Airway Management
INTRODUCTION
The Priority of the Airway
In the crucial initial management of the injured patient, securing the airway is quite literally the single most important priority; failure to oxygenate and ventilate represents the difference between life and death as well as functionality and disability. Airway loss initiates a terminal and irreversible cascade of events. Not only are hypoxia and hypoventilation common injury-related causes of mortality, but also they additionally represent one of the most common causes of preventable mortality following injury. The trauma patient represents a unique and exquisite airway challenge, from both anatomic and physiologic perspectives. The multisystem trauma patient represents the culmination of interrelated insults to oxygenation and ventilation, where under extreme duress, clinicians must rapidly recognize compounding injury and prioritize the airway in what may be multiple conflicting priorities.
BASIC AIRWAY EQUIPMENT AND PREPARATION
Preparation for Airway Management
Successful airway management starts with planning and preparation. Planning begins by assembling an airway kit or cart containing the necessary equipment for intubation. The practitioner should take the time to inventory the equipment prior to the intubation, ensuring function and availability.
Equipment
The airway cart should consist of drugs, endotracheal tubes, airways, laryngoscopes, airway adjuncts, a variety of syringes and needles, and equipment to establish a surgical airway.
The primary components of the airway kit are the endotracheal tube and laryngoscope. The airway cart should have a variety of tubes in both cuffed and uncuffed types, including tubes down to a 2.0 internal diameter size for pediatrics, and a variety of stylet sizes. Both Miller and Macintosh laryngoscope blades should be included. In addition, the airway cart should be stocked with both the oropharyngeal airway (OPA) and nasopharyngeal airway (NPA) in all sizes. In the event of failed intubation, several airway adjuncts should be included in the standard airway cart. An array of adjuncts is now available to assist with difficult intubation and failed airway, including supralaryngeal airways, lighted wand stylets, retrograde intubation kits, a variety of video laryngoscopic devices, and the gum elastic bougie (GEB).
The goal of airway management in the trauma admitting area is to closely simulate the control of the operating room. Key personnel should work as a team to assure a successful intubation (Fig. 11-1). The optimal intubating team should consist of at least three members including the intubator, a respiratory therapist, and an assistant to maintain cervical spine alignment or to provide the Sellick maneuver to prevent aspiration.
FIGURE 11-1 The optimal rapid sequence intubation team is comprised of (1) the intubating provider at the head of the bed, (2) an assistant to the patient’s right to facilitate passage of necessary equipment from the airway cart (to the intubating provider’s right) and to provide cricoid cartilage pressure, (3) a provider to administer drugs to the patient’s left, and (4) a respiratory therapist to assist with airway maneuvers. Note the presence of full barrier precautions, pulse oximetry, telemetry, colorimetry, and two functioning large-bore intravenous catheters.
Monitoring and Troubleshooting
Pulse Oximetry. The pulse oximeter is a portable, noninvasive, and reliable device that measures SpO2. Although very accurate in analysis of peripheral oxygen delivery, the pulse oximeter may display inaccurate readings in the case of carbon monoxide poisoning, high-intensity lighting, hemoglobin abnormalities, and a pulseless extremity or in severe anemia.
Capnography. End-tidal carbon dioxide (ETCO2) detectors measure the partial pressure of carbon dioxide in a sample gas. The patient’s Paco2 is typically 2–5 mm Hg higher than the ETCO2 and a normal reading in a trauma patient is approximately 30–40 mm Hg. ETCO2 reading may be used to confirm placement of an endotracheal tube. The presence of carbon dioxide in the exhaled air strongly suggests correct placement of the endotracheal tube in the trachea in a perfusing patient. The disposable capnometer indicates the presence of carbon dioxide with a color change. The electronic capnometer provides the health care provider with a numerical ETCO2 and plots the CO2 concentration against time. Although the use of capnometry as an adjunct to monitor the patient’s exhaled carbon dioxide has met some success, conditions such as hypotension, increased intrathoracic pressure, and pulmonary embolus resulting in an increased dead space ventilation may decrease the accuracy of the capnometer.
