Outline
Epidemiology of Chronic Kidney Disease and the Impact on Heart Failure, 214
Worsening Kidney Function and Prognosis, 214
Pathophysiology of Chronic Kidney Disease in Heart Failure, 215
Peripheral Volume Sensors, 215
Renal Sympathetic Nerves, 217
Central Venous Pressure as a Determinant of Kidney Function, 218
Neurohormonal Regulation of Kidney Function, 218
The Renin-Angiotensin-Aldosterone System, 218
Catecholamines, 218
Natriuretic Peptides, 218
Arginine Vasopressin, 219
Prostaglandins, 219
Biomarkers of Kidney Dysfunction, 220
Summary and Future Directions, 220
Control of circulating blood volume is a tightly regulated physiological process and is critical for maintaining cardiovascular homeostasis. Under normal homeostatic conditions, there is extensive cross-talk between the kidney and the heart (the “cardiorenal axis”) that is essential for regulation of salt and water homeostasis. However, as will be discussed here, in the setting of heart failure (HF), the normal mechanisms that control sodium and water balance become dysregulated secondary to the activation of neurohormonal pathways that lead to increased sympathetic activity ( see Chapter 13 ) and increased activation of the renin-angiotensin system, with a resultant increase in peripheral vasoconstriction and sodium and water reabsorption.
As discussed in Chapter 13 , a decline in cardiac function results in a change in the “effective” arterial blood volume of the peripheral circulation, which can lead to a decrease in kidney function. For example, among 156,743 US veterans with HF and an estimated glomerular filtration rate (eGFR) ≥60 mL/min/1.73m 2 , adults with HF had a 2.12-, 2.06-, and 2.13-fold higher multivariable-adjusted risk of incident chronic kidney disease (CKD), composite of CKD or mortality, and rapid eGFR decline, respectively. The kidney acts as both a bystander and a contributor to several maladaptive processes, to maintain organ perfusion. However, reduced kidney function as a consequence of reduced cardiac output and reduced kidney blood flow is only one component of the complex cardiorenal interaction. Substantial evidence also supports the role of additional pathways, such as neurohormonal mechanisms ( see Chapter 5, Chapter 6, Chapter 13 ) and venous congestion, in the deterioration of kidney and cardiac function.
Epidemiology of Chronic Kidney Disease and the Impact on Heart Failure
Kidney dysfunction is commonly seen in both stable patients and those with acute decompensated heart failure (ADHF). Ezekowitz and colleagues demonstrated that almost 40% of outpatients with both coronary artery disease and HF have Stage 3 or higher CKD, defined as an eGFR less than 60 mL/min/1.73m 2 . The prevalence of Stage 3 or higher CKD was as high as 64% among patients admitted for ADHF.
The presence of CKD is one of the most important prognostic factors for patients with HF, regardless of whether the ejection fraction (EF) is preserved or reduced ( Fig. 15.1 ). In a prospective cohort of 754 adults referred to an outpatient HF clinic, a 1% increase in 1-year mortality was seen for every 1 mL/min decrease in creatinine clearance. In an observational study of 24,331 adults with HF, Smith and colleagues found a graded association of eGFR with hospitalization for HF, all-cause hospitalization, and mortality. The association was similar between patients with preserved (n = 14,579) and reduced (n = 9762) EF. Importantly, patients with CKD experienced the same reduction in mortality from treatment with angiotensin-converting enzyme inhibitors and beta-blockers as did patients with normal kidney function, although they were less likely to receive the medications.

The presence of CKD is also associated with poor short-term outcomes. Data from the Acute Decompensated HF National Registry (ADHERE) showed that in-hospital mortality increased from 1.9% among patients with normal kidney function to 7.6% and 6.5% among patients with baseline CKD and kidney failure, respectively. While GFR remained a significant predictor of mortality after adjusting for confounders, the blood urea nitrogen (BUN) level remained the single best predictor. Other investigators have confirmed the usefulness of BUN as a marker of increased risk. In the Acute and Chronic Therapeutic Impact of a Vasopressin Antagonist in Chronic Heart Failure (ACTIV in CHF trial), patients with a baseline BUN greater than 40 mg/dL experienced an event rate of 30%, whereas patients whose baseline BUN less than 18 mg/dL had an event rate of 8.6%. It is likely that, in the acute setting, BUN is a marker of neurohormonal activation, which leads to constriction of the afferent arteriole, secretion of vasopressin, and enhanced reabsorption of sodium, water, and urea. These pathways will be discussed in more detail as follows.
