4
Advanced Echocardiographic Modalities
As detailed in Chapter 5, appropriate education and training in cardiac ultrasound is needed by these physicians. In addition, the cardiac sonographer and physician often are involved in ensuring optimal data acquisition and interpretation of these examinations. This chapter provides an introduction these other echocardiographic modalities. Intraoperative TEE echocardiography is discussed in more detail in Chapter 18. Advanced echocardiographers will want to read further on these topics as indicated in the Suggested Readings.
Three-Dimensional Echocardiography
Image Acquisition
Three-dimensional imaging utilizes a complex multiarray transducer that simultaneously acquires ultrasound data from a 3D pyramidal volume. Rapid parallel image processing provides ultrasound images that can be viewed in real time in any orientation on the screen (Fig. 4-1). These matrix array transducers typically include about 3000 piezoelectric elements with a transmission frequency of 2 to 4 MHz for TTE and 5 to 7 MHz for TEE. There are several approaches to the acquisition of echocardiographic data using a 3D matrix array transducer:
Figure 4–1 3D echocardiography.
3D echocardiographic images are acquired using a fully sampled matrix array transducer. 2D imaging (left) focuses the transducer beam on a single tomographic slice with optimal temporal and spatial resolution. Different 2D image planes are obtained by manually moving, rotating, and tilting the transducer. 3D real-time narrow sector imaging (center) uses a matrix array transducer to display a 300 × 600 pyramidal volume, which maintains high temporal resolution within this narrow volume image. Real-time imaging also can be enlarged to include the entire anatomic structure in “zoom” mode at the expense of decreased spatial and temporal resolution. Full-volume imaging (right) stitches together volumetric image data from more than one cardiac cycle (typically four beats, as shown here) to provide higher temporal and spatial resolution while including the entire cardiac anatomy in the field of view.
Real-time narrow 3D section: A beat-by-beat view with a wider image plane than standard two-dimensional (2D) imaging that can be rotated to view from different perspectives—looks like a “thick” tomographic image.
Real-time 3D-zoom volume rendered images: A full-volume image of an enlarged area of interest that is rotated to show the structure of interest in a “surgical” view. These images are displayed with a perspective-type image similar to a photographic view from inside the heart.
Full-volume gated acquisition volume rendered images: Multiple beat volumetric imaging stitches together narrow volumes of data over several cardiac cycles to provide a full volume of data that can be rotated and cropped to show the structures of interest.
Simultaneous multiplane mode: Simultaneous display of two 2D image planes with the ability to adjust the rotation angle, tilt and elevation of the second image plane.
3D color Doppler imaging: Real-time or full-volume color Doppler data acquisition, but at frame rates lower than for imaging data.
Advantages of real-time narrow sector imaging are rapid image capture, familiar image planes, and evaluation of complex anatomy; however, only a narrow field of view is seen (Fig. 4-2). With focused wide section or real-time “zoom” mode, the entire structure (such as the mitral valve) is included in the image, but spatial and temporal resolution are poor, and the image must be rotated to display the internal cardiac anatomy (Fig. 4-3). Full-volume gated images look similar to real-time zoom mode images but have better spatial and temporal resolution. Full-volume images also can be analyzed after acquisition to provide additional views. Simultaneous multiplane imaging shows only a few (typically two) tomographic images but provides the highest temporal and spatial resolution within these image planes (Fig. 4-4). Three-dimensional color Doppler imaging is helpful for showing the spatial distribution of a flow disturbance, such as prosthetic paravalvular regurgitation or an intracardiac shunt, but currently has very low temporal resolution.
Figure 4–2 Real-time 3D narrow section volume rendered imaging. A standard 2D parasternal long-axis view (top) has a frame rate of 50 Hz, which drops to 5 Hz in the 3D mode (bottom).
Figure 4–3 Real-time 3D “zoom” volume rendered imaging.
This real-time TEE image has been rotated to show the mitral valve from the LA perspective. In systole, the closed anterior leaflet (A) is bounded by the “smile” of the curved leaflet closure line with the two commissures (arrows) separating it from the posterior leaflet (P).
Figure 4–4 TTE simultaneous multiplane imaging.
From the parasternal window, both the long-axis and short-axis views of the aortic valve can be recorded on the same cardiac cycles.