Troubleshooting. The intubating team should have the patient attached to a cardiac monitor, blood pressure cuff, and pulse oximeter (Fig. 11-1). Intravenous access should be established. The patient should receive supplemental oxygen via a nonrebreather mask or a bag valve mask (BVM) depending on the patient’s inherent respiratory drive. The correct size of endotracheal tube should be brought to the bedside and a correctly sized stylet should be inserted. The endotracheal balloon should be checked for leaks. Once the balloon has been checked, a 10-cm3 syringe is left attached to the pilot balloon. A suction device with a large catheter tip should be readily available and be placed near the right side of the patient’s head. The laryngoscope handle/blade connection should be checked for a functional light source. A means to secure the endotracheal tube should be available. Dentures should be removed just before intubation, particularly if the patient is being bagged and his or her dentures allow for a tight mask seal. If cervical spine injury has been excluded, the patient should be positioned with the neck slightly flexed and the head slightly extended on an imaginary axis through the patient’s ears. Placing a pillow or towel under the patient’s occipital region and elevating the head approximately 10 cm may facilitate this position. Due diligence with respect to preparation of both personnel and equipment makes for a less stressful intubation and improves the practitioner’s chances of successfully intubating the patient.1
Manual Airway Maneuvers
Before any airway maneuver is undertaken, a quick visual inspection of the oropharyngeal cavity should be done. Any foreign or loose material should be swept clear with a gloved finger or removed with suction. Blood may be present in the mouth of a trauma patient and adequate suctioning is essential to maintaining an open airway. Administration of oxygen prior to suctioning may prevent hypoxemia due to prolonged suctioning. The tongue can cause airway obstruction in the unresponsive patient as it often lacks tone and falls into the oropharynx. Manual airway maneuvers serve to elevate the tongue out of the hypopharynx.
Jaw Thrust. The cervical spine should be kept in normal alignment. The provider should grasp the sides of the patient’s face with fingers 3-5 along the ramus portion of the mandible. The provider’s thumb is on the patient’s cheek and the index finger on the chin and lower lip. These two fingers can open the patient’s lips or serve to seal the mask on a BVM. The provider’s fingers should form an “E” with the three lower fingers and a “C” with the thumb and index finger. Force is applied to the angle of the mandible forcing the mandible forward and anteriorly, while simultaneously opening the mouth with the index finger on the chin.
Chin Lift. With either a free, gloved hand or another provider’s gloved hand, the provider’s thumb is placed into the patient’s mouth, the patient’s lower incisors and chin are grasped, and the patient’s mandible is lifted anteriorly. This maneuver supplements the jaw thrust and works to lift the mandible anteriorly, elevating the tongue out of the oropharynx.
Sellick Maneuver. The injured patient may have swallowed large amounts of air, or air has been forced into the stomach during BVM ventilation. The use of the Sellick maneuver, particularly during BVM ventilation, aids in preventing aspiration. The maneuver is accomplished by applying gentle posterior pressure with the thumb and index finger to the patient’s cricoid cartilage. This pressure causes the cricoid cartilage to be displaced posteriorly, effectively closing off the esophagus.
Basic Mechanical Airway Devices
Oropharyngeal Airway
Oropharyngeal and nasopharyngeal devices can be inserted into either the mouth or nose of the patient, serving to elevate the tongue out of the oropharynx. The OPA is a curved, plastic or hard rubber device, which comes in various sizes and has channeling for suction catheters. The device is sized by placing the OPA in the space between the patient’s ear and corner of the mouth. A correctly sized OPA will extend from the patient’s mouth to the angle of the jaw.
Indications for the use of the OPA include a patient who is unable to maintain his or her airway or to prevent an intubated patient from biting the endotracheal tube. Advantages for use of the OPA include the following:
1. Prevention of obstruction by the patient’s teeth and lips
2. Maintenance of the airway in a spontaneously breathing unconscious patient
3. Ease of suctioning
4. Use as a bite block in a patient who is having a seizure
The OPA is contraindicated in a conscious patient as it may stimulate a gag reflex. In addition, it does not isolate the trachea, nor can it be inserted through clenched teeth. It may obstruct the airway if it is improperly placed and can be dislodged easily. To place the OPA, the mouth is opened and the OPA is inserted with the curve reversed and the tip pointing toward the roof of the patient’s mouth. Using a twisting motion the OPA is rotated into position behind the base of the patient’s tongue. Alternatively, a tongue blade can be used to depress the tongue with the OPA placed directly into the oropharynx.
