Chronic obstructive pulmonary usually is subcategorized into 2 groups: chronic bronchitis and emphysema. The main cause of chronic bronchitis and emphysema is smoking; however, alpha1-antitrypsin also has been seen to cause emphysema in patients who are deficient. As symptoms and lung function decline, treatment modalities, such as lung volume reduction surgery, have been used in individuals with chronic obstructive pulmonary disease and upper lobe predominant emphysema. This article analyzes multiple published series where lung volume reduction surgery has been used in individuals with alpha1-antitrypsin deficiency and their overall outcomes.
Key points
- •
There are 5 published series of severe alpha 1 -antitrypsin disease (AATD) patients who underwent lung volume reduction surgery (LVRS) and 3 published series of severe AATD patients who underwent endoscopic lung volume reduction (ELVR).
- •
The improvement in forced expiratory volume in the first second of expiration (FEV 1 ) was on average not sustained, such that by 12 months to 24 months only 2 LRVS and 1 ELVR series reported that the FEV 1 remained higher than the baseline value.
- •
In the 4 series with data at 3 months to 8 months postprocedure, 4 found a statistically significant decrease in dyspnea scores and there was a statistically significant decrease in dyspnea scores in 3 of 6 studies by 12 months to 24 months postprocedure.
Introduction to chronic obstructive pulmonary disease
Chronic Obstructive Pulmonary Disease/Emphysema: Pathology and Physiology
Chronic obstructive pulmonary disease (COPD) is described as airflow obstruction that not always is reversible and usually is subcategorized into 2 groups: chronic bronchitis and emphysema. , In 2015, the Global Burden of Disease study estimated global prevalence of COPD at approximately 174 million cases, which likely represents an undercount. The same year, COPD ranked as third leading cause of death, with 3.2 million people dying from the disease.
Chronic bronchitis and emphysema are differentiated based on anatomy. Chronic bronchitis is defined by permanent obstruction of the airways, with a forced expiratory volume in the first second of expiration (FEV 1 ) to forced vital capacity (FVC) ratio of less than 70%. Emphysema is an anatomic description of the destruction of alveolar walls beyond the terminal bronchioles. Centrilobular emphysema is associated with cigarette smoking, whereas panacinar (panlobular) emphysema commonly is found in individuals with alpha 1 -antitrypsin disease (AATD). Both patterns can be seen in the same patient.
The main cause of COPD is smoking. Smoke inhalation causes inflammatory cells to infiltrate the bronchiole mucosa, submucosa, and glandular tissue, leading to increased mucus, hyperplasia of epithelial cells, bronchial wall thickening, and impairment of tissue repair. These lead to airway remodeling and emphysema with loss of elastic recoil, which subsequently cause a decrease in FEV 1 , enhance gas trapping, and lead to hyperinflation and dyspnea. ,
Treatment
Smoking cessation
Smoking cessation is imperative to reduce the accelerated decline of lung function over time as well as the associated exacerbations and comorbidities. If initiated early, smoking cessation can decrease the incidence of respiratory morbidity. The Lung Health Study in 1994 found that in individuals with mild or moderate COPD (FEV 1 >50%), intensive smoking cessation programs were associated with improvements in lung function at 5 years. Various modalities, both pharmacologic and nonpharmacologic, are available to help with smoking cessation.
Bronchodilators
Maintenance pharmacotherapy is used in those with stable COPD to improve symptoms and quality-of-life, decrease exacerbations and improve exercise tolerance. Inhaled long-acting β 2 -agonists (LABAs), long-acting antimuscarinic antagonists (LAMAs), and inhaled corticosteroids (ICSs) are used individually or in combination for maintenance therapy. Inhaled LABA and ICSs have been known to decrease exacerbations. The addition of LAMA to LABA/ICS therapy is associated with fewer exacerbations than LABA/ICSs alone.
