–small cell lung cancer (NSCLC) in nonsurgical patients.
- •
Overall survival after thermal ablation in large series and clinical trials is similar to radiation therapy.
- •
Thermal ablation does not cause a decline in pulmonary function tests and may therefore be used to treat multiple synchronous or metachronous lung tumors.
- •
Advances in ablation technology, including microwave and cryoablation, now allow safe and effective treatment of nearly all small tumors regardless of location within the lung.
ACOSOG | American college of surgeons oncology group |
CRYO | Cryoablation |
CT | Computed tomography |
DLCO | Diffusion capacity for carbon monoxide |
ECLIPSE | Evaluating cryoablation of metastatic lung tumors in patients – safety and efficacy |
FDG | Fluorodeoxyglucose |
FEV1 | Forced expiratory volume in one second |
FVC | Forced vital capacity |
Gy | Gray |
IRE | Irreversible electroporation |
MWA | Microwave ablation |
NSCLC | Non–small cell lung cancer |
OS | Overall survival |
PET-CT | Positron emission tomography – computed tomography |
PFTs | Pulmonary function tests |
RAPTURE | Radiofrequency ablation of pulmonary tumors response evaluation |
RFA | Radiofrequency ablation |
RTOG | Radiation therapy oncology group |
SBRT | Stereotactic body radiotherapy |
SEER | Surveillance epidemiology and end results |
TLC | Total lung capacity |
Introduction
Treatment of early-stage non–small cell lung cancer (NSCLC) with thermal ablation involves the percutaneous application of heat or cold energy to lung tissue by strategic insertion of an applicator under image guidance, to create a region of cell death that encompasses the targeted tumor and an ablative margin. The first reported application of thermal ablation to lung tumors in humans was a series of 3 patients, 2 with primary lung cancer and 1 with pulmonary metastasis, who underwent percutaneous image-guided ablation of their tumors using radiofrequency energy, a technique that had previously been applied to liver tumors. Since this initial proof of concept, more than 3000 studies of thermal ablation for lung tumors have been published in the medical and scientific literature. Refinements of ablation techniques have continually enhanced the efficacy and safety of these procedures and better defined specific patient populations for treatment. Over the past 2 decades, microwave and cryoablation have been introduced, allowing for the creation of larger ablation zones and enabling effective treatment of larger tumors with adequate ablative margins. Techniques have been developed that allow safe treatment of tumors in difficult locations within the lung, for example, the pulmonary hila or central lung, the lung apices, and peripheral or subpleural locations. Investigations in animal and human subjects have refined the understanding of the ablation zone, allowing for more precise treatment planning based on anatomic considerations and improving patient and morphologic follow-up. Furthermore, the publication of numerous large series of patients with long-term data, as well as several clinical trials, has provided robust validation of the efficacy of ablation with respect to both local progression, or incomplete ablation, and overall, cancer-specific and disease-free survival. This article briefly reviews the clinical and technical aspects of thermal ablation for lung cancer and provides an overview of the available clinical outcomes data.
Clinical aspects of thermal ablation
Patient Selection
Careful patient selection is the cornerstone of successful lung tumor ablation. In general, ablation provides only local control for small lung tumors, or early-stage lung cancers: those who would otherwise be surgically resectable but in patients who are medically inoperable, a patient cohort that has become defined as “high risk.” The reasons for unresectability may include poor pulmonary reserve, medical comorbidities, advanced age and frailty, or contraindications to anesthesia, and in some cases, patient refusal. None of the preceding factors preclude successful percutaneous tumor ablation. Studies have shown that ablation has either no effect on lung function or at most a small temporary decrement in forced expiratory volume in 1 second (FEV1) and diffusing capacity of the lung for carbon monoxide (DLCO), statistically indistinguishable from baseline after recovery. In 2006, a phase 2 study of radiofrequency ablation (RFA) and conventional radiotherapy for patients with unresectable stage NSCLC demonstrated no worsening of pulmonary function tests (PFTs) after treatment. In fact, a follow-up study in 2015 examining RFA alone for stage I NSCLC showed a sustained improvement in forced vital capacity (FVC) 2 years later, possibly because of a reduction in air trapping from cicatrization of the ablation zone after treatment, or thermoplasty. No changes in FEV1 or DLCO were observed in that study cohort. In contrast, even sublobar surgical resection has been associated with a measurable decline in pulmonary function. One study showed 7% to 9% decreases in total lung capacity (TLC), FEV1, FVC, and DLCO after wedge resection, with each additional resection associated with a linear functional decline. Likewise, stereotactic body radiation therapy (SBRT) is also associated with demonstrable decreases in TLC, FEV1, FVC, and DLCO. , For this reason, patients who may require treatment of multiple tumors, because of either multiple synchronous or metachronous primary cancers, or limited oligometastases, are often good candidates for percutaneous ablation because options for repeated surgical resection or radiation therapy may be limited. Tumors throughout both lungs may be ablated over multiple sessions, provided there is a reasonable expectation of improved oncologic outcomes from continued local treatment. This determination is often best made in the context of a multidisciplinary tumor board.