Nasopharyngeal Airway
The NPA is a soft rubber or latex uncuffed tube that is designed to conform to the patient’s natural nasopharyngeal curvature. It is designed to lift the posterior tongue out of the oropharynx. Like the OPA, it is indicated for patients who cannot maintain their airway. The advantages of the NPA include ease and speed of insertion, patient tolerance and comfort, and effectiveness when the patient’s teeth are clenched. Disadvantages of the NPA include its smaller size, the risk of nasal bleeding during insertion, and lack of utility when a basilar skull fracture is suspected.2
The provider should first size the NPA by selecting an NPA that is slightly smaller than the patient’s nostril. The distance from the patient’s nose to earlobe determines the length. The NPA should be liberally lubricated with lidocaine gel prior to insertion. The right nare is preferentially chosen, as it is typically larger. Gentle pressure should be applied until the flange rests against the patient’s nostril. After a basic mechanical airway has been inserted, the patient should be oxygenated with supplemental oxygen or a BVM.
Bag Valve Mask
The BVM assists the provider with oxygenation and ventilation in the apneic or hypoventilating patient. With an effective mask seal and an open airway, the BVM can deliver tidal volumes approaching 1.5 L and nearly 100% inspired oxygen with an attached oxygen reservoir. The BVM consists of a bag with a volume of 1.6 L and a standard face mask attached via a oneway, nonrebreathing valve. A reservoir bag and oxygen source is attached to the opposite end of the bag. Multiple sizes are available to treat neonatal, infant, children, and adult patients. To effectively use the BVM, a second provider may be required to establish a properly fitted mask seal while the second provider squeezes the bag. Maintenance of oxygenation and ventilation can be improved by utilizing a basic mechanical airway and proper jaw thrust and chin lift techniques while “bagging” the patient.
ASSESSMENT OF THE AIRWAY
A single indicator has not been identified that accurately predicts the inability to intubate. However, an attempt at airway assessment is mandatory to assist with the prediction of difficulty to intubate or ventilate.3
History
Time permitted, a history should be quickly obtained. The patient should be interrogated as to prosthetic dental devices, any previous difficulty with anesthesia or intubation, sleep apnea, trismus, and the timing of his or her last meal.
Physical Examination
A general assessment of the oropharynx, nose, maxilla, mandible, and neck should be rapidly undertaken. An assessment of the patient’s overall level of consciousness (e.g., Glasgow Coma Score) and the ability of the patient to open his or her mouth should also be performed. If possible, have the patient stick out his or her tongue to evaluate the size of the tongue. The vocal quality of the patient should be noted during the physical exam, as well. The presence of stridor usually indicates some degree of injury to the upper airway. Assess for dentures or other prosthetic devices that may need to be removed prior to intubation.
INTUBATION OF THE TRACHEA
The majority of injured patients are able to undergo successful orotracheal intubation (OTI). In compliance with the ATLS course, the preferred definitive airway is tracheal intubation through the mouth using direct laryngosocopy.1
Guidelines for Emergency Tracheal Intubation
Indications for intubation relate to the following three simple questions:
• First, is the patient able to oxygenate and ventilate?
• Second, is the patient able to maintain an airway?
• Third, will the underlying injury and physiology of the patient lead to a failure to maintain the airway, oxygenate or ventilate?
The practice management guidelines of the Eastern Association for the Surgery of Trauma (EAST) for tracheal intubation secondary to trauma reveal no Level I data (evidence from randomized, controlled trials) that predict the need for urgent intubation.4 The guideline calls for emergency tracheal intubation in trauma patients exhibiting the following characteristics:
• Acute airway obstruction
• Hypoventilation
• Severe hypoxemia despite supplemental oxygen
• Severe cognitive impairment (Glasgow Coma Scale score ≤8)
• Cardiac arrest
• Severe hemorrhagic shock
In concordance with ATLS, the EAST guidelines promulgate the concept that OTI utilizing direct laryngoscopy is the procedure of choice for airway control following trauma.4
Anatomy and Technique of Direct Laryngoscopy and Intubation
Success in intubating the injured patient is dependent on thorough knowledge of the anatomy of the upper airway and a meticulous adherence to proper technique. The vocal cords lie posterior and inferior to the pliable epiglottis, which should be visualized as a constant reference point during laryngoscopy. The posterior-most esophagus may be lifted into view with sufficient elevation of the epiglottis. Since the majority of intubations occur prior to cervical spine clearance, head and neck immobilization is of highest priority. In the rare instance that the cervical spine is cleared, the patient should be placed in the sniffing position initially (extension of the head, slight flexion of the lower cervical spine), which optimizes the alignment of the oral, pharyngeal, and laryngeal axes.