During the acquisition of 3D images, transducer position is adjusted to optimize visualization of the structure of interest; for example by imaging the mitral valve from the left atrial (LA) side on TEE imaging with the ultrasound beam perpendicular to the closed mitral leaflets. Next, gain and compression are set in the mid-range (about 50 units) and the time-gain-compensation (TGC) curve adjusted so the image is slightly “over gained” to avoid echo-dropout appearing as “holes” in anatomic structures. With real-time imaging, transducer position and gain can be adjusted iteratively to improve image quality and to center the structure of interest in the image. My practice is to optimize position and gain on a zoomed real-time 3D view before the acquisition of a four beat gated full-volume data set from the same transducer position. Postprocessing, gain and compression then can be adjusted after image acquisition. With full-volume gated acquisitions, any change in heart position from beat to beat may result in a vertical line across the image with misregistration of the image data on both sides of this “stitch” artifact. Causes of a stitch artifact include patient movement, respiratory motion, and an irregular heart rhythm.
Image Display
There are currently several types of 3D echocardiographic image displays, including:
In both the real-time 3D zoom mode and in full-volume imaging, the display is “cropped” to show different views of the interior structures of the heart. For example, the mitral valve can be viewed from the perspective of the LA; this provides a compelling view of prolapsing segments of the valve in patients with myxomatous mitral valve disease (see Fig. 12-30). The image can then be rotated and recropped to show a long-axis type image of the mitral valve or to view the valve from the left ventricular (LV) side. Similarly, the aortic valve can be viewed en face from the perspective of the aorta, a view that correlates closely with the surgical view of valve anatomy, from the LV side of the valve or in a long-axis orientation. Real-time 3D images are cropped and rotated as the images are acquired. Full-volume gated acquisitions can be cropped and rotated during the exam but also can be reevaluated later because the full-volume data set is saved digitally.
Surface rendered images or wireframe displays are based on identifying the boundaries of a cardiac structure, either by using semiautomated methods or by tracing the boundaries on multiple 2D images. For example, the LV endocardial surface is shown as a 3D solid structure with contraction shown by a sequence of 3D volumes over the cardiac cycle, so the rendered volume appears to beat on the display screen (Fig. 4-5). Alternatively, a wireframe-type display can be used. A graphical display also may be helpful with time on the horizontal axis and with either LV volume or the position of each myocardial segment shown on the vertical axis (see Fig. 6-9).
Figure 4–5 Surface rendered volumetric imaging.
The apical window was used to acquire a full-volume 3D image of the LV. Using three orthogonal planes through the data set for guidance, endocardial borders were traced using semiautomated border detection to provide a surface-rendered image of the ventricular chamber, with color coding for myocardial regions. The graphical curve shows LV volume over a single cardiac cycle.
The 3D echocardiographic data set also can be used for simultaneous display of multiple image 2D planes (Fig. 4-6). The ability to acquire LV images in multiple planes simultaneously may speed image acquisition during stress echocardiography, potentially improving diagnostic accuracy. In addition, the ability to “move through” a 3D data set in any 2D image plane will allow better appreciation of cardiac anatomy in patients with complex structural heart disease and allow precise localization of abnormalities.
Figure 4–6 Multiple simultaneous 2D image planes.
The 3D full-volume image of the LV recorded from the apical window is displayed as multiple tomographic short-axis views spanning the ventricle from apex (top left) to base (lower right).
Examination Protocol
In routine clinical practice, 3D imaging is used as an adjunct to the 2D study only in selected cases. Often 3D imaging focuses on a particular structure of interest, such as a prolapsing mitral valve or an atrial septal defect. A systemic 3D study has not yet become routine. However, the American Society of Echocardiography (ASE) and the European Association of Echocardiography (EAE) have made recommendations for an imaging protocol and for standard orientation of 3D echocardiographic views. With TTE imaging, typically only limited 3D imaging is performed to complement a full 2D study. Examples of a focused 3D study include 3D quantitation of LV volumes and ejection fraction in a heart failure patient (see Fig. 9-4), 3D measurement of mitral orifice area in a patient with mitral stenosis, or 3D short-axis images of the aortic valve in a patient with bicuspid aortic valve disease (see Fig. 11-6). With TEE imaging, a systematic approach to 3D image acquisition and display is recommended, with additional views as needed depending on the specific pathology (Table 4-1).
TABLE 4-1
ASE/EAE Recommendations for a Systematic 3D Study
∗3D images of the tricuspid valve are best obtained from transthoracic, not TEE, imaging.
