Adult Congenital Heart Disease
Philip Kilner
Sonya V. Babu-Narayan
This chapter considers the uses of magnetic resonance imaging (MRI), magnetic resonance angiography (MRA) and, where specifically important, computed tomography (CT) in the assessment of adolescents and adults with congenital heart disease (CHD). The number of such patients is increasing because of the success of surgical, catheter, and other interventions. The effects of congenital disease, surgery, and acquired disease may combine to create varied and challenging pathology (1,2 and 3). Residual structural and functional abnormalities generally must be followed on a lifelong basis, preferably at a specialist referral center. Appropriate imaging facilities represent a key aspect of such a centre. Cardiovascular MRI can make outstanding contributions to the assessment of CHD in adults, in whom body size and the results of surgery tend to limit echocardiographic access. The costs of imaging should be weighed against the potential costs of inappropriate management, which can entail complicated repeated surgery and extended hospitalization.
Imaging specialists need not be deterred by the anatomic variability found in these patients as discussion with the CHD specialist can clarify questions to be answered, and the comprehensive anatomic coverage offered by MRI almost always provides useful diagnostic information. Variations of anatomy and function do, however, mean that decisions on cine imaging planes and sequences generally must be made during acquisition. It is not only anatomic structures that vary; surgical techniques have also evolved and changed. Operations for conditions such as transposition of the great arteries (TGA) and heart with only one effective ventricle have changed radically during the last few decades, and those investigating older patients need to be aware of both previous and current surgical practices. A number of reviews of MRI of CHD have been published (4,5 and 6), and information on the investigation and management of malformations has been put together by the Canadian Consensus Conference on Adult Congenital Heart Disease (7), conveniently accessible through the Web site of the Canadian Adult Congenital Heart Network (see www.cachnet.org/res_managing.shtml for information on managing congenital heart defects).
The diagnostic contributions of MRI tend to complement those of transesophageal echocardiography. The latter can be more effective than MRI for imaging thin, mobile intracardiac structures such as the atrial septum, valve structures, and the vegetations of endocarditis. Diagnostic cardiac catheterization is rarely needed now, and interventional catheterization may be expedited after MRI and transesophageal echocardiography. Further, information from MRI can direct cardiac catheterization and thus shorten radiation exposure. However, adequate assessments of coronary artery stenoses or pulmonary vascular resistance still rely on invasive investigations. Uses of MRI-guided catheterization (8) include attempted measurement of pulmonary vascular resistance by MRI flow combined with catheter pressure measurement (9).
Since many adults with CHD have a complex clinical history with multiple complementary investigations, collaborative presentation and discussion can be important, allowing a comprehensive picture to be built up that can clarify questions
of clinical management and further the process of learning. At such meetings, MRI studies should be shown with interactive interrogation of multislice, cine, velocity, and threedimensional (3D) acquisitions.
of clinical management and further the process of learning. At such meetings, MRI studies should be shown with interactive interrogation of multislice, cine, velocity, and threedimensional (3D) acquisitions.
Cardiovascular MRI can provide functional as well as anatomic information, including the location and severity of stenoses (e.g., aortic coarctation or pulmonary artery stenosis), severity of regurgitation (e.g., pulmonary regurgitation), size and function of the heart chambers (the right as well as the left ventricle [LV]), and measurements of shunt flow. In adults with CHD, the underlying cardiac anatomy is likely to have been assessed previously. Although it is important to understand the descriptions, they should not necessarily be assumed to be correct and should be reviewed in the light of new information gained from the MRI study. The terminology used for malformed cardiovascular anatomy is described in Chapter 29.
CT offers unrivalled 3D spatial resolution in shorter acquisition times than MRI. In patients with CHD, it has particular roles in the visualization of anomalous coronary arteries and coronary arteries in relation to RV to PA conduits where percutaneous dilatation and valve placement are considered, and in the visualization of aortopulmonary collateral arteries, calcification, and stents in various locations. ECG-gated cine CT allows measurements of biventricular size and function, albeit at a lower temporal resolution than MRI, and subject to adequate opacification of each intraventricular blood volume. In patients with a pacemaker or an ICD, CT provides an alternative to MRI. However, exposure to ionizing radiation can be an issue, especially in relatively young patients who may need repeated follow-up (10).
TECHNIQUES
MULTISLICE SCOUT IMAGING
In CHD, it is advisable to acquire coronal and sagittal as well as transaxial multislice images. To help with tissue characterization, a full transaxial stack of half-Fourier acquisition single-shot turbo spin-echo (HASTE) images should be acquired. We recommend following this with multislice steady-state free precession (SSFP) image stacks in transaxial, coronal, and sagittal orientations. These bright blood acquisitions provide an overview of cardiovascular anatomical structures and connections, including the pulmonary veins. These stacks should, ideally, each be acquired during a breath-hold to assist accurate location of subsequent breathhold cine acquisitions.
