Summary
The right ventricle (RV) has to face major changes in loading conditions due to cardiovascular diseases and pulmonary vascular disorders. Clinical experience supports evidence that the RV better compensates for volume than for pressure overload, and for chronic than for acute changes. For a long time, right ventricular (RV) pathophysiology has been restricted to patterns extrapolated from left heart studies. However, the two ventricles are anatomically, haemodynamically and functionally distinct. RV metabolic properties may also result in a different behaviour in response to pathological conditions compared with the left ventricle. In this review, current knowledge of RV pathophysiology is reported in the setting of chronic pressure overload, including recent experimental findings and emerging concepts. After a time-varying compensated period with preserved cardiac output despite overload conditions, RV failure finally occurs, leading to death. The underlying mechanisms involved in the transition from compensatory hypertrophy to maladaptive remodelling are not completely understood.
Résumé
Le ventricule droit (VD) fait face à d’importantes variations de ses conditions de charge en réponse aux maladies cardiovasculaires et pathologies vasculaires pulmonaires. Le VD supporte mieux une surcharge de volume qu’une surcharge de pression, de même il s’adapte mieux aux variations progressives qu’aux changements aigus. La physiopathologie du VD a pendant longtemps été résumée à des extrapolations de la physiopathologie du ventricule gauche. Cependant les deux ventricules sont différents, tant sur le plan anatomique, hémodynamique que fonctionnel. Les caractéristiques métaboliques singulières du VD peuvent également être à l’origine d’une adaptation différente aux conditions pathologiques. Après une longue période compensatrice avec préservation du débit cardiaque, la dysfonction du VD s’installe finalement face à la surcharge chronique persistante. Les mécanismes impliqués dans la transition depuis l’hypertrophie compensatrice jusqu’au remodelage inadapté sont méconnus. Dans cette revue, les connaissances actuelles de la physiopathologie du VD dans l’hypertension pulmonaire chronique sont reportées à partir des résultats récents issus de la recherche expérimentale.
Background
Right heart failure is commonly related to left ventricular (LV) dysfunction. Ischaemic myocardial injury or, less frequently, arrhythmogenic dysplasia of the right ventricle (RV), congenital heart disease and chronic respiratory disease, including pulmonary vascular disorders, can also result in right ventricular failure (RVF). RVF is also a current problem in the post-operative course of heart transplantation and LV assist device implantation . In pulmonary hypertension (PH), pulmonary vascular damage will inevitably affect the whole cardiopulmonary unit . It has been appreciated over the last two decades that right ventricular (RV) dysfunction is the most important determinant of long-term outcomes in PH patients. Actually, prolonged survival is related more to RV function than to pulmonary haemodynamics per se. In studies addressing haemodynamic variables and survival in PH, high mean right atrial pressures and low cardiac output are consistently associated with poorer survival . Despite major improvements in pharmacological management over the last 15 years, PH patients still die from RVF.
RV pathophysiology has been overlooked for many years. In the past, several experiments relegated the RV to a passive conduit, suggesting that the RV pump might be neglected. For example, Starr et al. showed that electrocautery ablation of the RV free wall in dogs was not associated with significant changes in haemodynamics, and that all animals survived . For a long time, the Fontan procedure also supported the clinical evidence that RV absence did not compromise overall heart function . Physicians have recently shown clinical interest in the right heart, as RV function appears to be a prognostic factor in left heart failure, PH and other chronic respiratory disorders. Most of mechanisms involved in left heart diseases were first extrapolated to RV pathophysiology, despite the two ventricles differing in their embryology, geometry and physiology . Recently, a new focus on RVF has emerged, which aims to improve both understanding and clinical management. In PH, there are different phenotypic abnormalities in the RV. Surprisingly, some patients share the same phenotype, whereas the RV is not exposed to the same degree of pressure overload . Considering the central role of the RV in cardiopulmonary diseases, Mehra et al. suggested a comprehensive nomenclature of right heart failure, based on aetiology, anatomical injury, pathophysiology and functional status . Experimentally, major efforts have been made to improve our understanding of RV remodelling. Several animal models of chronic RVF have thus been reported over the last decade to reproduce the main features of RV dysfunction .
The mechanisms of RVF as well as the clinically relevant variables for measuring RV function in PH are still being debated. In this paper, after a brief description of the normal RV, we first seek to overview the functional evaluation of RV contractility and reserve in the setting of chronic pressure overload. We then present current knowledge of RV remodelling related to PH. Based on experimental findings, the cellular mechanisms and molecular pathways involved in the transition from compensated RV hypertrophy to dysfunction and failure are discussed. Finally, recent therapeutic approaches for the failing RV are considered. Isolated ex vivo studies of the RV will not be considered in this review.
