Chapter 80 Air Pollution
History
Air pollution has posed a threat to human health since the advent of fire, 500,000 years ago. Evidence of soot in prehistoric caves indicates that early humans were exposed to high levels of indoor air pollution. Outdoor pollution began to rise as populations grew and clustered in cities. During the Industrial Revolution, there was a dramatic increase in coal use by factories and households. The resulting smog caused significant morbidity and mortality, particularly when combined with stagnant atmospheric conditions. During the Great London Smog of 1952, heavy pollution for 5 days caused at least 4000 excess deaths (Figure 80-1). This episode highlighted the relationship between air pollution and human health and, together with other episodes, led to clean-air policies in Europe and in the United States. Over the next several decades, with improvements in the regulatory framework, air quality in the developed world steadily improved. Even as energy consumption and greenhouse gas emissions have increased, pollutants that are toxic to humans have been better controlled. Nevertheless, 186 million Americans, or 60% of the population, still live in counties with levels of air pollution higher than U.S. Environmental Protection Agency (EPA) standards (Figure 80-2). Air pollution also continues to be a growing problem in cities and households of the developing world.
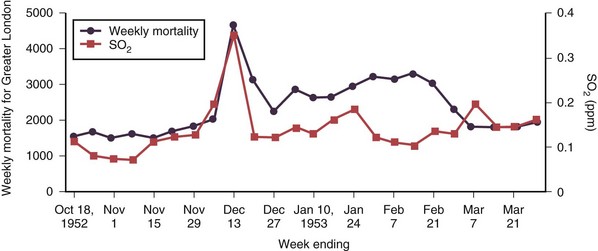
Figure 80-1 Weekly mortality in greater London, October 1952 to March 1953; SO2, oxygen saturation.
(Modified from Bell ML, Davis DL: Reassessment of the lethal London fog of 1952: novel indicators of acute and chronic consequences of acute exposure to air pollution, Environ Health Perspect 109(suppl 3):389–394, 2001.)
Composition of Air Pollution
Many different substances are considered “air pollutants,” which typically exist in combination in the environment; these include particulate matter (PM), carbon monoxide (CO), ozone (O3), nitrogen oxides (NOx), sulfur oxides (SOx), and volatile organic compounds (VOCs). The composition varies by season and regions, and the chemistry, state, and size of a pollutant influence the resulting health effects (Figure 80-3). Although CO has specific toxicity affecting oxygen transport, many pollutants appear to cause disease by triggering injury through inflammation and oxidative stress. Physiologic effects can be confirmed by experimental studies, but health impacts of air pollution on populations can be difficult to attribute to a single pollutant because they often share sources and vary together. A multipollutant approach to understanding health effects is becoming more common. Nevertheless, this section discusses some of the physiologic effects of individual pollutants before a description of the health outcomes seen with exposure to air pollution.
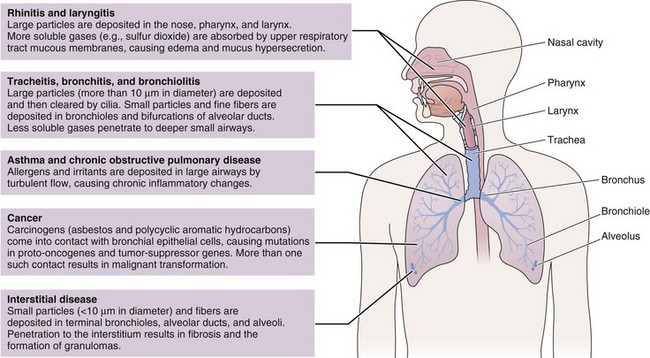
Figure 80-3 Physiologic effects of air pollutants on the respiratory tract.
(Modified from Beckett WS: Occupational respiratory diseases, N Engl J Med 342:406–413, 2000.)
Particulate Matter
Among air pollutants, PM is most consistently associated with increased mortality effects at ambient exposure concentrations regularly encountered today. Although PM can be formed by a wide variety of natural and man-made processes, one of the largest sources of PM pollution is through combustion of biomass and fossil fuels. PM is composed of organic and inorganic compounds that vary greatly in size (Figure 80-4). Fine PM (<2.5 µm) has the most well-recognized health effects, and the ultrafine PM fraction (<0.1 µm) may be especially important. Larger PM is filtered and trapped in the proximal respiratory tract, but fine particles can penetrate deep into the lung, where they are efficiently deposited, cause local effects, and may be systemically absorbed.
The composition of PM also influences its health effects. The mechanism of PM toxicity in the lung is still being elucidated but is likely multifactorial and can include impaired ciliary function, damage to epithelial cells, inflammation, and oxidative stress. Neutrophils and macrophages phagocytize the poorly soluble particles and initiate inflammatory cascades. PM also causes oxidative stress by generating reactive oxygen species (ROS) on the surface of particles that may then cause cellular effects. Inflammation and oxidative stress apparently provoke cardiovascular disease through atherosclerosis, thrombogenesis, vasoconstriction, and reduced heart rate variability (Figure 80-5).
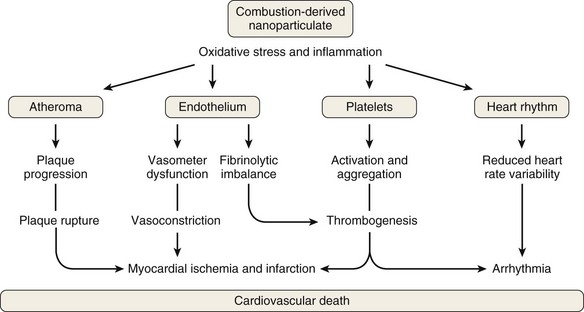
Figure 80-5 Mechanism of cardiovascular disease from exposure to air pollution.
(From Mills NL, Donaldson K, PW Hadoke, et al: Adverse cardiovascular effects of air pollution, Nat Clin Pract Cardiovasc Med 6:36–44, 2009.)