Background
It has been suggested that myocardial systolic impairment may not be accurately detected by the evaluation of endocardial excursion alone. The aim of this study was to test the hypothesis that changes in left ventricular (LV) subendocardial and subepicardial strain are sensitive markers of severity of aortic stenosis (AS) and LV function in patients with AS.
Methods
Transthoracic echocardiography was performed in 73 consecutive patients with AS who had preserved systolic function and in 20 controls. Longitudinal strain, subendocardial radial strain, subepicardial radial strain, and transmural radial strain were measured using LV apical and short-axis images.
Results
The 73 patients enrolled in this study were classified according to AS severity: mild ( n = 10), moderate ( n = 15), or severe ( n = 48). Although transmural and subepicardial radial strain showed similar values in all groups, subendocardial radial strain and longitudinal strain could differentiate mild or moderate AS from severe AS. Only the ratio of subendocardial to subepicardial radial strain (the bilayer ratio) decreased significantly as the severity of AS increased. Bilayer ratio showed weak correlations with LV ejection fraction ( r = 0.37) and E/E′ ratio ( r = −0.33) and moderate correlations with LV mass ( r = −0.55) and aortic valve area ( r = 0.71). Moreover, bilayer ratio was independently associated with AS severity ( P = .001). In 21 patients who underwent aortic valve replacement, subendocardial radial strain and bilayer ratio increased 7 days after surgery, whereas other echocardiographic parameters of LV function showed no improvement.
Conclusions
Bilayer ratio can reliably differentiate patients with varying degrees of AS severity and is a sensitive marker of LV function. These findings suggest that the evaluation of subendocardial and subepicardial radial strain might be a novel method for assessing LV mechanics in patients with AS.
The prevalence of symptomatic aortic stenosis (AS) increases with age, and if left untreated, AS results in a high mortality rate. In these patients, aortic valve replacement improves survival, left ventricular (LV) ejection fraction, and regression of LV mass. However the management of asymptomatic patients with severe AS remains controversial, particularly with respect to the timing of surgical intervention. Because of the adaptive remodeling of the left ventricle, patients can remain asymptomatic or minimally symptomatic for prolonged periods of time, despite the presence of severe AS. However, once LV function is impaired, outcomes after surgical intervention are suboptimal. Hence, it is of crucial clinical importance to identify the onset of LV dysfunction in its early phase so that surgical intervention can be performed in a timely manner. The most commonly used ejection phase indices, such as ejection fraction, may not be early indicators of cardiac alteration. As a result, the development of more accurate, noninvasive methods for assessing subclinical LV dysfunction is necessary in patients with AS.
It is well known that the subendocardial layer of the left ventricle plays an important role in ventricular function. In normal subjects, the subendocardium contributes more to the overall myocardial thickening and uses more oxygen than the subepicardium. As a result, when myocardial blood flow decreases, the subendocardium is affected to a greater degree than the subepicardium. In addition, transmural thickening is not uniform, with the endocardial portion of the wall thickening more than the epicardial portion, in part because of nonuniform transmission of end-systolic stress. Previous reports have suggested that myocardial systolic impairment in LV hypertrophy may originate at the endocardial side and then extend toward the epicardium. Therefore, the evaluation of differences in subendocardial and subepicardial LV deformation might be useful in detecting subclinical LV systolic dysfunction in patients with LV hypertrophy secondary to AS.
The aim of this study was to examine (1) whether the severity of AS affects subepicardial and subendocardial deformation (i.e., subendocardial and subepicardial radial strain), (2) whether changes in subepicardial and subendocardial radial strain may reflect subclinical changes in LV function in patients with AS, and (3) whether the assessment of subendocardial and subepicardial radial strain may detect an early phase of recovery of LV function after aortic valve replacement.
Methods
Study Population
A total of 168 patients who had different level of AS severity and were planned to undergo coronary angiography and LV pressure recordings were recruited for this study from a patient population referred to our hospital between March 2007 and March 2010. Exclusion criteria were obstructive epicardial coronary artery disease (>50% reduction in absolute luminal diameter of a major artery or major branch vessel), ejection fraction ≤ 50% by echocardiography, regional myocardial wall motion abnormalities, left bundle branch block, atrial fibrillation or flutter, renal insufficiency, moderate or severe hypertension (diastolic blood pressure > 100 mm Hg and/or systolic blood pressure > 160 mm Hg), significant mitral valve stenosis and regurgitation or aortic valve regurgitation, and history of coronary bypass surgery or valve surgery. Finally, 80 patients (33 men; mean age, 85 ± 9 years) were included in this study population. All patients underwent complete transthoracic echocardiography at baseline, and patients who underwent aortic valve replacement had follow-up echocardiographic studies 7 days and 6 months after surgery. Controls were an age-matched and gender-matched population from the same region who had undergone echocardiography and had normal echocardiographic findings. Written consent for the study was obtained from all patients. This study protocol was approved by the institutional ethics committee.
