Background
The left ventricle fills in early diastole because of a progressive intraventricular pressure difference (IVPD) that extends from the left atrium to the left ventricular (LV). The aim of this study was to test the hypothesis that in patients with symptomatic heart failure (HF) regardless of LV ejection fraction (EF), an increase in left atrial (LA) pressure maintains early diastolic filling because of a preserved IVPD from the left atrium to the mid left ventricle, while the IVPD from the mid left ventricle to the apex is diminished because of reduced LV suction.
Methods
One hundred fifty-one patients with HF (50 with HF with preserved EF [HFpEF; EF ≥ 50%] and 101 with HF with reduced EF [HFrEF; EF < 50%]) and 28 normal controls were prospectively enrolled. The IVPDs from the left atrium to the LV apex (total IVPD), the left atrium to the mid left ventricle (basilar IVPD), and the mid left ventricle to the apex (apical IVPD) were determined using color M-mode Doppler echocardiographic data to integrate the Euler equation. The propagation of early diastolic filling was also assessed by color M-mode Doppler.
Results
The mean LV EF was 0.63 ± 0.07 in patients with HFpEF, 0.32 ± 0.09 in those with HFrEF, and 0.64 ± 0.06 in controls. Peak early diastolic transmitral flow velocities (E) were similar among the groups, and basilar IVPDs were maintained in the HFpEF and HFrEF groups (HFpEF, 1.59 ± 0.62 mm Hg; HFrEF, 1.49 ± 0.75 mm Hg; controls, 1.80 ± 0.61 mm Hg; P = NS, analysis of variance). However, apical IVPDs were decreased in both HF groups (HFpEF, 1.18 ± 0.56 mm Hg [ P < .01 vs controls]; HFrEF, 0.87 ± 0.48 mm Hg [ P < .01 vs controls]; controls, 1.65 ± 0.62 mm Hg), resulting in decreased total IVPDs in patients with HF (HFpEF, 2.55 ± 0.80 mm Hg [ P < .01 vs controls]; HFrEF, 2.16 ± 0.80 mm Hg [ P < .01 vs controls]; controls, 3.17 ± 0.91 mm Hg). E/e′ ratios were increased in patients with HF, consistent with elevated LA pressure. In patients with HF, E was correlated with basilar IVPD but not with apical IVPD, whereas propagation of the filling was correlated with the apical IVPD but not with the basilar IVPD.
Conclusions
In patients with HFpEF and those with HFrEF, apical IVPDs were reduced while basilar IVPDs were maintained by elevated LA pressure, resulting in preserved E.
The left ventricle fills in early diastole because of a progressive pressure difference from the left atrium to the left ventricular (LV) apex. Normally, the intraventricular pressure difference (IVPD) is generated as rapid myocardial relaxation and recoil of elastic elements compressed during ejection causes LV pressure to fall below left atrial (LA) pressure. This results in a progressive IVPD that extends from the left atrium to the LV apex, driving brisk early diastolic filling that rapidly propagates to the apex. Normally, a reduction of early diastolic pressures at the LV apex during exercise allows enhanced LV filling without an elevation of LA pressure. This process is altered with diastolic dysfunction. In the presence of impaired relaxation, the pressure fall in the LV apex is diminished, resulting in a reduced IVPD and decreased early diastolic filling. In an animal model of progressively impaired LV function, an increase in LA pressure maintains or even increases the early diastolic IVPD and filling despite higher LV diastolic pressures. Although there is the normal or even increased peak early diastolic velocity (E) (pseudonormalized or restricted filling pattern) in most symptomatic patients with heart failure (HF), the total IVPD from the left atrium to the LV apex has been reported to be reduced in these patients. To explain this apparent paradox, we hypothesized that in patients with symptomatic HF regardless of LV ejection fraction (EF), increased LA pressure maintains the basilar IVPD from the left atrium to the mid left ventricle, while the apical IVPD from the mid left ventricle to the apex is diminished, resulting in reduced total IVPD. The basilar IVPD from the left atrium to the mid left ventricle accounts for the preserved or increased E wave in patients with symptomatic HF.
The IVPD can be analyzed noninvasively by integrating the Euler equation from echocardiographic color M-mode Doppler (CMMD) imaging, providing a noninvasive method to test our hypothesis. Accordingly, the aim of the present study was to determine the difference of the spatial distribution of the IVPD between patients with HF and normal controls.