Summarized from Lang RM, Badano LP, Tsang W, et al: EAE/ASE recommendations for image acquisition and display using threedimensional echocardiography. J Am Soc Echocardiogr 25(1):3-46, 2012.
Recommendations for volume-rendered 3D image displays (Fig. 4-7) are:
Figure 4–7 ASE/ESE recommendations for image orientation of cardiac valve.
Three-dimensional TEE images of cardiac valves should be recorded in standard orientations as shown in the right column and in Table 4-1. First, the valve is centered in a 2D TEE view of the valve as shown in the first column. Next, real-time 3D is used to optimize gain settings for visualization of valve anatomy. Then a full-volume image is acquired and is rotated around the x-or y-plane as shown followed by rotation in the image plane (except for the aortic valve) to show the valve in the standard display format. Aortic, mitral, and pulmonic valves are best imaged on TEE as shown. Tricuspid valve 3D TTE imaging is recommended using the TTE views shown. (From Lang RM, Badano LP, Tsang W, et al: EAE/ASE recommendations for image acquisition and display using 3D echocardiography. J Am Soc Echocardiogr 25[1]:3-46, 2012.)
Aortic valve: The right coronary cusp is located inferiorly (at 6 o’clock position) for both aortic and LV views of the valve (see Fig. 3-21).
Mitral valve: Aortic valve is located at the top of the image so the anterior mitral leaflet is superior to the posterior leaflet for both LA and LV views of the valve (see Fig. 3-23).
LV: 3D TTE views of the LV are oriented like standard 2D images in either an apical four-chamber view (apex at the top of the image, LV on the right side of the screen) or a short-axis view.
Right ventricle (RV): A four-chamber view or short-axis view is oriented with the LA superior (12 o’clock position).
Pulmonic valve: The anterior valve cusp is located superiorly (12 o’clock position) for both the pulmonary artery and RV sides of valve.
Tricuspid valve: The ventricular septum is placed inferiorly for both the right atrial (RA) and RV views of the valve.
Interatrial septum: From the LA side, the right upper pulmonary view is shown in the 1 o’clock position. From the RA side, the SVC is at the 11 o’clock position.
LA appendage: This display shows the LA appendage en face from the LA perspective with the pulmonary veins shown superiorly or longitudinally.
Quantitation from 3D Images
Each of these parameters can be displayed on a 3D perspective color-coded LV shape or as a graph over the cardiac cycle (see Fig. 18-7).
Compared to 2D approaches, 3D quantitation of LV function avoids geometric assumptions and is more accurate and reproducible and thus is recommended when technically feasible (see Chapter 6). Three-dimensional measures of LV mass, regional strain, curvature, and wall stress are more complicated and are currently investigational approaches.
Potentially other 3D measurements have advantages compared to 2D measurements for nonplanar structures, such as a stenotic valve. For example, although experienced operators can accurately measure mitral valve area from 2D images aligned at the minimal orifice area in patients with rheumatic mitral stenosis, inexperienced operators show improved accuracy with 3D imaging, which reliably shows the stenotic orifice and is less dependent on transducer position or image plane positioning. For planimetry of mitral valve area, the 3D volume is acquired and then a 2D plane is aligned at the minimal orifice with the valve opening traced in mid-diastole (Fig. 4-8). In research applications, more complex structures, such as the mitral leaflets and annulus, can be reconstructed in 3D by tracing the structure in a series of 2D image planes within the 3D volumetric data set (Fig. 4-9).
Figure 4–8 3D measurement of mitral valve area.
A full-volume image of the mitral valve was acquired (same patient as Fig. 11-10) in a patient with mitral stenosis and asymmetric fusion of the commissures. For measurement of the mitral valve area, offline analysis of the 3D volume used three orthogonal planes (x, y, z) shown in red, green, and blue to align an image plane at the tips of the stenotic valve. The resulting tomographic image at the minimal orifice area in diastole (lower left) was traced to determine mitral valve area.
Figure 4–9 3D reconstruction of the mitral valve apparatus by using real-time 3D.