Echocardiographic Measurements
Conventional transthoracic echocardiographic examinations were performed using commercially available ultrasound transducer and equipment (S5-1, iE33; Philips Medical Systems, Andover, MA). All two-dimensional grayscale echocardiographic images were obtained using the second harmonic mode. Standard two-dimensional measurements were performed, including the thickness of the interventricular septum and posterior wall at end-diastole, LV dimension at end-diastole, and LV dimension at end-systole. LV ejection fraction was calculated using the modified biplane Simpson method as recommended by the American Society of Echocardiography. LV mass and LV mass index were calculated using the formula proposed by Devereux and Reichek. Fractional shortening and midwall fractional shortening were calculated using standard formulas. Continuous-wave Doppler recordings were obtained from multiple views, including apical, right parasternal or clavicular, and subcostal views, and the peak and mean aortic valve velocity profiles were measured. LV outflow tract velocity was measured using pulsed-wave Doppler with the sample volume in the LV outflow tract just below the region of proximal flow convergence. Aortic valve area (AVA) was calculated using the continuity equation according to standard protocol. The study population was divided into three groups on the basis of echocardiographic AVA: the mild AS group included patients with AVAs > 1.5 cm 2 , the moderate AS group included patients with AVAs ≥ 1.0 and ≤ 1.5 cm 2 , and the severe AS group included patients with AVAs < 1.0 cm 2 . Early diastolic transmitral velocity (E) and late diastolic transmitral velocity (A) were recorded by pulsed-wave Doppler in the apical four-chamber view. Early diastolic tissue velocity (E′) and the diastolic tissue velocity (A′) were recorded in the same view with the sample volume positioned at the lateral mitral annulus. Measurements were averaged over at least three cardiac cycles. An experienced operator blinded to the clinical data performed these measurements.
Longitudinal and Radial Strain Measurements
Two-dimensional LV apical four-chamber, two-chamber, long-axis, and parasternal short-axis (at the level of the papillary muscle) images were recorded to obtain strain measurements. Three consecutive cardiac cycle loops were recorded, and the measurements were averaged. The frame rate was kept between 70 and 100 Hz, and offline software (Syngo Velocity Vector Imaging [VVI]; Siemens Medical Solutions USA, Inc., Mountain View, CA) was used to analyze the Digital Imaging and Communications in Medicine–formatted grayscale images. B-mode pixel-tracking algorithms for measuring strain have been previously validated. Recently, measurements of subendocardial and subepicardial function by strain analysis were reported. For the measurements of transmural radial strain, a circular region of interest was traced on the endocardium using the end-diastolic frame. Then, a second larger concentric circle was automatically generated and manually adjusted near the epicardium. Finally, a point of reference was placed at the center of the cavity for the calculation of radial strain. For the measurement of subendocardial radial strain, the endocardial tracing remained the same, but the second larger concentric circle was manually adjusted in the midwall of the myocardium using the end-diastolic frame. For the measurement of subepicardial radial strain, the first circular region of interest was traced on the midwall of the myocardium, and the second larger concentric circle was manually adjusted near the epicardium. For the measurement of longitudinal strain, the subendocardial border was traced manually. A region of interest was manually adjusted to include the entire myocardial thickness. VVI software automatically divided the LV mid short-axis image into six equal segments and the apical images into 18 segments and then generated time-strain curves from each segment. The maximum strain values of the mid six segments of the left ventricle were averaged and taken as the radial and longitudinal strain values for each patient ( Figures 1 and 2 ).


Cardiac Catheterization
All patients underwent left-sided cardiac catheterization within 7 days of echocardiography, including coronary angiography and LV pressure recordings. Patients with mild to moderate AS underwent catheterization on suspicion of angina pectoris and those with severe AS for aortic valve evaluation.