Tumor Size
Tumor size is arguably the most important factor in patient selection. The best oncologic outcomes in the setting of NSCLC have been reported for T1 tumors less than 3 cm. Although complete ablation of larger tumors can be achieved, efficacy is more operator dependent, and rates of incomplete ablation are greater. Obtaining adequate ablation margins around larger tumors, between 3 and 5 cm, usually requires multiple ablation applicators and applicator repositioning, adding to the complexity of the procedure. Nevertheless, experienced centers have reported good outcomes even for these larger tumors. For tumors larger than 5 cm, the likelihood of complete ablation decreases significantly, and the risk of complications increases. However, if local treatment is indicated and other options are not available, such tumors may be treated after appropriate counseling of the patient. Although the goal of the operator generally is to completely eradicate the tumor with 1 procedure, new or residual tumor detected on postprocedure imaging surveillance can be re-treated as necessary to achieve complete tumor control. Local tumor progression within the first year was found not to affect overall survival (OS) when re-treated. Because there is no or minimal effect of the procedure on lung function, there is likewise no maximum number of times a patient may undergo ablation.
Tumor Location
Location of the tumor within the lung plays an important role in treatment planning. Although there are few locations that preclude successful thermal ablation, local anatomic factors may influence choice of ablation modality and applicator or probe placement. For example, tumors in peripheral or subpleural locations may be better suited to cryoablation over heat-based ablation modalities, such as microwave energy, because of the greater tendency of the latter to result in indiscriminate tissue destruction in and around somatically innervated parietal pleura causing sometimes excessive procedural and recovery pain, and complications, such as bronchopleural fistula. Similarly, tumors in close proximity to central airways are often best treated with cryoablation, particularly because the ice created during the ablation can be visualized by computed tomographic (CT) scan. Proximity to large pulmonary blood vessels does not preclude thermal ablation provided the ablation probes are positioned carefully and direct puncture of the vessels is avoided. However, flowing blood within large vessels will dissipate thermal energy, a phenomenon known as heat or cold sink, and therefore, greater energy may be required to overcome this effect and achieve successful ablation. An in vivo animal study by Steinke and colleagues showed that RFA of lung adjacent to vessels greater than 3 mm in diameter consistently demonstrated evidence of heat-sink effect, potentially resulting in incomplete ablation and local tumor progression. Likewise, cryoablation is vulnerable to cold-sink effects, and probe placement adjacent to a vascular structure can result in inhomogeneous temperatures and increased risk for local failure. Location within the lung has also been found to influence the size of the ablation zone, with upper lobes and peripheral locations allowing larger ablation zones than lower lobes and central locations, possibly related to differences in tissue perfusion. Extrapulmonary structures at risk for damage must always be considered when planning the ablation procedure, particularly for tumors in peripheral locations within the lung lobe but central within the chest. These peripheral locations include the esophagus, pericardium, heart, and diaphragm, as well as nerves, such as the brachial plexus, phrenic and recurrent laryngeal nerves. Damage to intercostal nerves can occur, but clinical sequelae are usually not as severe.