Anti-inflammatory drugs
Patients whose COPD remains poorly controlled despite maximal inhaler regimens may require oral pharmacotherapy. Roflumilast is a phosphodiesterase-4 inhibitor that may reduce airway inflammation and bronchoconstriction. , Roflumilast decreases exacerbations requiring corticosteroid treatment but has not been shown to improve life expectancy or quality of life. Macrolide antibiotics have been studied for continuous or intermittent use in COPD and may decrease exacerbations and hospitalizations as well as improve quality of life.
Exacerbations, antibiotics, and systemic steroids
Exacerbations of COPD usually involve increased sputum, cough, and dyspnea. , Mild exacerbations can be treated with bronchodilators, moderate exacerbations require antibiotics and often systemic steroids, and severe exacerbations often require hospitalization. Exacerbations are associated with worsening disease progression, decreased quality of life, and increased risk of mortality. The most frequent cause for COPD exacerbations is a viral or bacterial infection. In those with severe exacerbations requiring hospital admission, 78% of the cases were identified as being cause by infection.
Current guidelines recommend, in the absence of contraindications, that oral corticosteroids should be used with other therapies in all patients hospitalized with acute exacerbations. The role of systemic steroids in the outpatient setting is unclear. A 5-day duration of corticosteroids is noninferior to a 14-day course. ,
Supplemental oxygen and non-invasive ventilation
In 1980, the Nocturnal Oxygen Therapy Trial Group compared continuous oxygen therapy (24 h/d) with 12-hour nocturnal oxygen therapy in individuals with hypoxemic COPD. At 2-year follow-up, continuous oxygen therapy was found associated with lower mortality compared with the nocturnal oxygen group. , In 1981, the UK Medical Research Council (MRC) trial randomly allocated patients with COPD and severe hypoxemia to receive oxygen (at least 15 h/d) or no oxygen. , The 5-year difference in mortality was 21% in favor of those using supplemental oxygen. These 2 trials established long-term oxygen therapy as the standard of care. For years it was assumed that oxygen for nocturnal and exercise-induced hypoxemia also was beneficial. In 2018, it was shown that the use of supplemental oxygen in patients with exercise-induced hypoxemia (but normal resting oxygen levels) improved exercise capacity but had no impact on survival or quality of life. Similarly, the role of supplemental nocturnal oxygen for patients with isolated nocturnal hypoxemia recently has been called into question. Despite this, the use of supplemental oxygen for exercise-induced hypoxemia and isolated nocturnal hypoxemia remains widespread.
Pulmonary rehabilitation
Pulmonary rehabilitation (PR) improves exercise tolerance in those patients with COPD. PR is recommended for patients with COPD who also have a modified (mMRC) dyspnea scale score of greater than or equal to 2. In these individuals, PR increased exercise capacity and quality of life and decreased dyspnea and BODE index. , PR has been described as a cost-effective way to reduce hospital admissions and mortality in patients who have had severe exacerbations. Despite this, PR remains underutilized worldwide.
Prognosis and Mortality Risk
Mortality from COPD is higher for men, those with severe disease, and those greater than 45 years of age. Prognosis after hospitalization is poor. The mortality rate during hospitalization for a COPD exacerbation ranges from 2.5% to 10%. This increases with time post-hospitalization, with a mortality rate of 16% to 19% at 3 months post-hospitalization, 23% to 43% at 1 year post-hospitalization, and 55% to 60% at 5 years. Comorbidities also are a major cause of hospitalization and death in COPD patients. The only factors that have been shown to yield a decrease in mortality have been smoking cessation and long-term oxygen therapy in those with chronic respiratory failure from COPD.