Worsening Kidney Function and Prognosis
Worsening kidney function (WKF) among patients admitted with ADHF is often defined as an increase in creatinine ≥0.3 mg/dL. As many as one-third of patients may experience WKF, which typically occurs in the first 3 to 4 days of admission, but has also been shown to occur in the week after hospital discharge. In the Prospective Trial of Intravenous Milrinone for Exacerbations of Chronic Heart Failure (OPTIME-CHF), 12% of participants experienced a greater than 25% decrease in eGFR and 39% experienced a greater than 25% increase in BUN. In a meta-analysis of eight randomized controlled trials of renin-angiotensin-aldosterone system (RAAS) inhibitors in stable outpatients (6 HFrEF, 1 HFpEF and 1 that included both populations), the incidence of WKF was slightly higher in HFrEF compared with HFpEF (12% vs. 7%). Risk factors for WKF include baseline creatinine greater than 1.5 mg/dL, diabetes, and pulmonary edema.
While earlier studies suggested that WKF portended a worse prognosis, more recent analyses suggest that the association of WKF with prognosis is highly dependent on the clinical context. For example, in a study of 599 adults admitted to the hospital with ADHF, the development of WKF was associated with 1-year mortality only in patients who also had signs on physical exam of congestion at the time of hospital discharge. In a study of 1232 adults admitted for ADHF, Salah and colleagues compared the relative contribution of a decrease in N-terminal-pro-B-type natriuretic peptide (NT-pro-BNP) and WKF to prognosis. The authors found that WKF was not associated with 180-day mortality or readmission to the hospital for CVD among patients who had at least a 30% reduction in their NT-pro-BNP. In addition, the change in NT-pro-BNP did not predict development of severe WKF (defined as an absolute increase in serum creatinine ≥0.5 mg/dL), suggesting that severe WKF is multifactorial, and not simply explained by overly aggressive diuresis. Ibrahim and colleagues also evaluated the relative importance of NT-pro-BNP versus WKF in a randomized controlled trial of biomarker-guided HF therapy for patients with class II-IV HF with reduced ejection fraction (HFrEF). The authors found that adults who achieved NT-pro-BNP levels less than 1000 pg/mL were on higher doses of RAAS inhibitors, regardless of WKF. Further, patients with WKF but an NT-pro-BNP less than 1000 pg/mL experienced significantly fewer events at 1 year (worsening HF, hospitalization for HF or CVD death) compared with patients with no WKF but an NT-pro-BNP greater than 1000 pg/mL. Paradoxically, in the Diuretic Optimization Strategies Evaluation (DOSE) trial, a prospective multicenter trial investigating strategies of loop diuretic administration, increases in serum creatinine were associated with a lower risk for death, emergency room visits within 60 days, or rehospitalization (hazard ratio [HR] = 0.81 per 0.3 mg/dL increase, 95% CI 0.67–0.98, P = .026). Moreover, compared with those with stable kidney function, there was a strong association between improved kidney function (n = 28) and the composite endpoint (HR = 2.52, 95% CI = 1.57–4.03, P < .001). Taken together, available data suggest that for both acute and chronic management of HFrEF, it is important to prioritize decongestion and the use of evidence-based therapy, such as RAAS inhibitors, even with modest degrees of WKF.
There are few studies comparing the risk of WKF in patients with HFpEF versus HFrEF. In a meta-analysis of eight RCTs of RAAS inhibitors versus placebo (6 HFrEF, 1 HFpEF, 1 both), adults with HFrEF who were randomized to RAAS inhibitors and had WKF experienced higher rates of HF hospitalization, compared with patients who experienced no WKF (RR, 1.19 [1.08–1.31]; P < .001). However, the risk associated with WKF in patients allocated to placebo was larger (RR, 1.48 [1.35–1.62]; P < .001), and significantly different from patients randomized to RAAS inhibitors with WKF ( P for interaction = .005). Adults with HFpEF who were taking RAAS inhibitors also experienced a higher risk of hospitalization if they developed WKF compared with those without WKF (RR 1.78, 95% CI 1.43–2.21). However, unlike adults with HFrEF, WKF for those on placebo did not confer an increased risk of hospitalization. While is it possible that the pathophysiology of WKF differs between HFrEF and HFpEF, it is likely that the use of RAAS inhibitors simply unmasked greater disease severity.