Following preparation of equipment and personnel, the laryngoscope is grasped firmly with the left hand. It should be emphasized that the right hand should be kept free, for suctioning, manipulation of oral structures, and placement of the endotracheal tube. Selection of a straight versus curved blade has less to do with proven efficacy in a particular scenario than the comfort and proficiency of the laryngoscopist with a particular blade. In general, the straight blade is utilized to pass beneath, and directly elevate, the epiglottis. The straight blade is inserted into the esophagus, with the blade withdrawn slowly under direct visualization to expose the glottic opening. The same technique can be applied with a curved blade of sufficient size, although the curved blade technique typically utilizes insertion of the tip of the blade into the vallecula, with anterior traction of the epiglottis, exposing the glottic opening. The tongue should be displaced by the blade to the patient’s left for best visualization of the epiglottis.
The motion and direction of the laryngoscope in the left hand during laryngoscopy is of critical importance to safe and successful intubation of the trachea. The proper technique of laryngoscopy employs upward motion of the laryngoscope in the parallel plane of the handle. A “rocking” motion, during which the handle is rotated counterclockwise and posterior, should never be used. This posterior circular motion can impart dangerous extension on the cervical spine or fracture or dislodge teeth. In addition, the left elbow should not be placed on the bed or spine board for stabilization. As the blade is positioned to visualize the glottic opening, the right hand can be utilized to employ the BURP maneuver if the glottic opening is not readily visible. This technique includes Backward, Upward, Rightward, Pressure on the thyroid cartilage and is distinct from the Sellick maneuver.
RAPID SEQUENCE INTUBATION
Overview
Intubation by rapid sequence induction (RSI) has become the gold standard for management of the airway in trauma and critical illness. The technique of RSI has been demonstrated to increase intubation success rates and reduce complications compared to pre-RSI techniques in a variety of emergent settings.5–9 RSI benefits include provision of optimal intubating conditions for injured patients, rapid airway control, high success rates, and reduction of pulmonary aspiration. The adaptability of RSI to individual patient conditions renders the technique optimal for airway control in the injured.
First developed to facilitate operating room intubations in patients with full stomachs, thereby minimizing risk of aspiration,10 the technique is now widely utilized by prehospital paramedics,11,12 emergency medicine physicians,13 and trauma surgeons, with a high reported intubation success rate by nonanesthesiologists.5,14 Approximately 80% of intubations performed in North American emergency departments utilize RSI, with a 90% success rate by the first intubator.14 In the technique of RSI, laryngoscopy and intubation are facilitated by use of sedating induction agents and short-acting neuromuscular blockade (NMB). Rapid sequence intubation (RSI) can be divided into five phases: (1) patient and equipment preparation, (2) preoxygenation, (3) premedication, (4) paralysis, and (5) placement of the tube. A time line for RSI is demonstrated in Table 11-1.
TABLE 11-1 How We Do It: Steps and Timing of Rapid Sequence Intubation in a 70-kg Adult
Preparation
Although significant injury with physiologic instability may preclude prolonged preparation for RSI, all efforts should be made to allow for individualized assessment of comorbid conditions, airway status, predictors of difficult intubation, and anticipated pharmacologic regimen. The selection and sequence of pharmacologic agents should be determined, with all agents ready and available in clearly labeled syringes. A horizontal approach to the preparatory phase of RSI is optimal, during which multiple personnel with predetermined responsibilities and positions work simultaneously (Fig. 11-1). In this manner, the preoxygenation phase is initiated during preparation
Preoxygenation
Although not originally considered an essential component of RSI, preoxygenation is now considered optimal if the oxygenation, ventilation, and hemodynamic status of the patient permit. The purpose of preoxygenation is to replace the nitrogen-dominant room air occupying the pulmonary functional residual capacity with a 100% oxygen reservoir, such that saturation of arterial hemoglobin (SaO2) is prolonged. Preoxygenation can be accomplished by gently assisting spontaneous respirations with 100% oxygen or simply allowing the patient to breathe 100% oxygen. Forceful BVM ventilation should be assiduously avoided during the intubation sequence, as it may produce unnecessary gastric distention, increasing the risk for pulmonary aspiration of gastric contents. Recommendations for the duration of preoxygenation necessary for optimal hemoglobin saturation range from 3 to 5 minutes; however, the effectiveness of preoxygenation is dependent on the physiologic status of the patient as well as age, size, and comorbid conditions. For example, an optimally preoxygenated healthy 70-kg adult will maintain SaO2 over 90% for approximately 8 minutes, an obese adult less than 3 minutes, and a 10-kg child less than 4 minutes.15 More importantly, the desaturation from 90% SaO2 to 0% occurs much more rapidly than the fall from 100% to 90%. The approximate Pao2 at 90% SaO2 is 60 mm Hg, falling to 27 mm Hg at an SaO2 of 50%. An injured patient with little compensatory reserve can decline from 90% to 0% literally in seconds.