Methods
Study Population
The study protocol was approved by the institutional review board of the University of Mississippi Medical Center (No. 2013-0254). Between November 2013 and June 2014, we prospectively evaluated 608 consecutive patients who underwent clinically indicated transthoracic echocardiography and had documented histories of symptomatic HF on the basis of the Framingham criteria. Exclusion criteria were non–sinus rhythm, left bundle branch block, fusion of early and late diastolic mitral inflow, significant left-sided valvular disease, prosthetic valve, and LV assist device. Of the 334 patients who were eligible for study inclusion, 183 with insufficient two-dimensional or CMMD echocardiographic images were also excluded before the image analysis. Accordingly, the remaining 151 patients with HF were included in the final analysis. The patients with HF were divided into those with HF with preserved EF (HFpEF) and those with HF with reduced EF (HFrEF), with a partition EF of 50%. Twenty-eight subjects with no histories of cardiovascular risk factors who underwent transthoracic echocardiography for the assessment of cardiac function (other organ disease, n = 13; screening, n = 5; atrial arrhythmia, n = 4; syncope, n = 4; palpitation, n = 2) and had no abnormal echocardiographic findings served as normal controls.
Echocardiography
Echocardiography was performed by using an iE33 ultrasound system with a multiple-frequency transducer (Philips Medical Systems, Andover, MA). Digital two-dimensional cine loops were obtained in the apical four-chamber, two-chamber, and long-axis views and short-axis views at the basal, midventricular, and apical levels at a mean frame rate of 72 ± 17 frames/sec (range, 40–140 frames/sec).
LV volumes and LA volume were measured according to the recommendations of the American Society of Echocardiography. LV mass was calculated according to Devereux formula and indexed to body surface area. Transmitral Doppler flow was recorded in the apical four-chamber view, and E, peak atrial velocity (A), and the E/A ratio were measured. Septal and lateral peak systolic annular velocities (s′) as well as the early diastolic peak of mitral annular velocities (e′) were measured from the apical four-chamber view by using pulsed-wave Doppler tissue imaging, and the average of the septal and lateral velocities was used for the subsequent analysis. The ratio of E to e′ was calculated. CMMD images were recorded with the cursor parallel to LV inflow in the apical four-chamber view. Diastolic function was graded as normal, impaired relaxation (grade I), pseudonormal (grade II), or restrictive filling (grade III) using e′, E/A, and E/e′ as previously described.
Myocardial function was also assessed by the speckle-tracking method by using QLAB Advanced Quantification Software version 9.0 (Philips Medical Systems) as previously reported. Briefly, the longitudinal parameters were obtained from three apical views, and the circumferential parameters were from the midventricular short-axis view. The global strain and strain rate curves were extracted with the use of entire LV wall in the image, and peak global strain, peak systolic global strain rate, and the early diastolic peak of global strain rate were measured. Longitudinal indices from three apical views were averaged and used for the final analysis. In addition to global strain, peak segmental longitudinal strain was measured from each segmental strain curve and averaged for the basal and apical levels. LV rotation was measured in the basal and apical short-axis views, and LV twist was calculated as apical rotation relative to basal rotation at each corresponding time point, which was normalized to the percentage of systolic duration.
Analysis of CMMD Images
CMMD images were analyzed using an in-house-developed automated analysis algorithm based on MATLAB (The MathWorks, Natick, MA). A more detailed description of this algorithm has been published. The isovelocity contours between 45% and 55% of peak E were detected, and an ensemble contour was fit to the series of isovelocity contours ( Figure 1 ). The conventional flow propagation velocity of early diastolic mitral inflow (Vp) was automatically measured by tracing the isovelocity contour between 45% and 55% of peak E from the mitral annulus to 4 cm into the LV cavity. In addition, the extent of propagation of early diastolic filling into the left ventricle was assessed by measuring the distance from the mitral annulus to the deceleration point of the initial E-wave propagation velocity ( Figure 1 ) by using a statistical change-point analysis method. The initial propagation velocity from the mitral annulus to the deceleration point (initial Vp) and after the terminal point (terminal Vp) were also measured.

The IVPD was estimated by using the CMMD data to integrate the one-dimensional Euler equation, as previously described. The pressure difference at each point along with the scan line was measured relative to the left atrium just above the mitral valve at the mitral annulus just before mitral valve opening by calculating the line integral between them. From the temporal profile of the IVPD, the peak total IVPD from the left atrium to the LV apex was calculated. This method has been validated by comparison with direct measurements made using micromanometers. In addition to the total IVPD from the left atrium to the LV apex, the basilar IVPD from the left atrium to the mid left ventricle (2 cm from the mitral annulus) and the apical IVPD from the mid left ventricle to the LV apex were also measured ( Figure 2 ).