Pathophysiology of Chronic Kidney Disease in Heart Failure
The homeostatic relationship between the heart and kidneys with respect to maintenance of body fluid homeostasis has been appreciated for centuries. Indeed, Sir William Withering noted that patients with “dropsical” conditions, characterized by the swelling of soft tissues due to the accumulation of excess water, often experienced a brisk diuresis following the administration of foxglove. In the early phases of HF, the ratio of the glomerular filtration rate to kidney blood flow (referred to as the filtration fraction) is maintained, and can even be increased. This is largely due to vasoconstrictor peptides, such as arginine vasopressin (AVP), angiotensin II, and norepinephrine (NE), which help maintain GFR by maintaining blood pressure and constricting the efferent arteriole resulting in increased intraglomerular capillary pressure ( Fig. 15.2 ). The remainder of the chapter will review some of the normal physiology that controls salt and water homeostasis, and some of the cardio-kidney pathways activated and contributed to a failing heart in clinical HF.

Peripheral Volume Sensors
Baroreceptors and Mechanoreceptors
Numerous pressure receptors (baroreceptors, mechanoreceptors) are located in critical areas in the circulatory tree—the carotid sinus, aortic arch, afferent glomerular arterioles in the kidney, and the superior and inferior vena cava. The receptors detect alterations in pressure and stretch of the vessel wall. In volume-depleted states, where stretch is decreased, there is a subsequent loss of tonic inhibition by the parasympathetic nervous system, which results in reflex increase sympathetic neural tone ( Fig. 15.3 ). The baroreceptors of the carotid body and aortic arch contribute to the antinatriuretic response observed in HF patients. Studies have demonstrated a reduction in urinary output and sodium excretion following volume expansion in the presence of carotid sinus excitation, despite a constant arterial pressures.

The atria also possess baroreceptors of two types: (1) type A, located primarily at the entrance of the pulmonary veins, which discharge at the onset of systole and are not affected by volume; and (2) type B, which demonstrate increased activity with atrial filling and increase atrial size. The neuronal inputs of the cardiac atria and the carotid body are transmitted to the medulla and hypothalamus through cranial nerves IX and X. Atrial transmural pressure has a significant influence on kidney function. Experiments in dogs show that decreased atrial wall distension culminated in decreased sodium excretion and urine flow rate by the kidney. In addition, the normal diuretic response to volume expansion was attenuated when atrial wall distension was limited. Other studies examine the interaction between the carotid body and neuronal control of sodium homeostasis. For example, infusion of hypertonic saline increased neural activity along the tracts, leading to the hypothalamus and medulla. Conversely, increased left atrial stretch decreased activity of these neural tracts, and with a resultant increase in the urinary flow rate and sodium excretion. Plasma levels of atrial natriuretic peptide (ANP) were increased, while AVP and aldosterone levels were decreased. The atria and ventricles have their own baroreceptors that stimulate secretion of ANP and brain natriuretic peptide (BNP) in response to atrial or ventricular distension. ANP release can stimulate up to a 10-fold increase in sodium excretion. ANP and BNP will be discussed in more detail as follows.
The Juxtaglomerular Apparatus
The juxtaglomerular apparatus of the kidney serves as a intrarenal baroreceptor that is composed of four basic elements: the terminal portion of the afferent arteriole, the macula densa (a segment of the distal tubule), the extraglomerular mesangial region, and the efferent arteriole at the glomerulus (summarized in Fig. 15.4 ). Because of its location in the nephron, it is highly sensitive to changes in volume as induced by various diuretic classes, and thus it is sensitive to changes in kidney perfusion pressure. The juxtaglomerular apparatus is also known to be adrenergically innervated, and has β-1 adrenoreceptors.

Sympathetic stimulation or decreases in volume or perfusion pressure can all stimulate the juxtaglomerular apparatus response to release renin into the afferent arteriole. Renin then converts angiotensinogen, which is formed in the liver, to angiotensin I ( see also Chapter 5 ). Angiotensin-converting enzyme converts angiotensin I to angiotensin II, which is a potent vasoconstrictor and stimulates secretion of aldosterone from the adrenal cortex. Other factors that stimulate the renin-angiotensin-aldosterone system are noted in Table 15.1 . Most beta-blockers used clinically can cause adverse hemodynamic alterations in the kidney, including a decline in kidney blood flow and GFR, and a reduction of renin release with a subsequent antinatriuretic response, most prominently with upright posture. However, neither nebivolol nor carvedilol appears to have adverse effects on renal hemodynamics.