PHARMACOLOGY OF RAPID SEQUENCE INTUBATION
It is imperative that providers caring for the injured be facile with all pharmacologic agents utilized for RSI, including both barbiturate and nonbarbiturate hypnotics, neuromuscular blocking agents, benzodiazepines, dissociative agents, and opiates (Table 11-2). A “one method fits all” approach is not always applicable,16 and each patient should be individualized based on type and mechanism of injury, comorbidities, and potential for adverse events. However, the majority of trauma patients can be effectively intubated using a generalized pharmacologic regimen.
TABLE 11-2 Pharmacology of Rapid Sequence Intubation
Clinical predictors of need for sedation and paralysis have been identified. In data from a prospective study of endotracheal intubation, factors associated with drug-facilitated intubation, defined as intubation facilitated by the use of sedatives or paralytics, included clenched jaw or trismus, declining verbal Glasgow Coma Scale score, and use of cervical spine precautions.17 The vast majority of patients currently undergoing RSI received both sedative and paralytic agents. Evidence-based principles identified by the EAST airway workgroup for drugs administered during OTI include the following:4
• Neuromuscular paralysis
• Sedation
• Regimen that maintains hemodynamic stability
• Regimen that minimizes intracranial hypertension
• Regimen that prevents vomiting
• Regimen that prevents intraocular content extrusion
Premedication Agents
Airway stimulation, including laryngoscopy and endotracheal tube placement, results in the pressor response, an intense autonomic sympathetic discharge producing tachycardia, hypertension, and increased intracranial pressure.10,18 The degree of airway stimulation is proportional to the magnitude of the pressor response. Correspondingly, increased intragastric, intrathoracic, and intracranial pressures may result from Valsalva, bronchospasm, or coughing. As the foundation of RSI relates to abrogating the untoward effects of airway stimulation, preinduction agents are routinely utilized to blunt the physiologic response to laryngoscopy and endotracheal tube placement. A common mnemonic for the preinduction regimen is LOAD, which includes Lidocaine, Opiates, Atropine, and a Defasciculating agent, although atropine is typically utilized in pediatric populations only.
Opioids. Depth of sedation may correlate with speed of intubation in RSI.19 The sedative and analgesic effects of opioids may provide benefit to the injured patient prior to induction. A commonly used opioid for RSI in the prehospital and emergency department setting is fentanyl, which at 5.0 mcg/kg has been shown to be hemodynamically neutral compared to midazolam and thiopental during RSI.19 Fentanyl effectively blunts airway reactivity,20 and confers the significant added benefit of analgesia in the injured patient.
Benzodiazepines. Benzodiazepines, a family of gamma aminobutyric acid (GABA) agonists, have been utilized in RSI for sedation. Midazolam is the most widely studied agent, having favorable pharmacokinetics for RSI, including rapid onset and short half-life. Advantages include hemodynamic neutrality and retrograde amnesia, although onset is slower than comparable agents and the intubation reflex is not attenuated,19 rendering it less commonly used in RSI protocols than opioids.
