Background
Changes in left ventricular (LV) torsion have been related to LV geometry in patients with concomitant long-standing myocardial disease or pulmonary hypertension (PH). We evaluated the effect of acute high altitude-induced isolated PH on LV geometry, volumes, systolic function, and torsional mechanics.
Methods
Twenty-three volunteers were prospectively studied at low altitude and after the second (D3) and third night (D4) at high altitude (4,559 m). LV ejection fraction, multidirectional strains and torsion, LV volumes, sphericity, and eccentricity were derived by speckle-tracking on three-dimensional echocardiographic data sets. Pulmonary pressure was estimated from the transtricuspid pressure gradient (TRPG), LV preload from end-diastolic LV volume, and transmitral over mitral annular E velocity (E/e′).
Results
At high altitude, oxygen saturation decreased by 15%–20%, heart rate and cardiac index increased by 15%–20%, and TRPG increased from 21 ± 2 to 37 ± 9 mm Hg ( P < .01). LV volumes, preload, ejection fraction, multidirectional strains, and sphericity remained unaffected, but diastolic (1.04 ± 0.07 to 1.09 ± 0.09 on D3/D4, P < .05) and systolic (1.00 ± 0.06 to 1.08 ± 0.1 [D3] and 1.06 ± 0.07 [D4], P < .05) eccentricity slightly increased, indicating mild septal flattening. LV torsion decreased from 2.14 ± 0.85 to 1.34 ± 0.68 ( P < .05) and 1.65 ± 0.54 ( P = .08) degrees/cm on D3/D4, respectively. Changes in torsion showed a weak inverse relationship to changes in systolic ( r = −0.369, P = .013) and diastolic ( r = −0.329, P = .032) eccentricity but not to changes in TRPG, heart rate or preload.
Conclusions
High-altitude exposure was associated with mild septal flattening of the LV and reduced ventricular torsion at unchanged global LV function and preload, suggesting a relation between LV geometry and torsional mechanics.
Highlights
- •
Effect of pulmonary artery pressure on left ventricular (LV) geometry and torsion was studied.
- •
Increased pulmonary pressure led to increased LV eccentricity and decreased torsion.
- •
A relation between LV geometry and torsion is suggested.
Trekking or climbing in the Alps, Andes, or the Himalaya has become increasingly popular in recent years. These activities often comprise a rapid ascent to high altitude, which can result in acute mountain sickness, cerebral edema, and exaggerated pulmonary hypertension (PH) as a result of hypoxic pulmonary vasoconstriction. The pulmonary hemodynamic changes associated with high altitude are characterized by an increased pulmonary capillary pressure but unchanged or only slightly raised wedge pressure, indicating normal left ventricular (LV) systolic and diastolic function. Consistent with hemodynamic data, LV ejection fraction (EF) has been shown to stay unchanged or increase upon rapid ascent to high altitude. Nevertheless, subtle defects in LV diastolic function have been described at high altitude and have been attributed to a shift of the interventricular septum (IVS) toward the left ventricle.
The left ventricle reshapes during the cardiac cycle to reduce its volume against the load imposed on it. Besides myocardial shortening and thickening, this deformation encompasses a wringing motion of the left ventricle along its longitudinal axis, referred to as LV twist or torsion. LV torsion is characterized by a small clockwise rotation of the base and a larger counterclockwise rotation of the apex, attributed to a dynamic interaction between shortening in nearly perpendicularly oriented myofibers in the subendocardial and subepicardial layers. By deforming the subendocardial fiber matrix in the cross-fiber direction (cross-fiber shortening), torsion during ejection is believed to act as a systolic amplification mechanism, contributing to the transformation of a 15%–20% shortening of the myofibers into a volume reduction of about 60% at the LV cavity level. Consistent with the dependence on myofiber shortening and myofiber orientation across and along the LV myocardium, a number of studies have identified not only myocardial loading and contractility, but also concentric remodeling to influence torsion in the heart. Studies examining the role of LV geometry have been scarce and limited to patients with long-standing myocardial disease, remodeling, and LV loading as potential confounders. High altitude-induced increases in pulmonary artery pressure do occur in otherwise healthy individuals. We therefore set out to assess the influence of short-term cardiac shape changes induced by PH on LV torsion in healthy volunteers rapidly ascending to high altitude. Given the three-dimensional (3D) nature of LV geometry and deformation as well as the advantage of 3D echocardiography with regard to plane alignment, 3D speckle-tracking echocardiography was used to calculate LV volumes, geometry, torsion, and multidirectional deformation.