Clinical Aspects of the Ablation Procedure
In contrast to lung resection, thermal ablation can generally be performed as an outpatient procedure. Severe medical comorbidities or significant complications may necessitate an inpatient admission, usually of brief duration. The procedure may be performed under general anesthesia but may also be performed safely and comfortably with intravenous moderate sedation and analgesia when there is no anticipated need for cardiopulmonary support or unusual pain control requirements. Advantages of moderate sedation include lack of positive airway pressure, more accurate breath-holding, faster procedure setup, shortened recovery, and lower cost. The authors’ practice is not to use preprocedure or postprocedure antibiotics because significant infectious complications are rare (see discussion of complications in later discussion), but operators may differ in this regard. Oral anti-inflammatory medications are generally sufficient for postprocedure pain control. If the ablation zone involves the pleura or chest wall, a short course of narcotics may be required in addition to anti-inflammatory agents.
Postprocedure Follow-Up
A follow-up visit and a chest radiograph should be conducted within a week after the procedure, to assess for complications, such as delayed pneumothorax and reactive pleural effusion, as well as overall patient recovery and well-being. The ablation zone should be assessed with chest CT or PET-CT at regular intervals after ablation. The authors’ protocol is to obtain a contrast-enhanced CT 1 month after the procedure to allow for the edema and acute inflammation imparted by ablation to subside, and more importantly, to establish a baseline ablation zone, followed by CT or PET-CT at 3-month intervals for the first year, and 6-month intervals thereafter, for at least the first 2 years after ablation. Local failure is suspected when there is increasing nodularity at the margin of the ablation zone, increasing enhancement within the ablation zone, increasing size of the ablation zone after 3 and especially after 6 months following ablation, or increasing fluorodeoxyglucose (FDG) uptake within the ablation zone after 2 months or in specific FDG patterns.
Ablation modalities
Radiofrequency Ablation
RFA has been used to treat pulmonary malignancies for almost 2 decades and is best studied among all ablative modalities. In RFA, an electrode connected to a radiofrequency generator is percutaneously placed into a tumor with image guidance. The generator creates an alternating current (460–480 kHz) between the electrode and grounding pads typically placed on patient’s thighs. The current causes local frictional tissue heating, resulting in irreversible cell death at more than 60°C. An ablation zone of 1 to 3 cm is created using a single or clustered tip electrode, and multiple electrodes may be placed at once for large lesions. A treatment session takes 4 to 12 minutes. A pitfall of RFA is its susceptibility to heat-sink effect, that is, dissipation of heat energy by flowing blood or moving air within the adjacent pulmonary vasculature or large bronchi, respectively. This heat sink can significantly reduce the amount of heat concentrated in the tumor and prevent tissue in close proximity to pulmonary vessels and airways from reaching cytotoxic temperatures. In addition, RFA can cause tissue charring, which impedes thermal conductance and limits growth of the ablation zone. Some RFA systems use an infusion pump to internally cool the electrode tip and mitigate charring.
Microwave Ablation
Microwave ablation (MWA) is another heat-based thermal ablative technique with several advantages over RFA ( Fig. 1 ). MWA uses a higher frequency of 915 or 2450 MHz, which causes rapid heating via excitation of water molecules. Compared with RFA, MWA has been shown to achieve higher intratumoral temperatures, larger ablation volumes, and faster ablation times (2–5 minutes, compared with 12–15 minutes for similar-sized lesions). This property allows for ablation of larger tumors and their satellite tumor deposits, theoretically reducing failure rate. No grounding pads are necessary in MWA, and patients experience less procedural pain, possibly from the lack of electrical nerve stimulation associated with RFA. Moreover, MWA is less susceptible to heat-sink effect and produces less charring and thermal impedance because of deeper tissue penetration inherent to this energy.