Inhibit Activity | Stimulate Activity |
---|---|
Arginine vasopressin | Activation of renal sympathetic nerves |
Angiotensin II | Catecholamines |
Salt loading | Hypotension |
β-adrenoceptor antagonists | Baroreceptor stimulation |
Potassium | Hypoperfusion |
Adenosine | Prostaglandin E and I series |
Hypocalcemia | Upright posture |
Hyperkalemia | Hypovolemia |
Renal Sympathetic Nerves
Renal sympathetic nerve activity has been found to be intimately related to body fluid homeostasis. Immunofluorescent electron microscopic and histochemical studies have demonstrated kidney nerves in the afferent and efferent arterioles, proximal and distal tubules, ascending limb of the loop of Henle, and the juxtaglomerular apparatus. Dopaminergic receptors have also been found in the tubules and kidney nerves. Increased kidney sympathetic activity leads to a decrease in kidney blood flow and a reduction in urinary sodium excretion. These effects are mediated, in part, by α1-adrenoreceptors. Renal sympathetic activity is also modulated by salt intake. Low-salt diets increase and high-salt diets decrease nerve activity. Increased kidney sympathetic nerve activity has been documented in states of volume (salt) depletion, and contributes to the associated elevation of plasma renin activity, reduction in kidney blood flow, and the subsequent antinatriuretic state.
Given the importance of sympathetic activity in HF, there is interest in applying kidney denervation to this population. Catheter-based kidney denervation involves catheterization of the femoral artery, and then applying radiofrequency energy circumferentially to the endothelium of the distal kidney artery. Pilot studies of up to 60 participants have not shown any adverse safety events over 6 to 12 months follow-up. Some studies have shown significant improvements in ejection fraction, 6-minute walk, and NT-pro-BNP levels, while others have shown neutral effects. No studies with sham controls have been reported.
Central Venous Pressure as a Determinant of Kidney Function
Early animal models have confirmed the association between central venous pressure (CVP) and kidney function. As early as 1931, Winton used a canine model to demonstrate that urine production decreased substantially at venous pressures of 20 mm Hg, and almost completely stopped at pressures greater than 25 mm Hg. Chronic thoracic vena cava obstruction has been used as a model in dogs to study the association of elevated pressures in HF with kidney function. Hemodynamic changes in the dogs included a decrease in cardiac output, GFR, and kidney plasma flow.
Studies in patients with heart failure demonstrate that an elevated CVP identifies those patients who will progress to develop WKF based on cardiac hemodynamic parameters. In the Evaluation Study of Congestive Heart Failure and Pulmonary Artery Catheterization Effectiveness (ESCAPE) trial—a comparison of pulmonary artery catheter-guided treatment for acute HF versus clinical assessment—there was no correlation between pulmonary capillary wedge pressure (PCWP), cardiac index (CI), or systemic vascular resistance with baseline kidney function. Right atrial pressure was the only hemodynamic measure that was significantly associated with baseline kidney function. In a retrospective analysis of more than 2500 adults who underwent a right heart catheterization, Damman and colleagues found that, above a CVP of 6 mm Hg, there was a progressive decline in kidney function. In a study of 145 patients hospitalized for ADHF, Mullens and colleagues found that the mean baseline CVP in patients who developed WKF was significantly higher than those who did not develop WKF (18 ± 7 mm Hg vs. 12 ± 6 mm Hg, P < .001). Interestingly, the mean CI was higher in those who developed WKF compared with those who did not (2.0 ± 0.8 vs. 1.8 ± 0.4 L/min/m , P = .008), again challenging the notion that CKD in HF is primarily secondary to poor forward flow.
Research on the association of venous congestion and CKD has also extended to studies of intra-abdominal pressure (IAP). For example, among 40 consecutive patients admitted with ADHF, 60% had an elevated IAP, defined as ≥8 mm Hg. Of note, none of the patients complained of abdominal symptoms on admission or during therapy. Overall, mean arterial pressure, CVP, PCWP, and CI were comparable between those with and without an elevated IAP. However, those with an elevated IAP had a higher baseline creatinine (2.3 ± 1.0 mg/dL vs.1.5 ± 0.8 mg/dL, P < .009) and at follow-up (1.8 ± 0.8 mg/dL vs. 1.3 ± 0.9 mg/dL, P < .04). Among the patients with elevated IAP at baseline, improvement in kidney function was associated with an improvement in IAP, and not any other hemodynamic measurements.