Methods
Study Participants
In this prospectively designed study, we investigated 25 healthy volunteers 18–65 years old participating in a high-altitude expedition. Exclusion criteria included chronic medication, an abnormal baseline echocardiogram (mild valvular regurgitation accepted), or known cardiopulmonary and other chronic diseases. Furthermore, we excluded volunteers who had spent >5 nights above 2,500 m within the last 30 days. The study conformed to the Declaration of Helsinki and was approved by the Ethical Committee of the University of Zurich. All subjects gave written informed consent to participation. Two subjects were excluded because of poor echocardiographic windows.
Study Protocol
Four weeks after low-altitude measurements at the University Hospital of Zurich (low altitude, 450 m), participants travelled in groups of three to four individuals to Alagna (Italy, 1,205 m) and were carried by cable car to 2,900 m. They continued on foot to the Gnifetti hut (3,647 m), spending the night there. After an ascent of 4–6 hours they reached the Capanna Regina Margherita research facility (Italy, 4,559 m) on the following day (day 1). None of the participants used oxygen during the stay at high altitude. Echocardiography was performed following the first (D2), second (D3), and third night (D4) at the Margherita hut. Peripheral arterial O 2 saturation (SatO 2 ) was measured by pulse oximetry. Blood pressure was measured immediately prior to and resting heart rate was assessed during echocardiography.
Echocardiography
Transthoracic echocardiography was performed on an Aplio Artida (Toshiba Medical Systems, Tokyo, Japan) equipped with a phased-array two-dimensional (2D; PST-30SBT) and a matrix array 3D (PST-25SX) transducer. Doppler and 2D acquisitions were performed at end-expiratory breath hold, and measurements averaged over two to four beats. For the 3D-based analysis, wide-angled full-volume mode was used, and four to six subvolumes were acquired during a breath hold, resulting in a pyramidal four-dimensional data set at 17–25 frames per second. Echocardiography at low altitude was carried out over 8 days, and echocardiography at high altitude over 28 days by four different investigators (B.W.D.B., S.K., C.D., B.A.K.) who ascended to the Capanna Regina Margherita research facility together with the participants as described above. All echocardiographic studies were transferred to an offline computer for analysis. Measurements were performed by one single observer (B.W.D.B.) who was blinded to study participant identity but not to the altitude at which a particular study was performed (not possible due to higher heart rate at high altitude).
Doppler and 2D-Based Echocardiographic Analysis
From the mitral-inflow pattern, the early (E) and the effective late filling velocities and their ratio were determined. In case of fusion of the E and A wave, effective A was calculated as peak A velocity minus the residual E wave velocity at the onset of the A wave. The tissue Doppler early systolic (LV-s’) and diastolic (e’) velocity at the inferoseptal and lateral mitral annulus was averaged; the ratio E/e’ was considered to reflect LV filling pressure. Right atrial pressure was estimated from the inferior vena cava, and systolic and diastolic right ventricular (RV) pressure gradients (RVPGs and RVPGd) were derived from Doppler traces as described. Tricuspid regurgitation severity was graded visually using color flow Doppler. RV end-diastolic (RV-EDA), and end-systolic area (RV-ESA), fractional area change (RV-FAC), tissue Doppler-derived systolic velocity (RV-s’), and tricuspid annular plane systolic excursion (TAPSE) were measured on apical four-chamber (4Ch) views.