Alpha 1 -antitrypsin disease
History
René Laennec described the clinical features, pathology, diagnosis, and treatment of emphysema in his 1826 Treatise on Mediate Auscultation and Diseases of the Chest . , His views on emphysema heavily influenced pulmonary physicians for the next 135 years. By the 1960s, researchers were beginning to examine the molecular basis for emphysema, focusing on the destruction of the connective tissue scaffolding of emphysematous lungs. In 1964, Laurell and Eriksson published their seminal work on AATD. They analyzed 1500 samples of serum via paper electrophoresis and discovered that 5 samples lacked a band, which they correctly identified as AATD ( Fig. 1 ). Three of the 5 cases were symptomatic, having developed pulmonary emphysema at a young age. Two of the patients lacked obvious pulmonary disease but 1 of these had a familial history of pulmonary emphysema. Further work by Eriksson demonstrated the heterogeneous nature of disease in patients with AATD, with some experiencing severe early-onset obstructive lung disease and others manifesting no clinical symptoms. Laurell and Eriksson’s discovery of the role of AATD in the pathogenesis of panacinar emphysema led to the so-called proteolytic theory of emphysema, in which proteases are released by pulmonary neutrophils and macrophages, leading to excessive proteolytic activity in the lungs and subsequent tissue destruction. This theory gained support when it was demonstrated in 1964 that emphysema could be induced in rat lungs by the intratracheal instillation of papain into rat lungs.

Pathophysiology of Alpha 1 -antitrypsin Disease
A more detailed understanding of the genetics and biochemistry of AATD has been elucidated since Laurell and Eriksson’s initial findings in 1963. AATD is a member of the serine protease inhibitor (SERPIN) superfamily. Most people possess 2 copies of the wild-type M-allele of the SERPINA1 gene, which encodes the AATD protein. People with the Pi∗MM genotype, where Pi stands for protease inhibitor, have normal serum levels of functional AATD. AATD primarily is synthesized within hepatocytes, although it also is produced within intestinal cells, pulmonary alveolar cells, white blood cells, and the cornea. It is synthesized within the endoplasmic reticulum before being trafficked via the Golgi apparatus and ultimately secreted into the plasma. AATD most commonly is the result of deficiency alleles, in which a mutant AATD protein is synthesized, with the majority being destroyed within the endoplasmic reticulum prior to secretion (70%) and smaller proportions being secreted (15%) or going on to form polymers (15%), which themselves are degraded via autophagy or secreted into the plasma. The Z (Glu342Lys) allele is 1 such deficiency allele and is responsible for 95% of severe AATD cases. The Z allele is found in 1 in 25 people with European ancestry and 1 in 2000 people with European ancestry are homozygotes for Z (Pi∗ZZ), making AATD 1 of the most common inherited disorders among white persons ( Fig. 2 ). Other deficiency alleles result in misfolded proteins, varying degrees of polymerization, intracellular degradation, abnormal posttranslational biosynthesis, and unstable protein structure. The S (Glu264Val) allele is even more common than the Z allele, with a carrier frequency of 1:4 people in the Iberian Peninsula, 1:5 people in southern Europe, and 1:30 people in the United States. Individuals with Pi∗SS, Pi∗MZ, and Pi∗SZ generally have AATD levels reduced to 40% to 60% of normal, compared with 15% of normal for Pi∗ZZ individuals. They may be at increased risk of emphysema but it is not as severe as in Pi∗ZZ homozygotes. In addition to the deficiency alleles, there are several null (Pi∗QO) alleles, in which there is either no detectable AATD mRNA, or a truncated, nonfunctional protein is synthesized but not secreted.