Reproducibility of the VVI Method
Interobserver and intraobserver variability was assessed for longitudinal, transmural, subendocardial, and subepicardial radial strain measurements by VVI software in 10 randomly selected subjects. Interobserver variability was calculated as the SD of the differences between the measurements of two independent observers who were unaware of the other patient data and expressed as a percentage of the average value. Intraobserver variability was calculated as the SD of the differences between the first and second determinations (2-week interval) for a single observer and expressed as a percentage of the average value.
Statistical Analysis
Continuous variables are expressed as mean ± SD. Differences of continuous and normally distributed variables among groups were compared using analysis of variance. Differences between two groups were assessed using χ 2 or Fisher’s exact tests for categorical variables, and comparisons of continuous variables were made using unpaired Student’s t tests. Serial measurements within each group were compared using paired Student’s t tests. Correlation coefficients between various measurements and strain parameters were determined by linear regression analysis. Variables with P values < .10 on univariate analysis were incorporated in to the multiple linear regression model to identify determinants of the ratio of subendocardial to subepicardial radial strain (bilayer ratio). Differences were considered significant when the P value was < .05. All statistical analyses were performed using StatView version 5.0 for Windows (SAS Institute Inc., Cary, NC).
Results
Patient Characteristics
Among the 80 consecutive patients who had met eligibility criteria, seven patients were excluded from this study because of inadequate image quality for the measurement of strain values. The remaining 73 patients (29 men, 44 women; mean age, 84 ± 8 years) were classified into three groups: 10 with mild AS, 15 with moderate AS, and 48 with severe AS. Characteristics of the 73 patients and 20 controls are shown in Table 1 . As expected, there were significant differences regarding New York Heart Association class, relative wall thickness, stroke volume, midwall fractional shortening, E/E′ ratio, LV mass index, and peak and mean pressure gradient among the all groups.