Three-Dimensional Speckle-Tracking Deformation Analysis
Three-dimensional data sets were analyzed using 3D wall motion tracking software (Toshiba Medical Systems) by a single, experienced investigator. The 3D data set was aligned along the LV maximal long axis crossing the true apex and the mitral annular plane. Then an image plane corresponding to an apical two-chamber (2Ch) view plane parallel to the IVS and a slightly modified apical 4Ch view plane perpendicular to the 2Ch plane and bisecting the IVS and three equally distributed short-axis sections were reconstructed ( Figure 1 ). After defining an endocardial region of interest adjusted to include trabeculae and papillary muscles in the LV, the endocardial surface and epicardial boundaries were defined semiautomatically. Finally, automatic speckle-tracking of the resulting 3D region of interest was performed and fine-tuned using the cine-loop play feature as described elsewhere. Global torsion was analyzed and compared with global LV strains and geometry. LV mass, end-diastolic (LVEDV) and end-systolic (LVESV) volumes, and LV stroke volume (SV) were derived from the volumetric data.

Three-Dimensional Speckle-Tracking-Based LV Geometry
LV sphericity was defined as the actual 3D LV volume divided by the volume of a sphere with the LV major long-axis length as the diameter. To express septal flattening on the global LV level, LV eccentricity was defined as (area 2Ch/area 4Ch). Local eccentricity was calculated from the basal, midventricular, and apical short-axis views as D-2Ch/D-4Ch, where D-2Ch and D-4Ch represent the local diameters in the 2Ch and 4Ch planes, respectively ( Figure 1 ). All measures were performed at end diastole and end systole using the speckle-tracking-derived endocardial boundaries on axis-corrected views.
Reproducibility
In a random sample of 20 studies, 3D speckle-tracking was repeated by the same observer (B.W.D.B.) and by a different observer (B.A.K.) who were both blinded to prior measurements. The coefficient of variation for intraobserver/interobserver variability ranged from 7.5%/9.8% for LV mass, to 7.5%/8.3% for LV-EDV, to 14%/16.1% for LVESV for 3D volumes and from 6.5%/8.3% (area strain) to 16.7%/16.5% (radial strain) for global LV strain measurements; the coefficient of variation was 24.0%/25.9% for LV torsion. Systematic bias expressed as a percentage of the initial measurement was ≤3.5% for all, except for radial strain (8.4%/8.1%). For LV diastolic/systolic eccentricity the coefficient of variation was 7.1%/9.7% with a bias ≤2.0%.
Statistical Analysis
Continuous variables are presented as mean ± SD, and categorical data as numbers and percentages. Because of a low number of technically adequate 3D acquisitions on D2 due to pronounced tachycardia, invalidating mountain sickness and resting dyspnea, D2 measurements were excluded from the analysis. Data were tested for normality using the Shapiro-Wilk test. As not all data were normally distributed, either the Friedmann test with post hoc analysis using Wilcoxon signed-rank tests with a Bonferroni correction or repeated measures ANOVA with Sidak-Bonferroni post hoc testing was used to test for differences in hemodynamics, LV geometry, deformation, and function among the three time points, as appropriate. When systolic and diastolic parameters were compared at a particular stage, a Wilcoxon signed-rank or a paired t -test was used. The relation between changes in LV torsion, hemodynamics, RV/LV functional parameters, exercise parameters, and LV geometry was assessed using linear regression analysis with Pearson correlation or Spearman’s rho. Statistical analyses were performed with SPSS version 24.0 (SPSS, Chicago, IL). A P value <.05 was considered statistically significant.
Results
Patient Characteristics
The final study population comprised 23 healthy (age 43 ± 9 years, eight female, body surface area 1.73 ± 0.16 m 2 , body mass index 24 ± 2 kg/m 2 ) volunteers. Resting hemodynamic data and changes induced by altitude are displayed in Figure 2 . Exposure to altitude was associated with a drop in SpO 2 of 15%–20%, which appeared to be largely compensated for by a rise in cardiac output of 15%–20% ( Figure 2 ). Most of this increased cardiac output was due to a comparable rise in resting heart rate, while SV did not change. Exposure to high altitude markedly augmented RVPG (RVPGs/RVPGd), from a mean of 21/4 mm Hg at baseline to a mean of 38/13 and 36/12 mm Hg at D3 and D4, respectively ( Figure 2 ). Systemic blood pressures remained unchanged ( Figure 2 ).