Cryoablation
Cryoablation is a thermal ablative modality that uses subzero temperatures and development of ice to destroy tumor cells ( Fig. 2 ). Its mechanism of action is based on the Joule-Thompson effect, where the change from liquid to gaseous state of argon within the ablation probe lowers the temperature in adjacent tissue down to less than −150°C, leading to intracellular and extracellular ice formation. A subsequent thaw cycle results in osmotic shifts across the cell membrane and cell lysis. Multiple freeze-thaw cycles are performed, for a typical ablation duration of 20 to 30 minutes. , The visibility of the low-density ice ball on CT imaging is 1 major advantage of cryoablation over other modalities. However, studies have shown that complete cell death does not occur until temperatures are lower than −20 to −40°C, which in practice means the visible ice ball must extend beyond the desired ablation zone by approximately 1 cm. , Cryoablation has several other advantages over RFA, including larger tumor ablation volumes, less procedural pain owing to analgesic effect to intercostal nerves, and no need for grounding pads. Cryoablation has the unique benefit of preserving the underlying collagenous architecture or the ablated tissue, , making it a safer modality for tumors in proximity to the pleura or to major airways. In addition, there is evidence that cryoablation may potentiate systemic immunotherapy agents by promoting local inflammatory cytokines. Disadvantages of cryoablation include increased risk of bleeding, longer procedural times, and the need for multiple cryoprobes to generate adequate coverage.

Irreversible Electroporation
Irreversible electroporation (IRE) is a new investigational nonthermal ablation modality that uses high-voltage electrical currents to create permanent nanopores in the cell membrane, which leads to apoptotic cell death. Theoretically, IRE overcomes limitations associated with thermal ablation, including heat-sink effect and risk of thermal injury to collateral tissues. IRE requires general anesthesia with complete neuromuscular blockade to prevent generalized muscle contractions and requires electrocardiogram-gating of high-voltage pulses to avoid serious cardiac arrhythmias. Studies on IRE safety or efficacy for treatment of pulmonary malignancies in humans are limited.
Choice of Modality
The size and anatomic characteristics of the target tumor are major considerations in choosing among ablative modalities. Although all ablative modalities suffer from higher local failure rates with large tumors (≥3 cm), MWA and cryoablation are generally preferred over RFA for larger tumors given their ability to achieve greater ablation zones. Anatomically, central tumors pose a challenge for ablation because of its proximity to critical vascular and airway structures. RFA is likely the least ideal modality given the risk of thermal injury to the mediastinum and of inadequate treatment from heat-sink effect. Cryoablation has the unique ability to preserve the collagenous architecture and thus the integrity of central vessels and airways, as well as the diaphragm; however, it may be subject to a cold-sink effect. In MWA, there is less heat-sink effect, but the risk of thermal injury still exists. Peripheral tumor ablations are also challenging because of associated pleuritic and chest wall pain. A randomized trial comparing MWA and RFA for treatment of lung cancer and pulmonary metastasis found no difference in OS, but greater decrease in size of the tumor with MWA, and less pain following MWA. Both MWA and cryoablation are associated with less procedural pain compared with RFA. Cryoablation, in particular, is likely beneficial because of its analgesic effect on intercostal nerves. Patient characteristics also should be taken into account when choosing ablative modalities. Many patients with severe emphysema or pulmonary fibrosis can have significant pulmonary hemorrhage after cryoablation. Table 1 provides a comparison of ablation modalities.