The normal RV: embryology, morphology and physiology
The RV does not originate from the primary heart field, unlike the left ventricle (LV). Recent experiments have shown that the RV does not share the same origin as the LV, emphasizing the need for a specific comprehensive approach to each ventricle . The RV actually originates from the secondary heart field, while the atrium and the LV develop from the primary heart field. Three separate chambers can be described in the RV: the sinus or inlet, which consists of the tricuspid valve, chordae tendineae and papillary muscles; the trabeculated apical myocardium; and the infundibulum or conus . Compared with the LV, the RV has a crescent shape, with a higher capacity and a lower myocardial mass.
In utero the RV wall is thick because the pulmonary vascular resistance (PVR) is high. At birth, PVR decreases dramatically, leading to high compliance, resulting from progressive thinning of the RV free wall . RV contraction can be understood as a three-step motion. The tricuspid annulus first moves toward the apex, then the apex moves toward the infundibulum. The radial contraction of the RV free wall towards the interventricular septum is the third and minor component of RV contractility; it results from marked shrinking of the deep muscle fibres. This myofibre layer is continuous between the LV and the RV; it represents one of the three anatomical elements of ventricular interdependence, along with the pericardium and the interventricular septum, explaining why the LV is involved in RV contraction (from 20% to 40% of RV contractile performance) .
The RV is exposed to frequent changes in venous return, influenced by respiratory variations, by the supine or orthostatic position of the body and by pathological disorders affecting filling conditions. The RV is highly distensible because of its larger shape and four times thinner free wall compared with the LV. The RV is therefore known to adapt much better to chronic volume overload than to pressure overload . An increase in preload has been demonstrated to improve myocardial contraction in the left heart . The Frank–Starling mechanism characterizes the increase in ventricular stroke work in response to wall stress enhancement. Because of a weak relationship between surface area and ventricular volume, the preload recruitable stroke work is moderate in the physiological RV. The Frank–Starling mechanism may only improve ventricular contraction in case of significant enlargement of the RV . On the other hand, excessive volume loading may be detrimental to the RV, because of acute impairment of coronary flow within the RV free wall .
RV afterload is defined by pulmonary vascular load. The pulmonary vasculature is characterized by a low resistance and high compliance profile. PVR is usually linked to RV afterload in normal conditions because of easy assessment in clinical practice. However, PVR only accounts for the resistive component of the pulmonary vascular load; as a result, PVR underestimates RV afterload by neglecting blood flow pulsatility and wave reflections through the pulmonary vasculature. Most pulmonary vascular diseases are known to disturb both components of the pulmonary vascular load. Pulmonary arterial impedance is probably the most complete measure of the pulmonary vascular load. Input impedance actually includes both static and dynamic components of RV afterload, and it may be a better predictor of long-term outcomes in PH than PVR alone ; its definition in the frequency domain is, however, restrictive for wide clinical use. Sunagawa et al. characterized the systemic arterial load in the time domain as effective arterial elastance (Ea) . Ea is defined as the change in pressure for a change in volume, and can therefore be linked to the effective arterial load that the ventricle has to overcome during ejection. Pulmonary Ea can be determined using pressure-volume loops analysis or estimated as the ratio of stroke volume to end-systolic pressure.
Assessment of RV pump function: the importance of RV-PA coupling
As RV function is the main determinant of survival in several cardiopulmonary diseases, there is major interest in investigating the right heart. Echocardiography is the mainstay in the assessment of RV function. The main limitation is the load dependency of most of these noninvasive indices. The RV is optimally matched to pulmonary vascular load in the normal state. Afterload mismatch appears to be the most important prognostic factor for RV systolic dysfunction in pressure overload conditions . Ventricular-arterial coupling is an important determinant of cardiac performance and energetics; it reflects maximal efficiency between hydraulic work production and myocardial oxygen consumption. RV-pulmonary arterial (RV-PA) coupling can be quantified in real time, using pressure-volume loops analysis, as the ratio of the RV end-systolic elastance (Ees) to the Ea ( Fig. 1 ). Ees is defined as the slope of the end-systolic pressure-volume linear relationship obtained by changes in ventricular filling . To be load-independent, this end-systolic relationship is studied during transient occlusion of the inferior vena cava, so that Ees is currently considered to be the most reliable measure of ventricular contractility.

Despite significant interspecies differences in basal pulmonary haemodynamics, Wauthy et al. showed that Ees/Ea changes similarly, secondary to RV pressure overload, in different animal species . Furthermore, RV adaptation to changes in loading conditions is relatively preserved through mammalian spontaneous evolution . Ranges of usual RV-PA coupling values among animal species are summarized in Table 1 . Impairment of RV-PA coupling is reflected in an Ees/Ea ratio of < 1 in large animals, whereas the normal Ees/Ea ratio ranges from 0.5 to 0.8 in rodents. At the early stage of RV pressure overload, Ees is often increased to compensate for the elevated afterload . However, RV-PA coupling may be decreased at that time, without RV dysfunction at rest. This is related more to an increase in Ea than to a significant decrease in Ees. When contractile performance becomes insufficient to overcome the elevated afterload, RV stroke volume and RV ejection fraction decrease. RV dilatation and adverse remodelling then occur, leading to RVF .