LV and RV Function by Echocardiography
Echocardiographic characteristics at low and at high altitude are shown in Table 1 . Compared with the low-altitude measurements, LV torsion was impaired at high altitude. After correction for multiple comparisons, statistical significance was retained on D3. On D4, LV torsion tended to increase again, albeit with a strong trend to being impaired relative to baseline ( P = .08). Conversely, systolic annular velocities significantly increased. Other than in torsion and systolic annular velocities, no changes were noticed between low and high altitude on the LV side, whether considering parameters reflecting overall LV pumping and diastolic function, individual strain components, or the volumes needed to maintain systolic function. On the RV side, altitude exposure was associated with increases in RV-EDA and RV-ESA, which reached significance at D3. Except for RV-s’ at D4, RV systolic function at altitude did not significantly differ compared with low altitude. At low altitude, 21 and two subjects had trace and mild tricuspid regurgitation, respectively. At high altitude, tricuspid regurgitation was mild in seven and trace in 16 subjects ( P = .13 vs low altitude). None of the subjects developed moderate or severe tricuspid regurgitation at high altitude.
Low altitude | High altitude, D3 | High altitude, D4 | |
---|---|---|---|
Barometric pressure, mm Hg | 722 | 444 | 444 |
RV EDA, cm 2 | 20 ± 4.6 | 22 ± 4.3 ∗ | 22 ± 4.6 |
RV ESA, cm 2 | 11 ± 3.0 | 13 ± 3.0 † | 12 ± 2.9 |
RV FAC, % | 46 ± 9.9 | 42 ± 8.3 | 45 ± 9.0 |
RV-s’, cm/sec | 15.7 ± 3.0 | 16.7 ± 3.4 | 18.5 ± 0.8 ∗ , ‡ |
TAPSE, mm | 26 ± 5 | 25 ± 3 | 27 ± 5 |
LV mass, mg/mL | 154 ± 33 | 153 ± 33 | 155 ± 30 |
LV EDV, mL | 117 ± 22 | 114 ± 27 | 118 ± 22 |
LV ESV, mL | 50 ± 11 | 50 ± 15 | 49 ± 12 |
LV SV, mL | 67 ± 13 | 64 ± 13 | 69 ± 13 ‡ |
LV EF, % | 57 ± 4.6 | 57 ± 4.3 | 59 ± 5.2 |
LV-s’, cm/sec | 9.7 ± 1.7 | 11.5 ± 2.0 ∗ | 12.3 ± 2.0 † |
E/A eff | 1.4 ± 0.3 | 1.5 ± 0.6 | 1.6 ± 0.6 |
e’, cm/sec | 10.7 ± 2.2 | 10.8 ± 3.2 | 13.3 ± 6.0 † |
E/e′ | 6.9 ± 1.5 | 6.7 ± 1.8 | 6.7 ± 1.5 |
Diastolic 4Ch area, cm 2 | 30.3 ± 4.0 | 29.3 ± 5.2 | 30.0 ± 4.3 |
Diastolic 2Ch area, cm 2 | 31.3 ± 4.1 | 31.7 ± 4.8 | 32.6 ± 4.4 |
Systolic 4Ch area, cm 2 | 19.5 ± 2.8 | 19.0 ± 3.6 | 21.4 ± 5.4 |
Systolic 2Ch area, cm 2 | 19.5 ± 2.9 | 20.5 ± 3.7 | 19.7 ± 3.3 |
Circumferential strain, % | −28.3 ± 4.0 | -27.6 ± 3.1 | −29.2 ± 4.6 |
Longitudinal strain, % | −16.0 ± 2.8 | -16.4 ± 1.8 | −17.3 ± 2.1 |
Area strain, % | −41.5 ± 4.5 | -41.3 ± 3.2 | −43.2 ± 4.6 |
Radial strain, % | 34.8 ± 7.1 | 32.5 ± 7.5 | 35.6 ± 6.7 |
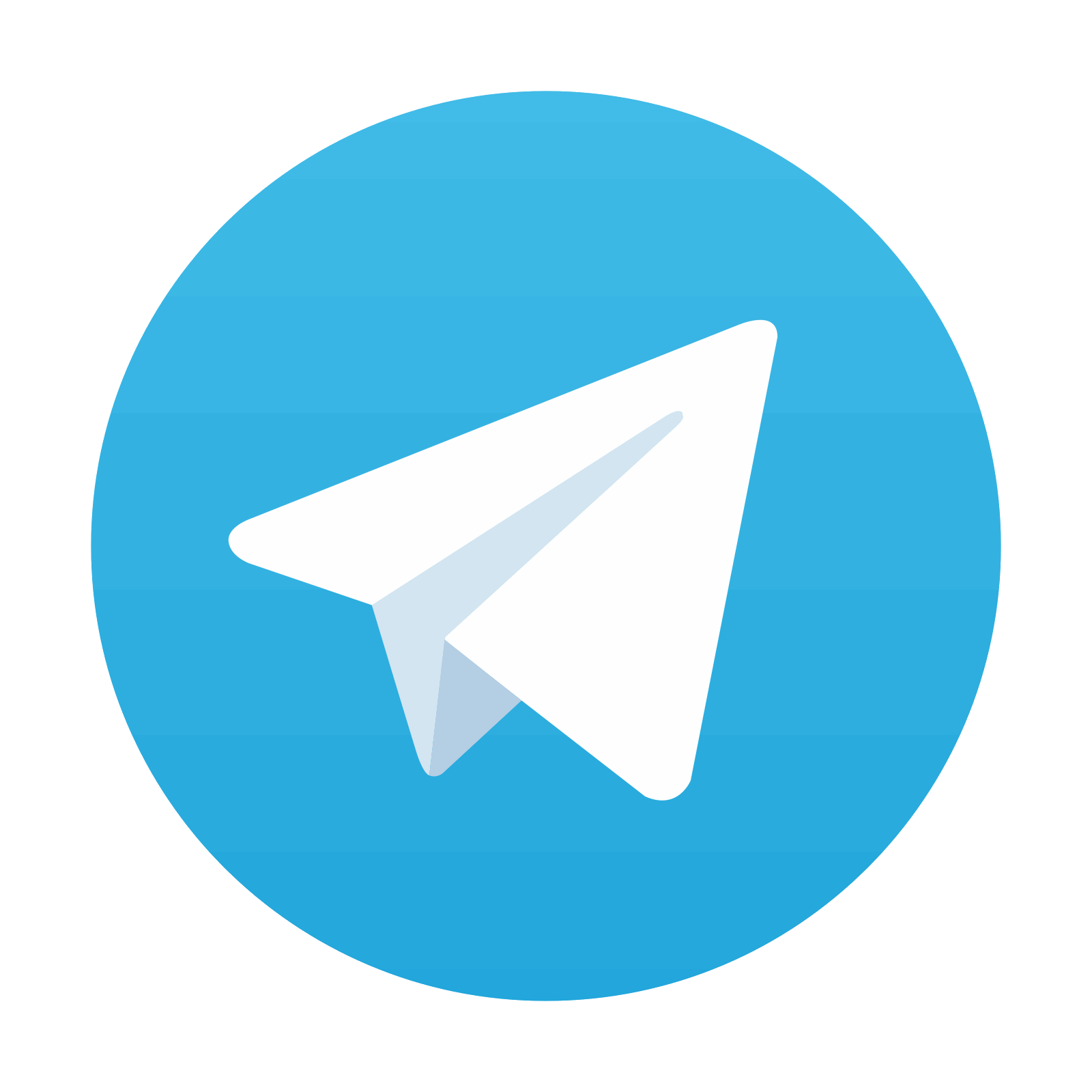
Stay updated, free articles. Join our Telegram channel

Full access? Get Clinical Tree
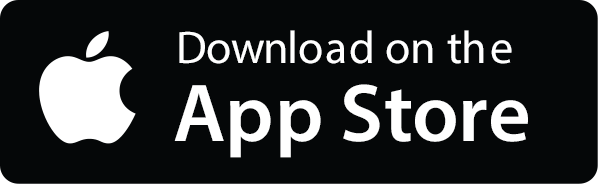
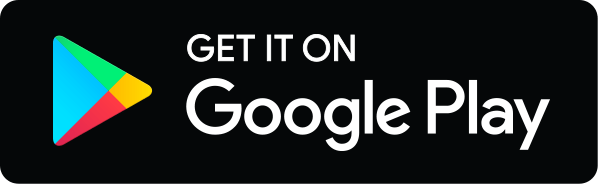