CINE AND MULTISLICE CINE IMAGING
SSFP imaging, which gives good contrast between blood and myocardium, is well suited for imaging and measuring ventricular function and mass and for visualizing valve leaflets. Where there is jet flow through a stenotic or regurgitant orifice, SSFP cine imaging can outline a coherent jet core, if present, because of the localized loss of signal from shear layers (Fig. 31.1). Rapid acquisition makes it possible to interrogate a jet area precisely and repeatedly. The approach of “cross-cutting”—locating an orthogonal slice through a partially visualized feature such as a valve orifice or jet— is an effective way of “homing in” on a particular feature of interest by MRI. In addition, or alternatively, contiguous stacks of cine images are easy to acquire and extremely valuable for relatively comprehensive coverage of anatomical regions or structures. In the case of thin structures such as the leaflets of the mitral valve or the atrial septum and any defect in it, the orientation of cine slices, which typically have 5- to 7-mm slice thickness, is a key consideration. Due to possible partial volume effects, a thin structure is only likely to be visualized clearly by cine slices oriented perpendicular or near perpendicular to it.
FLOW VELOCITY ACQUISITIONS
Phase-shift velocity mapping, if correctly implemented, can be an accurate and versatile way of measuring flow (11,12).
However, the development of MRI systems with rapidly switching gradients for cardiovascular acquisition has led to potential inaccuracies in velocity mapping, particularly when used for measurements of shunt flow and regurgitant fraction. In these cases, a slight shift of the background phase, due to eddy currents or any uncorrected Maxwell gradients, can introduce significant errors in flow calculation. Manufacturers have gradually become aware of these problems and have implemented modifications of hardware and software to avoid or counteract them in more recent systems. Unfortunately, however, it cannot be assumed that all systems in current clinical use are accurate in this respect (13).
However, the development of MRI systems with rapidly switching gradients for cardiovascular acquisition has led to potential inaccuracies in velocity mapping, particularly when used for measurements of shunt flow and regurgitant fraction. In these cases, a slight shift of the background phase, due to eddy currents or any uncorrected Maxwell gradients, can introduce significant errors in flow calculation. Manufacturers have gradually become aware of these problems and have implemented modifications of hardware and software to avoid or counteract them in more recent systems. Unfortunately, however, it cannot be assumed that all systems in current clinical use are accurate in this respect (13).
Phase-shift velocity mapping is based on the frequency changes experienced by nuclei moving relative to applied magnetic gradients (11,12). The direction, steepness, and timing of the applied gradients can be chosen, so that flows with a range of velocities, from low-velocity venous to high-velocity poststenotic flows, can be measured accurately. Clinical uses include measurements of cardiac output, valvar heart disease (14), shunt flow (15), collateral flow (16), regurgitant flow (17), and jet velocities through stenoses (18). However, for successful clinical application, the operator should have an understanding not only of the anatomy and pathophysiology of the operated CHD, but also of the available technical choices for velocity mapping. It is necessary to select a plane, echo time, velocity-encoding direction, and velocity sensitivity appropriate for a particular investigation.
Velocity can be encoded in directions that lie either in or through an image plane. The mapping of velocities through a plane transecting a vessel (velocity encoded in the direction of the slice-selection gradient) allows the measurement of flow volume. The cross-sectional area of the lumen and the mean axially directed velocity within that area are measured for each phase through the heart cycle. From these data, a flow curve is plotted, and systolic forward flow and any diastolic reversed flow are computed by integration.
To calculate shunt flow, both aortic and pulmonary artery flows are measured. Two separate acquisitions are generally needed, except in cases of TGA, in which the aorta and pulmonary trunk usually run almost parallel to each other. For aortic flow measurement, a suitable plane transects the aortic root at the level of the sinotubular junction, and for pulmonary flow measurement, a suitable plane transects the pulmonary trunk proximal to its bifurcation. Pulmonary regurgitation is common after repair of tetralogy of Fallot, and in such cases, the repaired outflow tract and pulmonary trunk are tethered by scarring. However, if the mobility of the aortic and pulmonary valves is normal, especially in cases in which the root is dilated, the diastolic regurgitant flow can be significantly underestimated because of compliance and movement of the arterial root relative to the imaging plane. A potential solution to this problem will be movement of the acquisition plane to follow valve movement through the heart cycle—“motion tracking.”