Animals | Weight (kg) | Methods a | Ees (mmHg/mL) | Ea (mmHg/mL) | Ees/Ea |
---|---|---|---|---|---|
Rat | 0.20–0.25 | Conductance | 82 ± 53 | 150 ± 60 | 0.53 ± 0.2 |
Mouse | 0.02–0.04 | Admittance | 1.8 ± 0.5 | 2.7 ± 1.2 | 0.71 ± 0.27 |
Dog | 25 | Single-beat | 1.11 ± 0.07 | 1.0 ± 0.06 | 1.13 ± 0.08 |
Goat | 24 | Single-beat | 2.0 ± 0.4 | 1.3 ± 0.1 | 1.7 ± 0.3 |
Piglet | 5.7–6.1 | Single-beat | 1.45 ± 0.08 | 0.91 ± 0.07 | 1.62 ± 0.09 |
Pig | 20–25 | Single-beat | 1.4 ± 0.2 | 0.9 ± 0.1 | 1.4 ± 0.4 |
a Methods of recording pressure-volume loops were specified as conductance or admittance catheterization, or as single-beat elastance.
The concept of ventricular-arterial coupling is not commonly used in clinical practice, mainly because it requires invasive and time-consuming measurement. Considerable efforts have been made to estimate the contractile performance of the RV from measurement of RV pressure and volume. Single-beat elastance is a promising method validated recently in clinical practice for the assessment of RV elastance without dramatic changes in loading conditions, using cardiac magnetic resonance imaging for volume assessment . Several echocardiography indices of RV function have been further reported to characterize the systole, such as tricuspid annular plane systolic excursion (TAPSE), RV fractional area change (RVFAC), RV myocardial performance index (RVMPI), stroke volume index (SVI) and, more recently, acceleration of the myocardium during isovolumic contraction (IVA) . Of all these indices, Vogel et al. showed that IVA may be the most relevant for RV contractility . There were two main limitations to this study. First, IVA (as well as TAPSE, RVFAC and RVMPI) is strongly load dependent, and thus may not accurately reflect RV pump function. Second, this study validated IVA as a contractility index for the RV in a ‘healthy’ animal model, not in the setting of chronic RV remodelling caused by either pressure or volume overload. We recently showed in a piglet model of chronic pressure overload for the RV, that usual noninvasive measures of RV systolic function were more strongly associated with RV-PA coupling than with contractility (Ees). Despite a marked improvement in Ees, RV systolic dysfunction was observed, with a marked decrease in TAPSE, RVFAC or RVMPI. This is in accordance with our hypothesis that commonly used indices of RV function do not appear to be specific for RV contractility, but rather reflect the interaction between RV pump function and pulmonary vascular load . However, the best way to assess contractile performance for the overloaded RV is still a matter of debate.
Clinical series in PH have highlighted that changes in RV function – rather than changes in pulmonary pressure or PVR – affect long-term survival dramatically . Impairment of RV contractility in both longitudinal and transverse planes is often observed at an advanced stage in the overloaded RV. This functional assessment is routinely performed at rest, whereas exercise capacity is a strong predictor of long-term outcome in cardiopulmonary diseases. RV contractile reserve has been poorly reported. Grunig et al. recently suggested that exercise-induced pulmonary artery systolic pressure increase could estimate RV contractile reserve, and may be a prognostic factor in PH . Pharmacological stress using dobutamine infusion is better adapted to heart failure patients than exercise testing, especially for echocardiography Doppler measurements during stress. Sharma et al. recently studied RV reserve using low-dose dobutamine stress echocardiography in PH patients . Contractile reserve for the RV was quantified as any significant changes in TAPSE and tricuspid annular systolic velocity (S′). The protocol was well tolerated in PH patients. This study showed that RV reserve was impaired in PH patients, even in those who displayed normal RV function at rest. Dobutamine stress may reveal early RV dysfunction in the setting of chronic pressure overload. These findings are in accordance with our experimental results. We recently reported that dobutamine-induced relative changes in RV stroke volume index (SVI) and Ees were lower in piglets exposed to chronic pressure overload. RV reserve, defined as any change in SVI during pharmacological stress, was strongly associated with resting ventricular-arterial coupling in this study . It could be a promising predictive measure for estimating RV response to therapy, as well as a prognostic marker, as long-term survival is strongly associated with the ability of the RV to durably face the elevated afterload.