In the lungs, AATD inhibits neutrophil elastase, a proteolytic enzyme thought to be the key enzyme involved in the pathologic degradation of pulmonary elastin. Instillation of neutrophil elastase in model animals leads to airspace enlargement, and neutrophil elastase knockout mice are protected from smoking-induced emphysema. AATD has been shown to inhibit neutrophil elastase in vivo and prevent lung digestion by leukoproteases in vitro. AATD has a 1000-fold more rapid rate of association with neutrophil elastase than with trypsin, further supporting neutrophil elastase inhibition as its most important function. Among AATD patients, it is thought that insufficient quantities of slower-acting mutant AATD reach the lungs, leading to unchecked activity of neutrophil elastase and resultant tissue destruction ( Fig. 3 ). Polymerized Z AATD has a proinflammatory effect in the lungs, attracting neutrophils and increasing proteolytic activity, ultimately leading to structural damage and increased susceptibility to infection. Oxidation also plays an important role in inactivation of AATD. A methionine residue at the active site of AATD may be oxidized in the presence of chemical oxidants, myeloperoxidase, or cigarette smoke. AATD methionine oxidation results in a loss of biologic activity. This discovery led to the development of the oxidation hypothesis, which seeks to explain smoker’s emphysema in patients with normal plasma levels of AATD as being due to increased inactivation of AATD by oxidative stress from cigarette smoke.

Systemic Disease in Alpha 1 -antitrypsin Disease
Patients with AATD also are susceptible to liver disease, although the mechanism differs from the loss-of-function seen in AATD emphysema. Misfolded Z AATD polymerizes within hepatocytes, resulting in proteotoxic stress and gain-of-function liver disease. Clinically significant liver fibrosis is seen in 20% to 35% of Pi∗ZZ AATD patients. In addition to the more common emphysema and liver disease, several systemic disorders also have been associated with AATD in persons with the Pi∗ZZ genotype. Severe adult asthma, necrotizing panniculitis, antineutrophil cytoplasmic vasculitis (ANCA)-associated vasculitis, chronic kidney disease, diabetes, and metabolic alterations all are overrepresented in patients with the Pi∗ZZ allele. ,
Lung Disease in Alpha 1 -antitrypsin Disease
AATD-related pulmonary emphysema is seen most commonly in Pi∗ZZ genotypes (95% of cases). Penetrance is approximately 60% in Pi∗ZZ individuals. AATD-related pulmonary emphysema in Pi∗ZZ individuals is thought to be responsible for approximately 1% to 2% of all COPD cases. Symptoms largely are indistinguishable from the garden variety smoker’s emphysema seen in individuals with normal serum AATD levels, making underdiagnosis and diagnostic delays common. Pulmonary symptoms, such as cough, dyspnea, and sputum production, may be seen by the fourth to fifth decades of life. Bronchial hyperreactivity may be seen in up to 20% of symptomatic patients, and bronchodilator response is a risk factor for FEV 1 decline. Early-onset obstructive lung disease with imaging findings of lower lobe–predominant panacinar emphysema in a patient with moderate smoking history is the classic clinical description of AATD-associated lung disease ( Fig. 4 ). Recent studies have shown that approximately two-thirds of AATD subjects have a predominantly basal pattern whereas approximately one-third have greater involvement in the apical regions. Presumably, the basilar predilection among AATD patients is due to the effects of gravity on pulmonary perfusion and the migration of hematogenous inflammatory cells, although the exact mechanism remains unknown. Likewise, approximately three-quarters of AATD-related emphysema subjects were noted to have panacinar emphysema, whereas approximately one-third were noted to have centrilobular emphysema. Approximately 27% of AATD-associated emphysema patients have bronchiectasis on CT.

Due to the lack of specific demographic and clinical characteristics, it is recommended that all patients with COPD should be tested for AATD, regardless of smoking history. Testing also is recommended in patients with unexplained chronic liver disease, severe adult asthma, unexplained bronchiectasis, ANCA and systemic vasculitis, and relapsing panniculitis. Severe AATD deficiency is defined as serum levels less than 35% of the mean expected value (80 mg/dL, or 11 μmol/L measured by radial immunodiffusion).