Statistical Analysis
Continuous variables are expressed as mean ± SD and were compared by two-tailed unpaired t test between the HFpEF and HFrEF groups or by using one-way analysis of variance among the groups, and post hoc analysis was then performed by using the Tukey-Kramer test. Proportions were compared using χ 2 analysis. Linear regression analysis was used for the detection of correlation between two continuous variables. For all tests, P values <.05 were considered significant. All data were analyzed using JMP software (SAS Institute, Cary, NC).
Results
Patient Characteristics
The clinical characteristics of the study subjects are presented in Table 1 . Among the 151 patients with HF, 50 had preserved LV EFs (HFpEF) and 101 had reduced LV EFs (HFrEF). Patients with HFpEF and those with HFrEF were older and had higher systolic blood pressures than normal controls. Patients with HFrEF tended to have worse HF symptoms, more nonischemic cardiomyopathy, and less hypertensive heart disease than those with HFpEF. The use of angiotensin-converting enzyme inhibitors or angiotensin II receptor blockers and β-blockers was less frequent and that of calcium antagonists was more frequent in patients with HFpEF than in those with HFrEF.
Variable | Controls | Patients with HFpEF | Patients with HFrEF | P |
---|---|---|---|---|
( n = 28) | ( n = 50) | ( n = 101) | ||
Age (y) | 36 ± 15 | 60 ± 16 ∗ | 55 ± 13 ∗ | <.001 |
Women | 15 (52%) | 28 (56%) | 35 (35%) | .027 |
Body surface area (m 2 ) | 1.85 ± 0.25 | 1.98 ± 0.28 | 2.01 ± 0.24 ∗ | .013 |
Systolic blood pressure (mm Hg) | 119 ± 19 | 142 ± 24 ∗ | 132 ± 26 ∗ | <.001 |
Diastolic blood pressure (mm Hg) | 69 ± 10 | 74 ± 18 | 80 ± 19 ∗ | .020 |
Heart rate (beats/min) | 73 ± 17 | 77 ± 14 | 79 ± 14 | .11 |
Duration from HF worsening (d) | NA | 28 ± 67 | 75 ± 141 † | |
NYHA functional class | .006 | |||
I | NA | 0 (0%) | 0 (0%) | |
II | NA | 33 (66%) | 43 (43%) | |
III or IV | NA | 17 (34%) | 58 (57%) | |
Cardiac disease | <.001 | |||
Ischemic heart disease | NA | 10 (20%) | 30 (30%) | |
Nonischemic dilated cardiomyopathy | NA | 1 (2%) | 36 (36%) | |
Hypertensive heart disease | NA | 31 (62%) | 18 (18%) | |
Undefined | NA | 6 (12%) | 16 (16%) | |
Others | NA | 2 (4%) | 1 (1%) | |
Comorbidities | ||||
Hypertension | NA | 47 (94%) | 81 (80%) | .018 |
Diabetes mellitus | NA | 22 (44%) | 48 (48%) | .68 |
Dyslipidemia | NA | 8 (16%) | 17 (17%) | .90 |
Medications | ||||
ACE inhibitors or ARBs | NA | 23 (46%) | 71 (70%) | .004 |
βblockers | NA | 32 (64%) | 84 (83%) | .010 |
Calcium antagonists | NA | 16 (32%) | 13 (13%) | .006 |
Diuretics | NA | 31 (62%) | 60 (59%) | .76 |
Aspirin | NA | 23 (46%) | 47 (47%) | .91 |
Statin | NA | 21 (42%) | 57 (56%) | .09 |
∗ P < .05 versus normal controls by Tukey-Kramer post hoc test.
Comparison of the Echocardiographic Parameters
The echocardiographic parameters are summarized in Table 2 . LV end-diastolic and end-systolic volumes were larger and EFs were lower in patients with HFrEF than in controls and patients with HFpEF, whereas they were comparable between patients with HFpEF and controls. LA volume index was enlarged in patients with HFpEF compared with controls and further enlarged in patients with HFrEF.