Parameters | RFA | Microwave | Cryoablation |
---|---|---|---|
≤3 cm tumor | +++ | +++ | +++ |
>3 cm tumor | + | +++ | ++ |
Subpleural location | + | + | +++ |
Chest wall | + | ++ | +++ |
Mediastinum | + | + | ++ |
Thermal sink | + | +++ | ++ |
Pacer/automated implantable cardioverter defibrillator | + | ++ | +++ |
Coagulopathy | +++ | +++ | + |
Maneuverability | + | + | ++ |
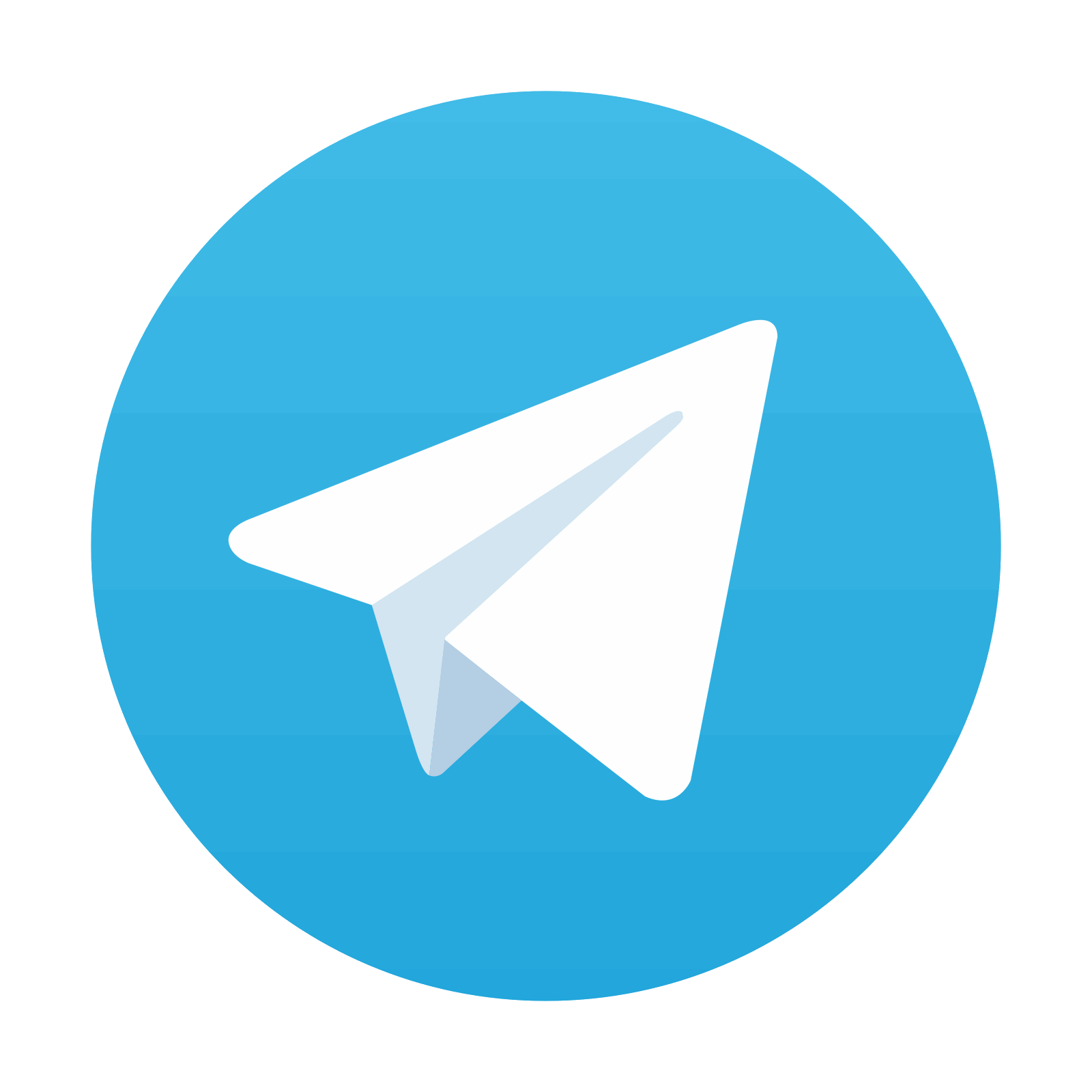
Stay updated, free articles. Join our Telegram channel

Full access? Get Clinical Tree
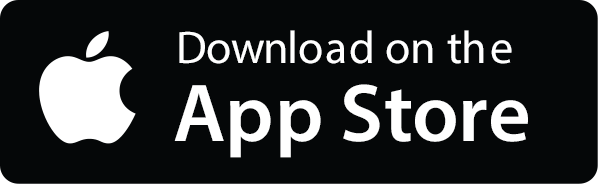
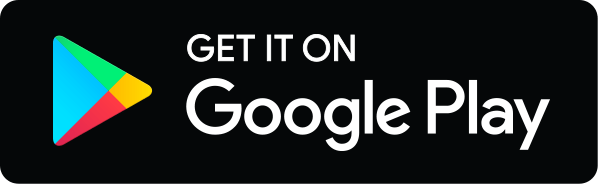