Treatment of Alpha 1 -antitrypsin Disease
Response to bronchodilators, ICSs, PR, supplemental oxygen therapy, and immunizations is not significantly different than in AATD-replete COPD patients. As such, treatment algorithms are not significantly different for AATD-associated emphysema compared with AATD-replete COPD. Every effort should be made to prevent exposure to tobacco or to facilitate cessation in those who smoke given increased rates of decline in lung function compared with those with AATD-replete COPD. Intravenous augmentation therapy with purified preparations of pooled donor-derived human AATD is recommended for patients with an FEV 1 in the range of 30% to 65% of predicted. Intravenous augmentation has been shown to decrease elastin degradation and rates of loss of CT lung density compared with placebo in individuals with AATD-associated emphysema. The use of intravenous augmentation in patients with FEV 1 less than 30% is debated. , Recent guidelines recommend the use of augmentation therapy in this population. Current smokers have not been shown to benefit from intravenous augmentation therapy and should not be offered treatment until they achieve smoking cessation. Intravenous augmentation therapy also is not recommended for Pi∗MZ patients or other heterozygote genotypes with a normal M gene. Current guidelines recommend against LVRS in AATD patients on the basis of prior studies showing short-lived benefits compared with AATD-replete COPD patients and basilar predominance of emphysema in AATD, making LRVS more technically challenging. The National Emphysema Treatment Trial (NETT) also found higher mortality among AATD patients treated with LVRS compared with those treated with medical management alone. There currently is no specific treatment of AATD-associated liver disease.
Lung volume reduction surgery
History
Lung volume reduction surgery (LVRS) can trace it roots to the 1950s in Baltimore, Maryland. Dr Otto Brantigan ( Fig. 5 ) and colleagues described the surgical techniques and respiratory pathophysiology that form the basis for modern day LVRS surgery. Brantigan reasoned that in patients with “idiopathic hypertrophic obstructive pulmonary emphysema,” the removal of the periphery of the upper lobes would reduce lung hyperinflation, restore lung “elasticity” and the circumferential pull that tethers open the bronchioles ( Fig. 6 ), improve diaphragmatic mechanics and possibly improve cardiac function. Brantigan and colleagues performed staged bilateral thoracotomies utilizing a clamp and suture technique to remove the periphery of the upper lobes. Brantigan and colleagues reported a series of 31 patients who underwent LVRS. There were 6 postoperative deaths (19%) but no deaths after the second surgery. They reported all but 1 patient who survived the first surgery “benefited.” Pulmonary function tests (PFTs) results were not reported but they noted that some patients had no improvement in measure pulmonary function after surgery but breath sounds and exercise capacity was “greatly improved.” The operation subsequently was abandoned due to high operative mortality and inconsistent results.


In the 1990s, LVRS was resurrected by Joel Cooper and colleagues. They performed bilateral LVRS via a median sternotomy and removed 20% to 30% of the upper lobes using an endostapler with reinforced bovine pericardium. Their case series showed benefit both in terms of PFT values and exercise capacity. Cooper and colleagues reported results from 150 patients who had undergone bilateral LVRS via median sternotomy. Six months after surgery, the average improvement in PFT values were impressive: 51% increase in FEV 1 , 14% decrease in total lung capacity (TLC), and 28% decrease in residual volume (RV). The average improvement in 6-minute walk distance (6MWD) after 6 months was 19%, and the percent of patients requiring supplemental oxygen with exercise went from 95% prior to surgery to 43% 6 months after surgery. Likewise, 6 months after LVRS, 98% of patients rated their health as somewhat or much better on the 36-Item Short Form Health Survey questionnaire (SF-36). The perioperative mortality rate was 4%.