Antiarrhythmics. Despite a great deal of controversy regarding the potential benefits of lidocaine during RSI, it is a preinduction agent common to RSI protocols,21 and is advocated in many emergency airway courses. Lidocaine has a number of theoretical beneficial preintubation effects, including abrogation of airway reactivity following placement of the endotracheal tube, the tachycardic response to intubation, and succinylcholine-induced myalgia and fasciculations.22 The two primary potential benefits of lidocaine use in RSI are to mitigate against reflex bronchospasm and increased intracranial pressure. Although definitive data are lacking regarding effects on intracranial pressure, the potential benefits outweigh the negligible side effects of the RSI dose.
Beta-Adrenergic Blockers. Esmolol has been utilized as a preinduction agent for mitigation of the tachycardic component of the pressor response. In comparison to lidocaine and fentanyl, esmolol has demonstrated superiority in attenuating the pressor response.23 Beneficial pharmacokinetics include rapid onset and short action; however, the bradycardic and negative inotropic effects of esmolol may blunt the compensatory response to hemorrhage. The cardiac effects may produce corresponding reductions in cerebral blood flow. Therefore, esmolol should be considered only in controlled circumstances in which hemorrhage of brain injury have been definitively excluded. The availability of other safer agents that effectively minimize the pressor response relegates beta-blockers to clear second-line status.
Defasciculation Paralytic Agents. Succinylcholine, the standard neuromuscular blocking agent utilized for RSI, produces significant myoclonal fasciculations, prompting a rise in intracranial pressure in patients at risk for intracranial hypertension. Therefore, a defasciculating dose of a competitive neuromuscular blocking is administered during RSI. Common defasciculating agents include vecuronium and rocuronium, and, less commonly, pancuronium. Defasciculating doses are administered as 10% of the paralyzing dose, given 3–5 minutes prior to administration of succinylcholine. It is unusual for defasciculating doses of neuromuscular blockers to cause apnea. However, patient weight is commonly an estimate during RSI following injury, and preparations to assist with ventilation should be made.
Induction Agents
The perfect induction agent would possess rapid onset and elimination, render the patient unconscious but also amnestic, possess analgesic properties, and have negligible side effects. In injured and critically ill patients, the ideal agent would produce little cardiovascular effects and maintain cerebral perfusion pressure. Regrettably, such an agent does not yet exist. Because many agents produce side effects, including myocardial depression with the potential for hypoperfusion, careful attention should be dedicated to the selection of individual agents.
Etomidate. Etomidate is a short-acting carboxylated imidazole hypnotic agent frequently utilized for rapid sequence induction. It possesses ideal characteristics for urgent and emergent RSI in trauma patients, including rapid onset and clearance, reduction in cerebral metabolic rate,24 and negligible effects on hemodynamics. This favorable pharmacokinetic profile has led to the widespread use of etomidate for RSI in head injury and hemodynamically labile patients. Accordingly, the American College of Surgeons Committee on Trauma added etomidate to the Advanced Trauma Life Support course as an induction agent for hypotensive trauma patients.1 The most significant side effect of etomidate relates to adrenal insufficiency, as it produces a reversible blockade of adrenal 11-beta-hydroxylase. In patients at risk for adrenal insufficiency, including head-injured, mechanically ventilated, and septic populations, etomidate has been independently correlated with reductions in serum cortisol.25,26 The controversial question relates to whether transient adrenal suppression produces lasting effects on outcome. In a recent large single-center study, a single induction dose of etomidate was independently associated with ARDS and multiple organ dysfunction syndrome.27 Further studies are warranted to determine the long-term safety of etomidate for RSI; however, given the multiple favorable characteristics of the drug, etomidate remains the standard induction agent in most RSI protocols.
Propofol. Propofol is a nonbarbiturate hypnotic agent that rapidly induces deep sedation and significant relaxation of laryngeal musculature.10 When used for induction, propofol produces intubation conditions equal to thiopental and equal to or superior to etomidate.28 Propofol should be used with caution in head-injured or hemodynamically labile patients due to a consistent hypotensive effect and potential reduction of cerebral blood flow.