Variable | Controls | Patients with HFpEF | Patients with HFrEF | P |
---|---|---|---|---|
( n = 28) | ( n = 50) | ( n = 101) | ||
Two-dimensional findings | ||||
LV mass index (g/m 2 ) | 78 ± 21 | 134 ± 51 ∗ | 154 ± 59 ∗ | <.001 |
LV end-diastolic volume (mL) | 86 ± 30 | 103 ± 39 | 170 ± 57 ∗ , † | <.001 |
LV end-systolic volume (mL) | 31 ± 13 | 39 ± 16 | 118 ± 49 ∗ , † | <.001 |
LV ejection fraction (%) | 64 ± 6 | 63 ± 7 | 32 ± 9 ∗ , † | <.001 |
LA volume index (mL/m 2 ) | 18.0 ± 6.0 | 31.8 ± 13.5 ∗ | 37.2 ± 12.4 ∗ , † | <.001 |
Doppler findings | ||||
E-wave velocity (cm/sec) | 83 ± 16 | 91 ± 28 | 92 ± 27 | .25 |
A wave velocity (cm/sec) | 64 ± 16 | 94 ± 31 ∗ | 64 ± 26 † | <.001 |
E/A ratio | 1.34 ± 0.41 | 1.08 ± 0.54 | 1.78 ± 1.05 ∗ , † | <.001 |
E-wave deceleration time (msec) | 192 ± 50 | 218 ± 60 | 167 ± 55 † | <.001 |
s′ (cm/sec) | 9.5 ± 2.3 | 6.8 ± 1.3 ∗ | 5.0 ± 1.1 ∗ , † | <.001 |
e′ (cm/sec) | 12.3 ± 2.4 | 6.4 ± 1.6 ∗ | 5.9 ± 1.7 ∗ | <001 |
E/e′ ratio | 6.8 ± 1.5 | 14.7 ± 4.6 ∗ | 16.3 ± 5.9 ∗ | <.001 |
Diastolic grade | <.001 | |||
Normal | 25 (89%) | 2 (4%) | 2 (2%) | |
I | 1 (4%) | 5 (10%) | 6 (6%) | |
II | 2 (7%) | 10 (20%) | 17 (17%) | |
III | 0 (0%) | 33 (66%) | 76 (75%) | |
CMMD findings | ||||
Vp (cm/sec) | 53.3 ± 30.2 | 46.2 ± 21.5 | 40.0 ± 20.8 ∗ | .018 |