Cooper and colleagues’ results garnered substantial enthusiasm and set off a resurgence of interest in LVRS. LVRS was widely adopted with numerous technical modifications. This fervor for LVRS waned in response concerns for patient safety and surging health care costs. , The data supporting LVRS were based on nonrandomized case series with short-term follow-up, inconsistent selection criteria, and no agreement on what defined patient benefit. ,
The National Emphysema Treatment Trial
The NETT started enrolling patients in January of 1998. Prior to completing the study, they reported that patients with an FEV 1 less than 20% of predicted and either homogeneous emphysema or a diffusing capacity for carbon monoxide (DLCO); less than 20% of predicted had high 30-day postoperative mortality (16% in the LVRS group vs 0 for the medical group) and they were unlikely to have functional improvement. The NETT was completed in July 2002. It enrolled 1218 patients (608 LVRS group and 610 medical treatment group) with advanced emphysema (FEV 1 <45% predicted, TLC >100% predicted, and RV >150% predicted) from 17 US medical centers. The NETT compared LVRS plus PR to optimal medical therapy plus PR. The primary endpoints were mortality and maximum exercise capacity at 24 months. Overall, LVRS was found to improve exercise capacity but have no effect on mortality (0.11 deaths per person-year in both groups). Exercise capacity at 24 months improved by greater than 10 W in 15% of the LVRS group versus 3% of patients in the optimal medical therapy group. Post hoc analysis identified 4 groups based on distribution of emphysema (upper lobe predominant versus non–upper lobe predominant) and exercise capacity (high exercise capacity low exercise capacity). In patients with predominantly upper lobe emphysema and low baseline exercise capacity, LVRS yielded a survival advantage over the medical therapy group (50% reduction in mortality). In the predominantly non–upper lobe emphysema and high baseline exercise capacity group, mortality in the LVRS group was twice as high as the medical therapy group, and there was no improvement in exercise capacity. In the predominantly upper lobe emphysema and high baseline exercise capacity group and the predominantly non–upper lobe emphysema and low baseline exercise capacity group, there was negligible improvement in exercise capacity but no improvement in survival. A subsequent meta-analysis of LVRS also found that patients with advanced heterogeneous emphysema and low exercise tolerance based on 6MWD experienced better functional outcomes from LVRS than from medical therapy.
Surgical Approaches
Varying surgical techniques are used for LVRS but most commonly median sternotomy, thoracotomy, or bilateral video-assisted thoracoscopic surgery (VATS) are employed. In the NETT data set, there was no significant difference in mortality, morbidity, or functional improvement between thoracotomy and median sternotomy. The VATS approach has been reported to decrease hospital cost and length of stay. The LVRS procedure, regardless of the initial incision, requires the resection of 20% to 30% of each lung using an endostapler with a continuous staple line reinforced with bovine pericardial strips to mitigate the development of air leaks ( Fig. 7 ). Emphysematous lung is left behind to avoid creating mismatch between the size of the lung and volume of thoracic cavity. In general, patients are extubated within 1 hour of completing the procedure. Chest tubes are placed to water seal in attempt to minimize transpulmonary pressure and reduce air leaks. Patients commonly require aggressive postoperative bronchial hygiene. The most common postoperative complications are prolonged air leaks and pneumonia. ,

Endobronchial valves are discussed elsewhere in this issue. In general, 3 to 5 one-way valves are placed in the bronchi of the most severely affected lobes to decompress the over inflated emphysematous lung and direct the air to less affected regions of the lung ( Fig. 8 ). In individuals with severe AATD, the values typically are placed in the lower lobe bronchi. When endobronchial valves are successful, the patient experiences the physiologic benefits of LVRS without having to undergo major thoracic surgery.

Physiology of Lung Volume Reduction Surgery
The mechanisms that result in the physiologic improvement following LVRS are not precisely known. Reducing the size mismatch between the hyperinflated, floppy emphysematous lungs and the overdistended chest cavity is believed to play a central role in the physiologic improvement following successful LVRS. Restoring the size mismatch between the lungs and chest wall should improve the elastic recoil of the lung and thereby help to tether open the small and medium-sized airways, which would prevent premature airway closure and improve expiratory airflow. , It also may reduce exercise-induced dynamic hyperinflation and thereby decrease exertional breathlessness. Sciurba and colleagues reported that improved lung elastic recoil after LVRS was associated with increased exercise capacity. Decreasing lung and chest wall hyperinflation would help to restore the curvature of the diaphragm and place it on a more advantageous part of its length tension curve. The effect should be similar for the intercostal and scalene muscles. Overall, there would be improved synchrony between the diaphragm, the intercostal muscles, the scalene muscles, and the other inspiratory muscles, resulting in amelioration of the chronic fatigue seen in the respiratory muscles of individuals with severe emphysema. , Relieving lung hyperinflation also may benefit cardiac performance by allowing improved left ventricular filling decreased left ventricular end-diastolic pressure. The physiologic benefit of endobronchial valves is believed to be similar to LVRS.