3D echocardiography and a novel software system are used for mitral valve quantification. Left panel, The 3D volumetric image is automatically cropped into 18 equally spaced radial planes. The mitral annulus and leaflets are traced in each cropped plane. From these data, 3D images of the mitral leaflets and annulus are reconstructed. Right upper panel, Reconstructed 3D image that shows the actual configuration of the curved annulus and leaflets with surface colorations. In this case with ischemic MR, the mitral annulus is flattened with apparent tenting of the mitral leaflets, which were tethered into the LV, showing mountain-shape leaflet bulging. Right lower panel, Mitral leaflet configuration is represented in contour to appreciate the degree of tenting in the vertical view from the LV. Blue dots indicate coaptation line. (Courtesy Nozomi Watanabe, MD, Kawasaki University, Okayama, Japan.)
Clinical Utility
The clinical role of 3D echocardiography will continue to evolve as this technology matures. In addition to providing more detailed anatomic relationships and more accurate quantitation, 3D images are more intuitive than 2D images, allowing quicker appreciation of cardiac anatomy by more health care providers (Table 4-2). Potentially, 3D echocardiography could be faster than 2D scanning and could reduce variability in image acquisition. However, because instrumentation is in development, 3D echocardiography is not yet a routine part of the clinical examination at all centers and typically is used to supplement the 2D study in selected patients, with imaging focused on a specific anatomic structure. Three-dimensional imaging is more widespread for intraoperative and intraprocedural imaging because of the improved image quality and the additive value of the 3D perspective in these clinical settings (see Chapter 18).
TABLE 4-2
Clinical Applications of 3D Echocardiography
Application | 3D Approach | Comments |
LV function | Surface-rendered LV volumes, ejection fraction and regional wall motion derived from a gated full-volume 3D acquisition | 3D echo underestimates LV volumes compared to CMR data. Trabeculae and papillary muscles are included in the LV chamber. |
RV function | Volume-rendered images allow visualization of entire RV. Surface-rendered images may allow measurement of volumes and ejection fraction. | 3D measurement of RV volumes and ejection fraction requires further validation but is a promising approach. |
Mitral valve | Volume-rendered images show mitral valve anatomy en face from the LA or LV side of the valve. Accurate measurement of valve area in mitral stenosis using 3D guided 2D image planes Annular shape and dimensions from volumetric images 3D color Doppler shows jet origin and direction. | 3D TEE is recommended for guidance of interventional mitral valve procedures. 3D TTE or TEE is recommended for clinical evaluation of mitral valve pathology. |
Aortic valve and sinuses | Volume-rendered images obtained from TTE parasternal or TEE high-esophageal views provide optimal spatial resolution. Planimetry of aortic valve area is possible on 2D images derived from the 3D full-volume data set. 3D images demonstrate the oval shape of the aortic annulus. | 3D imaging may be helpful in determining the mechanism of aortic regurgitation and defining the number of valve leaflets. 3D imaging is recommended for guidance of transcatheter aortic valve implantation. |
Pulmonic valve and pulmonary artery | The pulmonic valve can be imaged using biplane or real-time 3D imaging. | Routine 3D pulmonic valve imaging is not recommended. |
Tricuspid valve | 3D volume-rendered images of the tricuspid valve are acquired in a similar fashion to those for the mitral valve. | 3D views of the tricuspid valve may be helpful in determining the mechanism of valve regurgitation. |
LA and RA | 3D volume-rendered images of the atrial septum are helpful for defining the location, size, and shape of atrial septal defects and for guiding transcatheter closure procedures. | 3D imaging may improve assessment of LA volume but is not a routine measurement. |
LA appendage | 3D volume-rendered images are helpful in guiding transcatheter LA appendage closure. | Biplane imaging of the LA appendage is useful in evaluating for LA thrombus. |
3D stress echocardiography | 3D imaging provides simultaneous evaluation of wall motion in all myocardial segments, improved visualization of the LV apex, and rapid image acquisition at peak stress. | Disadvantages of 3D stress imaging include lower frame rates and spatial resolution compared to 2D imaging. Not all 3D systems allow side by side review of rest and stress images. |
Summarized from Lang RM, Badano LP, Tsang W, et al: EAE/ASE recommendations for image acquisition and display using threedimensional echocardiography. J Am Soc Echocardiogr 25(1):3-46, 2012.