Variable | AS | Control ( n = 20) | P | ||
---|---|---|---|---|---|
Mild ( n = 10) | Moderate ( n = 15) | Severe ( n = 48) | |||
Age (y) | 82 ± 10 | 80 ± 9 | 86 ± 7 | 82 ± 8 | .10 |
Men | 6 | 6 | 17 | 12 | .20 |
NYHA classification | |||||
I | 10 (100%) | 13 (87%) | 24 (50%) ∗,†,‡ | 20 (100%) | <.001 |
II | 0 (0%) | 2 (13%) | 18 (38%) ∗,†,‡ | 0 (0%) | <.001 |
III | 0 (0%) | 0 (0%) | 5 (10%) | 0 (0%) | .18 |
IV | 0 (0%) | 0 (0%) | 1 (2%) | 0 (0%) | .82 |
Body surface area (m 2 ) | 1.9 ± 0.2 | 1.8 ± 0.3 | 1.7 ± 0.2 | 1.8 ± 0.2 | .29 |
Systolic blood pressure (mm Hg) | 139 ± 26 | 131 ± 27 | 137 ± 22 | 130 ± 22 | .71 |
Diastolic blood pressure (mm Hg) | 65 ± 12 | 62 ± 12 | 60 ± 11 | 62 ± 11 | .67 |
LV systolic pressure (mm Hg) | 166 ± 18 | 171 ± 26 | 190 ± 29 † | — | — |
LV end-diastolic pressure (mm Hg) | 22 ± 5 | 18 ± 11 | 18 ± 6 | — | — |
Heart rate (beats/min) | 69 ± 16 | 68 ± 10 | 66 ± 9 | 66 ± 10 | .73 |
Echocardiography | |||||
LV end-diastolic volume/body surface area (mL/m 2 ) | 46 ± 8 | 48 ± 13 | 48 ± 12 | 47 ± 10 | .88 |
LV end-systolic volume/body surface area (mL/m 2 ) | 14 ± 4 | 15 ± 8 | 14 ± 6 | 16 ± 6 | .88 |
Relative wall thickness | 0.47 ± 0.12 | 0.51 ± 0.08 | 0.61 ± 0.15 ∗,†,‡ | 0.44 ± 0.09 | <.001 |
Stroke volume (mL) | 85 ± 20 | 73 ± 22 | 66 ± 21 ∗,† | 86 ± 18 | <.001 |
LVEF (%) | 63 ± 5 | 61 ± 2 | 61 ± 4 | 62 ± 4 | .53 |
MWFS (%) | 16 ± 3 | 15 ± 3 ∗ | 14 ± 3 ∗,†,‡ | 18 ± 3 | <.001 |
E/E′ ratio | 12 ± 3 | 15 ± 10 | 22 ± 8 ∗,†,‡ | 11 ± 4 | <.001 |
LV mass index (g/m 2 ) | 78 ± 15 | 104 ± 20 ∗ | 137 ± 31 ∗,†,‡ | 75 ± 21 | <.001 |
AVA (cm 2 ) | 1.6 ± 0.5 | 1.1 ± 0.1 † | 0.7 ± 0.2 †,‡ | — | — |
Peak PG (mm Hg) | 29 ± 11 ∗ | 50 ± 19 ∗,† | 82 ± 24 ∗,†,‡ | 9 ± 3 | <.001 |
Mean PG (mm Hg) | 16 ± 8 ∗ | 30 ± 12 ∗,† | 48 ± 17 ∗,†,‡ | 5 ± 2 | <.001 |
Longitudinal strain (%) | −18 ± 3 | −17 ± 3 ∗ | −14 ± 3 ∗,†,‡ | 20 ± 3 | <.001 |
Transmural radial strain (%) | 34 ± 13 | 34 ± 11 | 30 ± 9 ∗ | 35 ± 8 | .18 |
Subendocardial radial strain (%) | 56 ± 5 | 49 ± 16 ∗ | 37 ± 10 ∗,†,‡ | 59 ± 10 | <.001 |