Lung Reduction Surgery in Alpha 1 -antitrypsin Disease
There are 5 published series of severe AATD patients who underwent LVRS and 3 published series of severe AATD patients who underwent endoscopic lung volume reduction (ELVR). Table 1 compares baseline characteristics among the 8 published series. The studies ranged from n = 6 to n = 20, for a total of 107 patients; 76 patients underwent LVRS and 41 underwent ELVR via endobronchial valve. A majority of patients studied were men, ranging from 47% to 83% in the 5 studies that broke down participants by sex. The average age ranged between 48 years and 65 years. In the 4 series that reported the prevalence of prior cigarette smoking, 72% of patients were former cigarette smokers. All of the 64 patients with reported AATD levels had severe deficiency (<12 mmol/L or <65 mg/dL) and 96% (69/72) had the Pi∗ZZ allele. Nearly three-quarters (70/95) of patients had the classic AATD distribution of lower lobe predominant emphysema in the 7 series that reported distribution.
Series | N | Women (%) | Age (y), mean ±SD | Prior Cigarette Smoker (%) | Alpha 1 -antitrypsin <12 μmol/L (%) | Pi∗ZZ (%) | Augmentation Therapy (%) | Emphysema Distribution Lower Lobe Predominance |
---|---|---|---|---|---|---|---|---|
Cassina et al, 1998 | 12 | 5 (42) | 49 ± 10 | 5 (42) | 12 (100) | 12 (100) | 12 (100) | ND |
Gelb et al, 1999 | 6 | 1 (17) | 61 ± 9 | 4 (67) | 6 (100) | 6 (100) | 5 (50) | 6 (100) |
Tutic et al, 2004 50 | 21 | 10 (48) | 56 ± 2 | 19 (90.5) | 21 (100) | 18 (86) | 1 (5) | 10 (48) |
Dauriat et al, 2006 | 17 | ND | 56 ± 9 | ND | 15 (ND in 2 patients) | 15 (ND in 2 patients) | ND | 17 (100) |
Stoller et al, 2007 | 10 | 2 (20) | 65.8 (55.4– 77.0) a | ND | 10 (100) | ND | ND | 3 (30) |
Tuohy et al, 2011 | 6 | 1 (17) | 48 | ND | 6 (100) | 6 (100) | ND | 6 (67) |
Hillerdal et al, 2014 | 15 | 8 (53) | 64 | 11 (73) | ND | 15 (100) | 0 (0) | 13 (87) |
Delage et al, 2019 | 20 | ND | ND | ND | ND | ND | ND | 17 (85) |
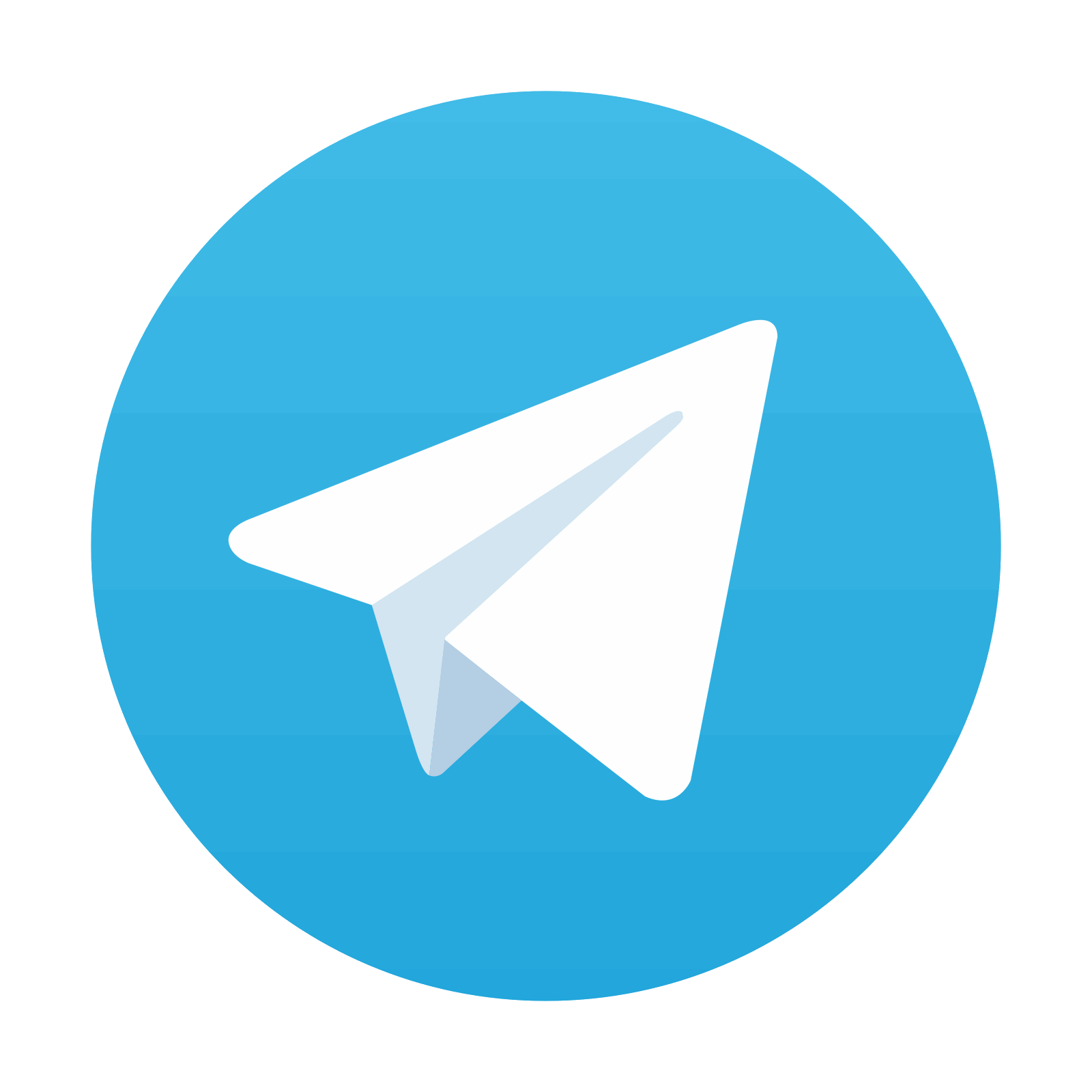
Stay updated, free articles. Join our Telegram channel

Full access? Get Clinical Tree
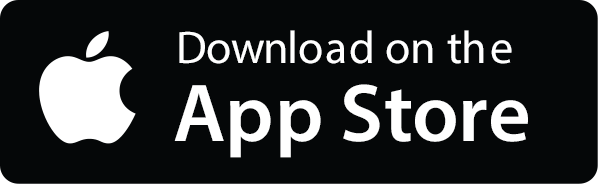
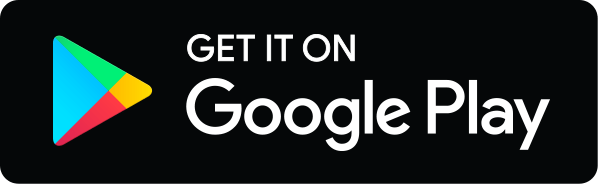