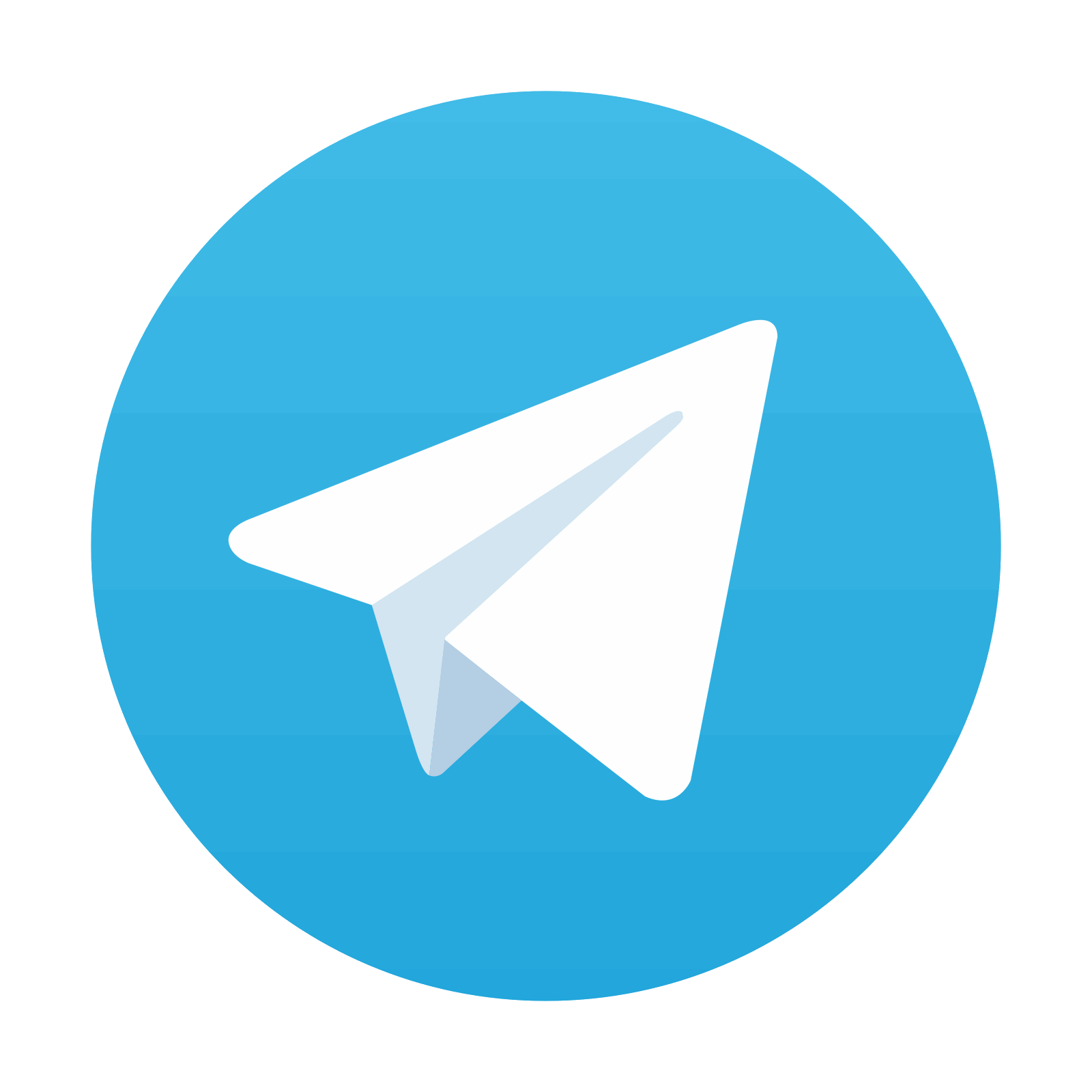
Stay updated, free articles. Join our Telegram channel

Full access? Get Clinical Tree
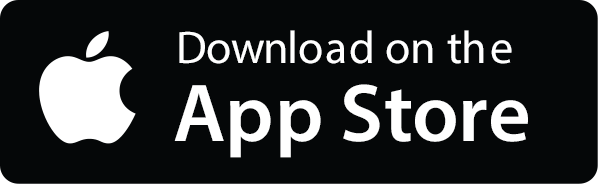
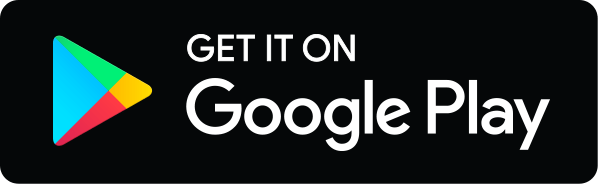