Subepicardial radial strain (%) | 24 ± 5 | 28 ± 8 | 28 ± 7 ∗ | 22 ± 5 | .38 |
Bilayer ratio § | 2.4 ± 0.5 ∗ | 1.7 ± 0.3 ∗,† | 1.3 ± 0.3 ∗,†,‡ | 2.7 ± 0.6 | <.001 |
Longitudinal and Radial Strain Parameters
Longitudinal, transmural, subendocardial, and subepicardial radial strain and bilayer ratio at baseline are shown in Table 1 . Although there were no differences among groups for transmural and subepicardial radial strain, bilayer ratio decreased significantly as the severity of AS became more serious ( Figures 1 and 2 ). Longitudinal strain and subendocardial radial strain could not differentiate mild and moderate AS patients. In addition, linear regression analysis showed the relationship between AS parameters and strain parameters ( Table 2 ). Although there were weak correlations between longitudinal strain or subendocardial radial strain and LV ejection fraction, LV mass index, AVA, and peak pressure gradient, these correlations were stronger for bilayer ratio. Moreover, there was a moderate correlation between bilayer ratio and E/E′ ratio that was not seen with longitudinal strain or subendocardial radial strain. By multivariate linear analysis, AVA was independently associated with bilayer ratio ( Table 3 ).
Variable | Longitudinal strain | Subendocardial strain | Bilayer ratio ‡ |
---|---|---|---|
LV systolic pressure | −0.20 | −0.059 | −0.24 |
LV end-diastolic pressure | 0.18 | 0.16 | 0.17 |
LVEF | 0.25 ∗ | 0.25 ∗ | 0.37 ∗ |
E/E′ ratio | −0.16 | −0.15 | −0.33 ∗ |
LV mass index | −0.30 ∗ | −0.35 ∗ | −0.55 † |
AVA | 0.45 † | 0.50 † | 0.71 † |
Peak PG | −0.25 ∗ | −0.24 ∗ | −0.54 † |
Mean PG | −0.15 | −0.17 | −0.50 † |
Variable | β | SE | P | Model R 2 |
---|---|---|---|---|
Age | −0.030 | 0.006 | .75 | 0.58 ( P < .001) |
LV systolic pressure | −0.036 | 0.002 | .73 | |
Relative wall thickness | 0.035 | 0.39 | .74 | |
Stroke volume | 0.13 | 0.002 | .19 | |
LV mass index | −0.035 | 0.002 | .77 | |
E/E′ ratio | 0.072 | 0.006 | .49 | |
AVA | 0.70 | 0.19 | <.001 |
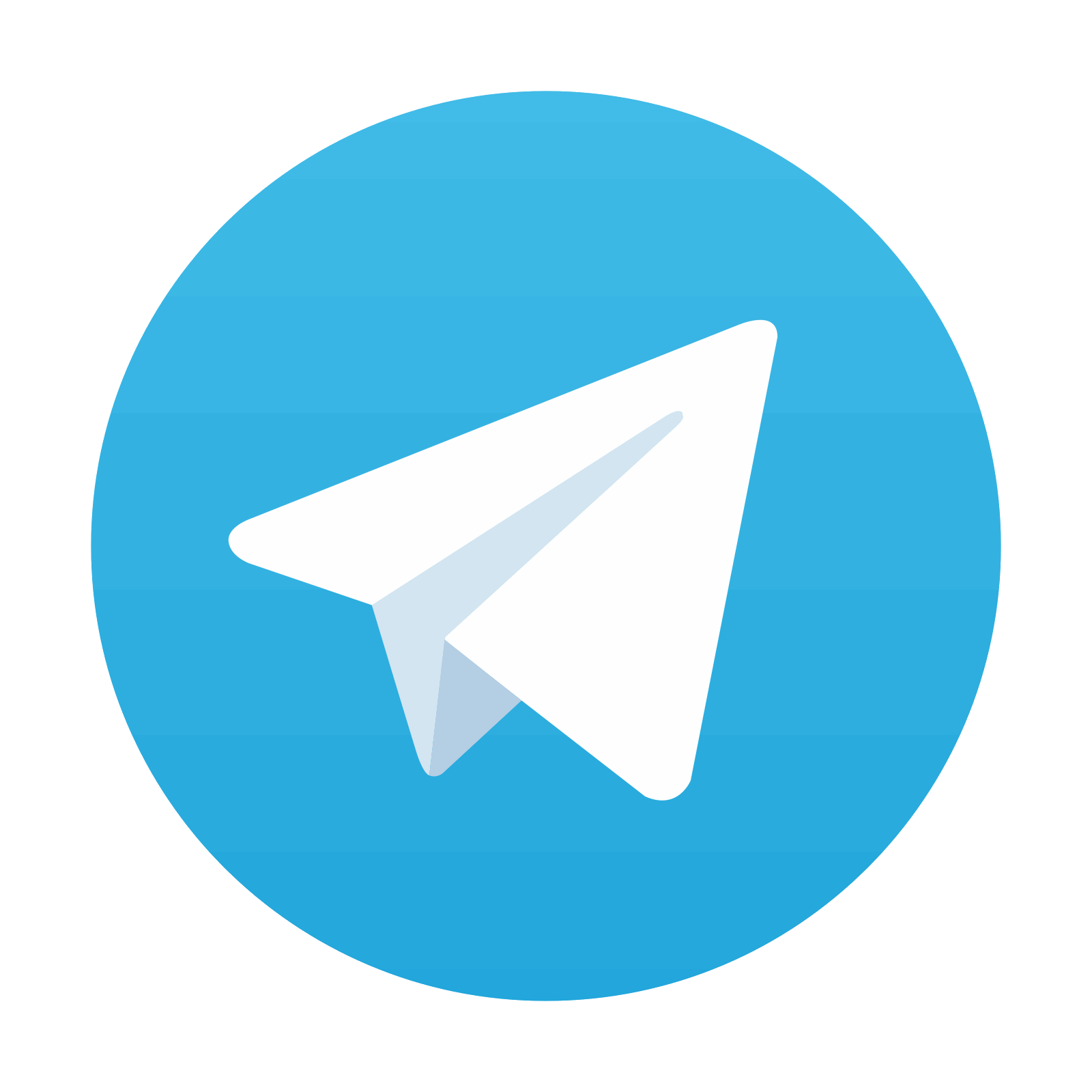
Stay updated, free articles. Join our Telegram channel

Full access? Get Clinical Tree
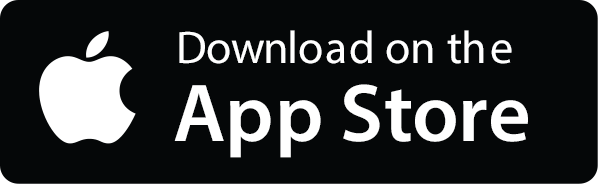
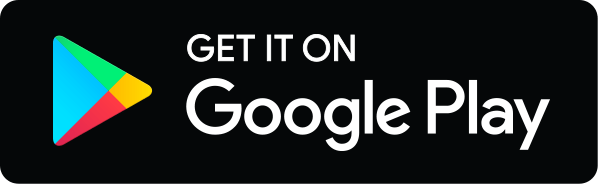