Barbiturates. Thiopental is the most commonly used barbiturate for RSI. Like other induction agents, thiopental has rapid onset and clearance. In the aeromedical setting, thiopental has been shown equally efficacious to etomidate as an adjunct to RSI.29 Similarly, in an evaluation of 2,380 RSI procedures, patients were more likely to be successfully intubated using thiopental or propofol as compared to etomidate or a benzodiazepine.28 Thiopental reduces cerebral oxygen consumption and exhibits anticonvulsant effects, rendering it useful in closed head injury (CHI). However, the significant limitation of thiopental use in trauma relates to inhibition of central nervous system sympathetic response. Consequently, thiopental produces reduced myocardial contractility and systemic vascular resistance, inducing hypotension. Therefore, it is best reserved for patients who are euvolemic and normotensive, limiting its application in critically ill patients. Methohexital is an additional barbiturate that has been used for RSI. It exhibits similar effects to thiopental, although it is significantly more potent and has shorter onset and duration than thiopental.
Dissociative Agents. Ketamine, a rapid-onset dissociative sedative and anesthetic agent, is frequently used for RSI in the pediatric population and in adults with chronic obstructive pulmonary disease. In addition to its sedative effects, ketamine exhibits the beneficial properties of potent analgesia and a partial amnesia. As a sympathomimetic agent, ketamine may induce tachycardia and increased blood pressure. In addition, ketamine induces cerebral vasodilatation, potentially exacerbating intracranial hypertension. Trauma patients with documented or potential CHI should therefore not undergo induction for RSI with ketamine.
Paralysis
Neuromuscular Blocking Agents
Pharmacologic paralysis represents an integral component of RSI, facilitating emergent intubation for more than three decades.30 Paralysis of facial musculature facilitates visualization during laryngoscopy, confers total control of the patient, and reduces complications during intubation. Prehospital NMB has been demonstrated to be safe,8,9 and to improve intubation success in injured patients undergoing RSI.31,32 Potential adverse effects should be diligently assessed, particularly with use of succinylcholine. In addition, preparations for rescue techniques must be made prior to administration of a paralytic agent, in the event of intubation failure. Because paralytic agents provide no sedative, analgesic, or amnestic effect, it is imperative to combine paralytic use with an appropriate induction agent.