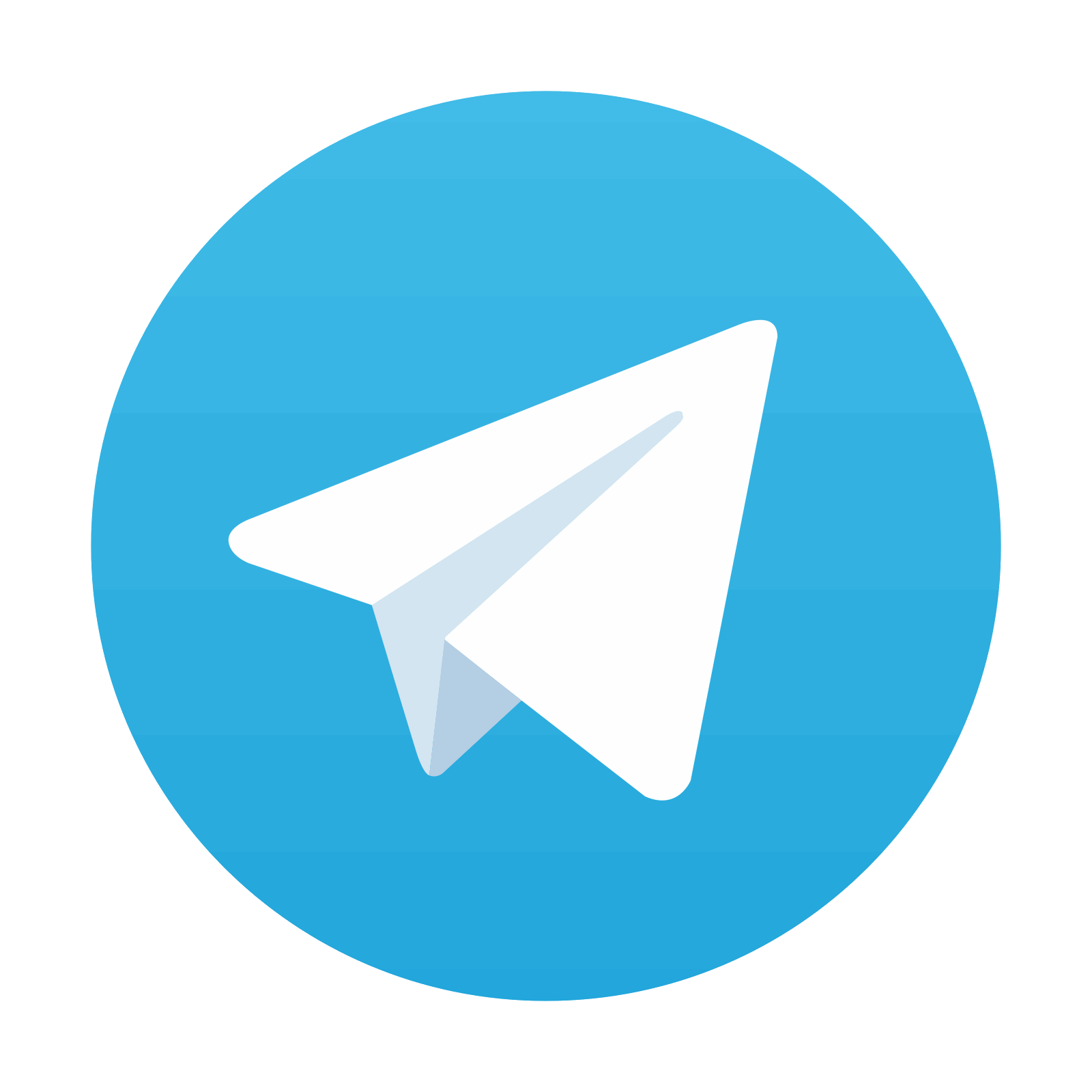
Stay updated, free articles. Join our Telegram channel

Full access? Get Clinical Tree
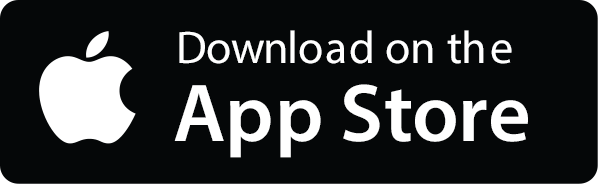
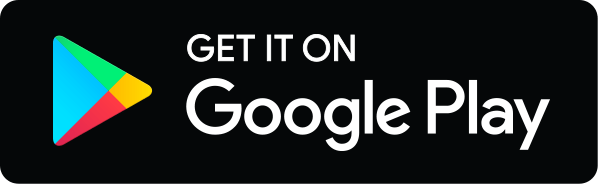