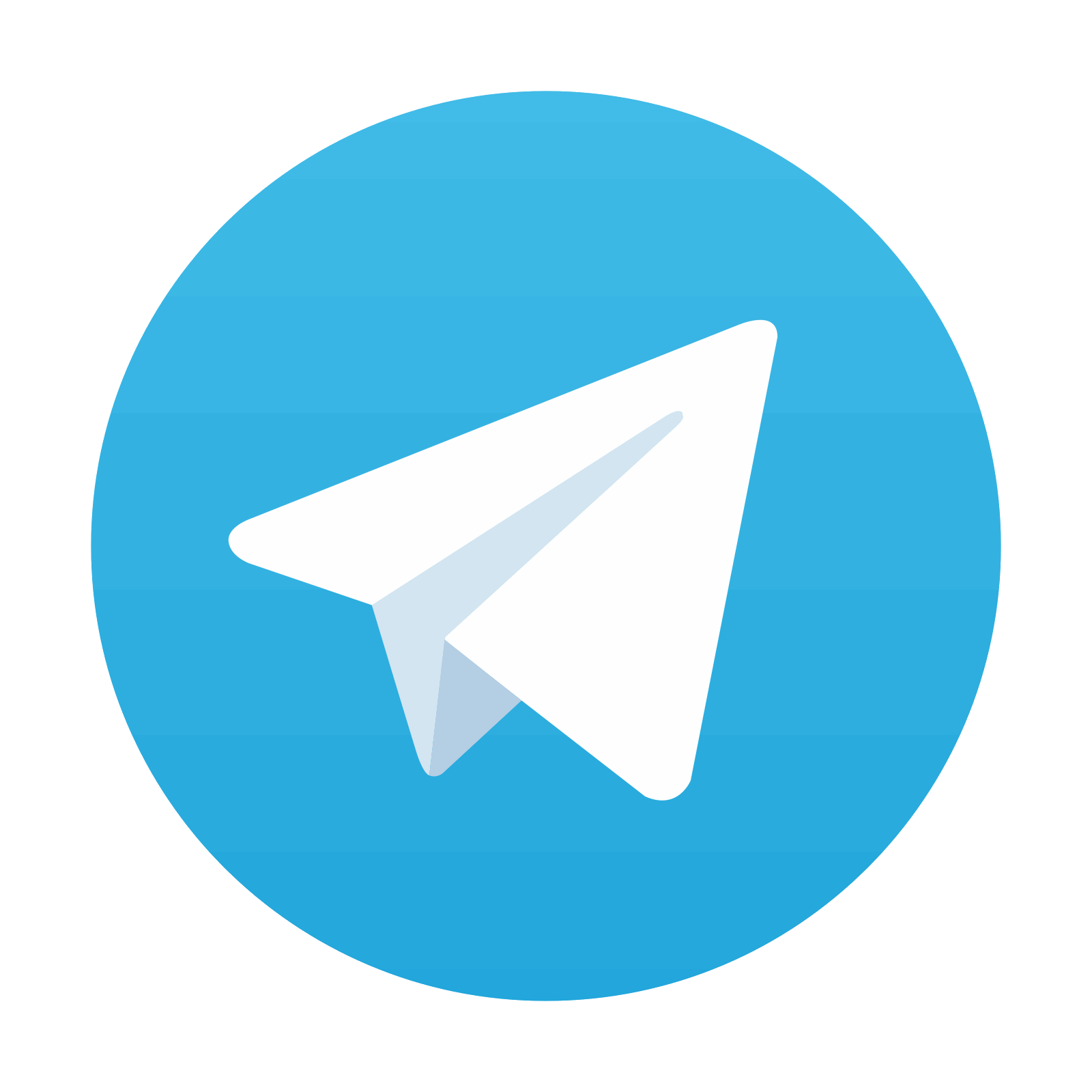
Stay updated, free articles. Join our Telegram channel

Full access? Get Clinical Tree
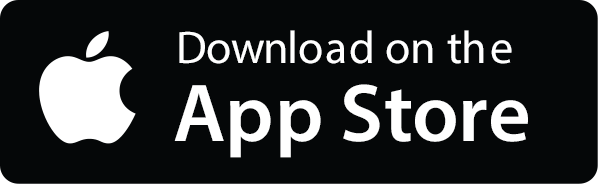
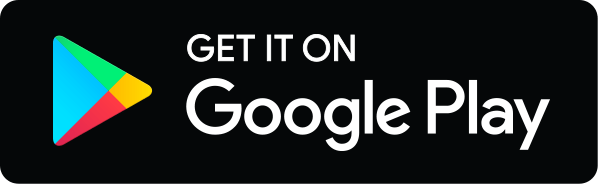