Succinylcholine. Succinylcholine, a depolarizing acetylcholine dimer, acts noncompetitively at the acetylcholine receptor in a biphasic manner to produce muscular paralysis at the motor end plate. Succinylcholine stimulates all muscarinic and nicotinic cholinergic receptors of both parasympathetic and sympathetic systems. Initial brief depolarization results in clinically notable muscular fasciculations, followed by sustained myocyte depolarization. Succinylcholine degradation is dependent on hydrolysis by pseudocholinesterase, and resistant to acetylcholinesterase. Due to rapid onset of action and short half-life, succinylcholine remains the gold standard for RSI in patients not at risk for adverse events. The standard dose of succinylcholine for RSI is 1.0 mg/kg, although recent data suggest a smaller dose of 0.5–0.6 mg/kg is sufficient for RSI,33 facilitating more rapid resumption of spontaneous respiration. Intramuscular injection of succinylcholine has been described, although the required dose, 4 mg/kg, is higher, and onset is slower, than intravenous injection.
A clear understanding of the potential adverse effects of succinylcholine is critical to its appropriate use in RSI. Contraindications are primarily related to conditions that accentuate the hyperkalemic effects of succinylcholine, as it normally produces a 0.5- to 1.0-mEq/L elevation of serum potassium. Contraindications related to hyperkalemia include thermal injury of age exceeding 24 hours, although receptor upregulation likely does not become clinically relevant until postburn day 5. Therefore, it is safe to use succinylcholine for RSI in most acute burns. Contraindications include crush injury or rhabdomyolysis with hyperkalemia, congenital or acquired myopathies, and conditions of subacute and chronic upper and motor neuron denervation including paralysis and polyneuropathy of critical illness.10 Contraindications related to known comorbid conditions include history of malignant hyperthermia and pseudocholinesterase deficiency. In addition, succinylcholine is reported to raise intragastric and intracranial pressure due to muscle fasciculations, and may contribute to increased intraocular pressure. It should be used with caution in head injury and penetrating globe injury, although the evidence that succinylcholine raises intraocular pressure is anecdotal at best.34
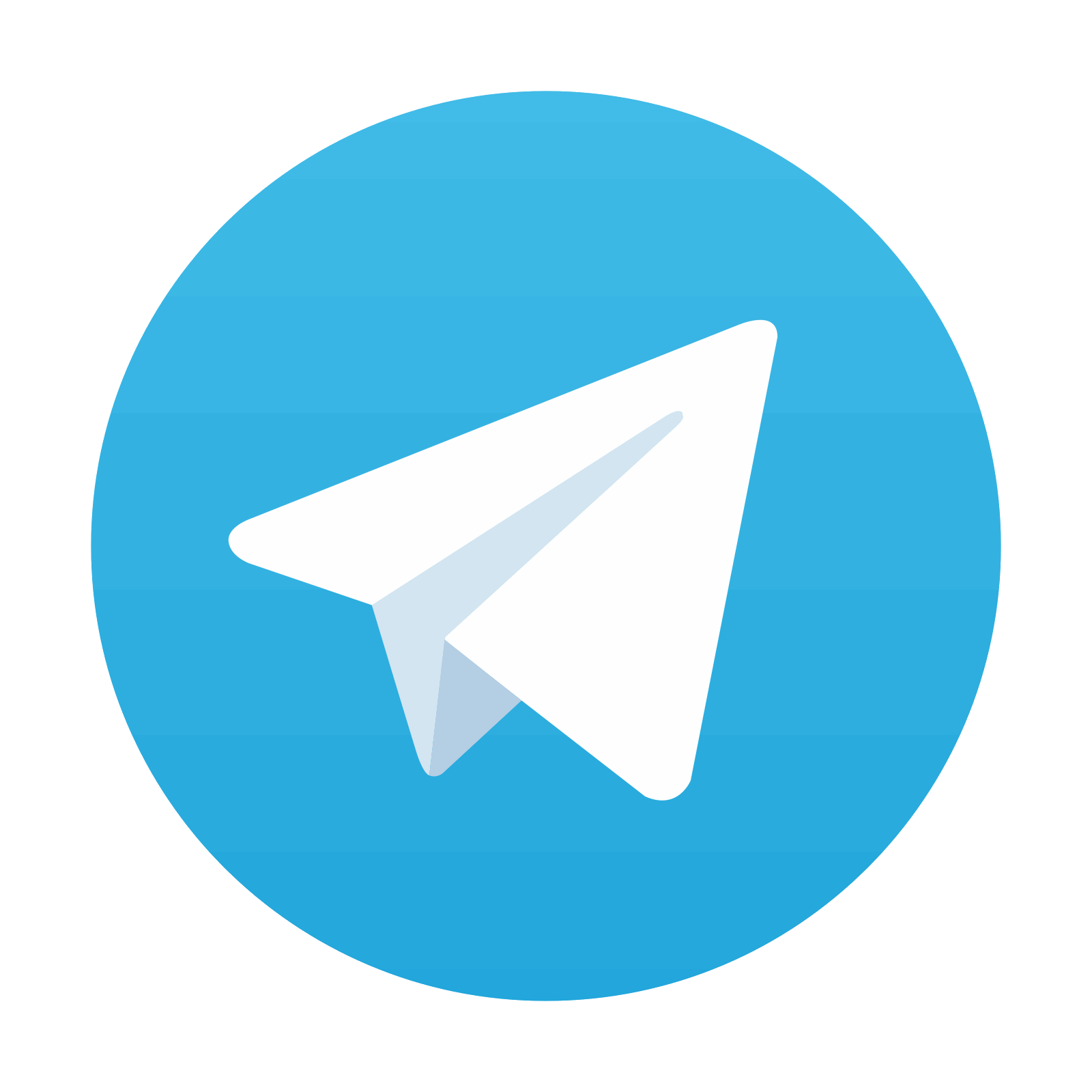
Stay updated, free articles. Join our Telegram channel

Full access? Get Clinical Tree
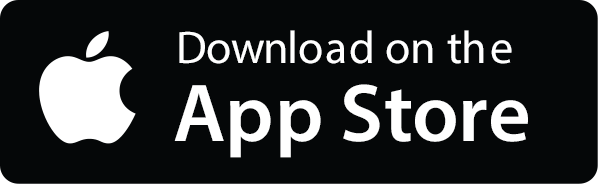
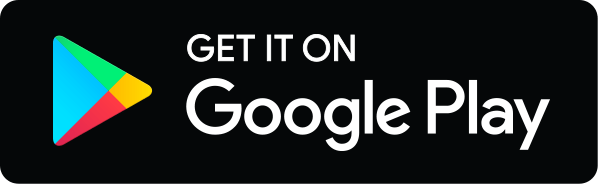