Initial Vp (cm/sec) | 81.7 ± 45.9 | 71.1 ± 31.7 | 47.4 ± 20.2 ∗ , † | <.001 |
Terminal Vp (cm/sec) | 22.8 ± 20.9 | 16.9 ± 12.9 | 16.3 ± 9.9 | .085 |
Deceleration point of initial Vp (cm) | 3.7 ± 1.3 | 3.5 ± 1.2 | 2.9 ± 1.1 ∗ , † | <.001 |
Speckle-tracking findings | ||||
Longitudinal global strain (%) | −21.2 ± 3.0 | −16.6 ± 3.3 ∗ | −8.8 ± 2.8 ∗ , † | <.001 |
Longitudinal basal strain (%) | −20.9 ± 5.2 | −18.1 ± 5.1 ∗ | −11.5 ± 3.8 ∗ , † | <.001 |
Longitudinal apical strain (%) | −23.7 ± 5.6 | −19.6 ± 6.0 ∗ | −10.4 ± 4.2 ∗ , † | <.001 |
Longitudinal SR Sys (%) | −1.2 ± 0.2 | −1.0 ± 0.2 ∗ | −0.6 ± 0.2 ∗ , † | <.001 |
Longitudinal SR E (%) | 1.5 ± 0.4 | 1.0 ± 0.3 ∗ | 0.6 ± 0.2 ∗ , † | <.001 |
Circumferential global strain (%) | −29.3 ± 6.1 | −26.2 ± 7.7 | −12.4 ± 4.9 ∗ , † | <.001 |
Circumferential SR Sys (%) | −1.7 ± 0.4 | −1.6 ± 0.5 | −0.8 ± 0.3 ∗ , † | <.001 |
Circumferential SR E (%) | 2.2 ± 0.6 | 1.7 ± 0.6 ∗ | 1.0 ± 0.4 ∗ , † | <.001 |
LV twist (°) | 12.0 ± 3.6 ( n = 24) | 15.2 ± 8.1 ( n = 33) | 6.0 ± 3.6 ∗ , † ( n = 88) | <.001 |
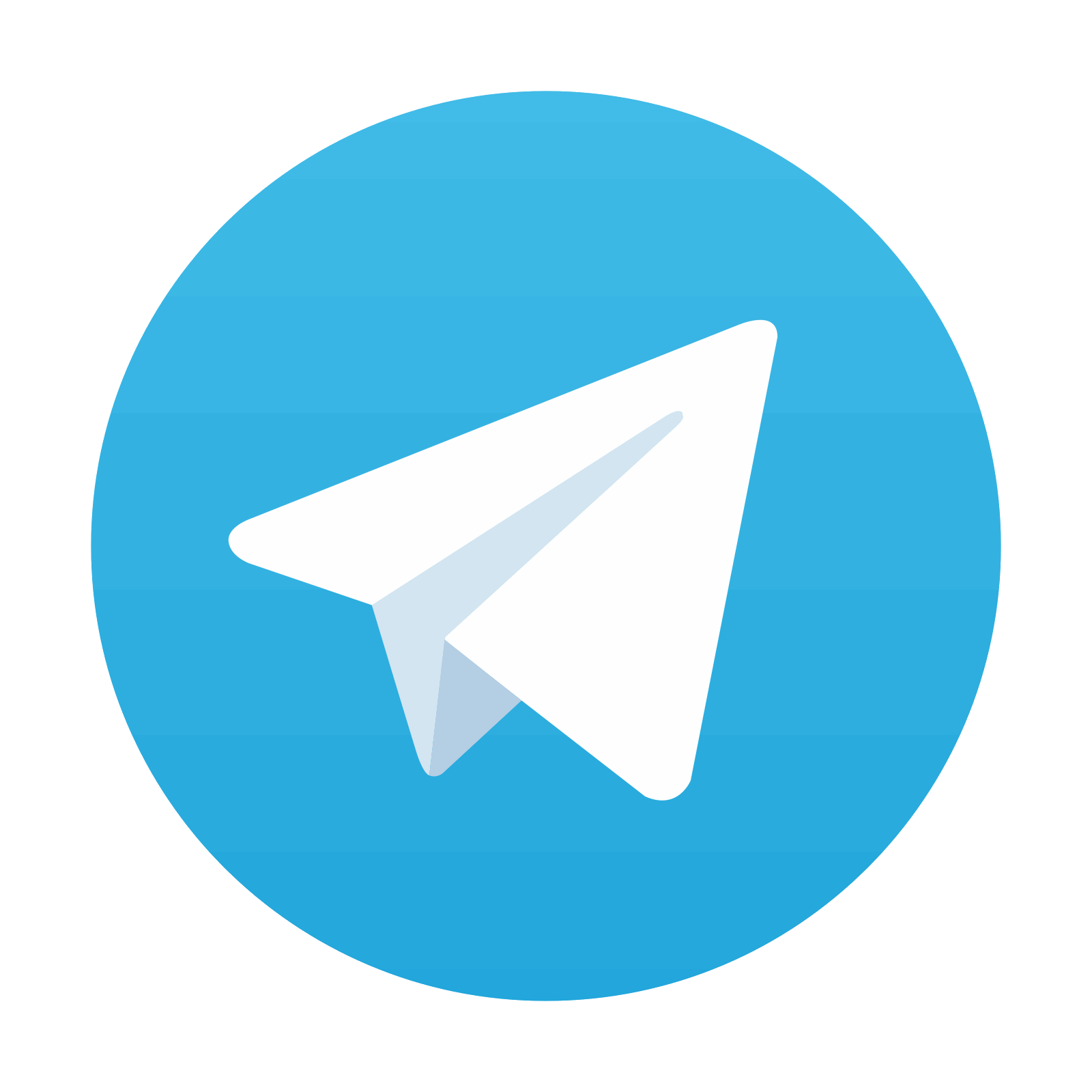
Stay updated, free articles. Join our Telegram channel

Full access? Get Clinical Tree
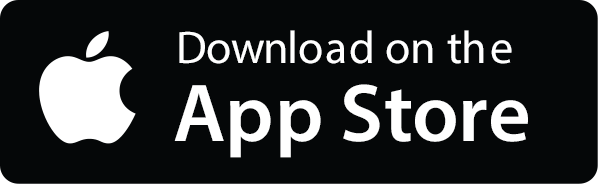
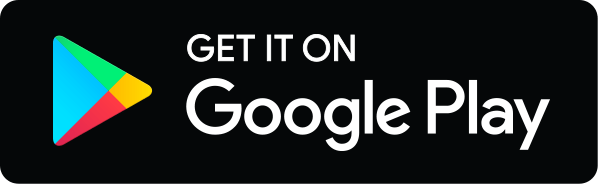