Through-plane velocity mapping can be used to delineate an atrial septal defect and measure the velocities of jets through stenotic orifices (18). Alternatively, it can be helpful to map velocities in a plane aligned with the direction of flow, with velocity encoded in the read-gradient direction, which must also be aligned with the direction of jet flow. This allows depiction of the jet in relation to upstream and downstream regions. It is necessary, however, to locate the image plane correctly by cross-cutting.
Jet velocity mapping can be particularly valuable for assessing stenoses in which ultrasonic access is limited, such as in aortic coarctation (native or repaired), ventriculopulmonary conduits, pulmonary artery branch stenoses, and obstructions at the atrial or atriopulmonary level following a Mustard, Senning, or Fontan operation.
The acquisition of all three components of velocity relative to voxels distributed throughout a 3D volume for each of 20 or more phases through the cardiac cycle is also feasible. Such four-dimensional (4D) flow datasets require relatively long acquisition times of about 10 to 25 minutes (19). This of course means that any instabilities or other beat-to-beat variations of flow are effectively averaged out, which makes attempted calculations of streaming, mixing, and energy dissipation of fluid-wall shear problematic. However, an important potential advantage, particularly in complex CHDs, is that multiple cavities, valves, and vessels can be covered in a single acquisition. The relative volumes of flow passing through multiple valve orifices and vessels can theoretically be calculated retrospectively (20). This requires relatively advanced postprocessing, which is not yet widely available.
THREE-DIMENSIONAL ACQUISITIONS WITH AND WITHOUT CONTRAST
Gadolinium-enhanced 3D MRA combines fast acquisition with good spatial resolution. Important applications of MRA in adults with CHD are depiction of anomalous pulmonary or systemic venous return, depiction of the geometry and dimensions of the aorta in the presence of coarctation with a view to balloon dilatation and stenting, and depiction of the size and distribution of aortopulmonary collaterals and surgical shunts in pulmonary atresia (Fig. 31.2). Although there may be some trade-off between spatial resolution and rapidity of acquisitions, shorter acquisitions allow several phases of contrast passage to image sequentially in a single breath-hold, which is valuable in the presence of complex cardiovascular connections or shunts.
Contrast-enhanced MRA is generally acquired in a breath-hold without cardiac gating, but this approach is not suitable for coronary angiography due to the relative importance of cardiac motion. For angiographic visualization of the proximal course of coronary arteries, ECG-gated 3D SSFP acquisitions can be used, either in a single breathhold or with diaphragm monitoring that accepts only data acquired during a limited part of respiratory cycles over a period of minutes. The suppression of signal from fat improves the delineation of coronaries. In the diagnosis and assessment of Kawasaki disease, CVMR angiography can be used to visualize coronary artery aneurysms or stenosis. ECG-gated 3D SSFP acquisitions are also widely used for relatively high resolution anatomic evaluation in CHD and, given the relatively bright signal from blood in all cavities, as the basis of 3D “road maps” to guide electrophysiologic
interventions (21). Acquisition can be in more than one phase of the cardiac cycle (22).
interventions (21). Acquisition can be in more than one phase of the cardiac cycle (22).
MEASUREMENT OF RIGHT VENTRICULAR FUNCTION AND MASS
Ventricular measurements have been described in greater detail elsewhere. In CHD, measurements of the right ventricle (RV) are at least as important as those of the LV, but they pose particular challenges. The RV cavity is crossed by coarse trabeculations, particularly near the apex. These become thick and significant in summed volume when the RV is hypertrophied, but even if clearly visualized, trabeculations are difficult to outline individually because of their complex structure. Furthermore, the base of the RV tends to be more difficult to delineate than that of the LV. After repair of tetralogy of Fallot, the RV outflow tract can be dilated and dyskinetic and may have no effective pulmonary valve. All these factors can make it difficult to decide on the limits of the RV. It is usual to include a dilated or aneurysmal outflow tract as part of the RV, where it lies beneath the level of the pulmonary valve annulus (4). A particular unit or researcher must establish a reproducible protocol for the acquisition and analysis of RV function, which, despite difficulties, are likely to prove more reliable than those obtained by any other imaging modality.
LATE GADOLINIUM ENHANCEMENT
Late gadolinium enhancement (LGE) imaging, which makes use of the property of intravenously injected gadolinium chelate to linger in extracellular spaces in scarred myocardial tissue after its concentration has begun to fall in the bloodstream, is described more fully elsewhere. Ventricular fibrosis imaging by LGE has been used for investigation of patients with CHD, particularly after surgery. Scarring has been found to be common in distinct locations. These are often directly attributable to the interventions performed, for example, scarring of the wall of the partially resected infundibulum in patients after repair of Tetralogy of Fallot (23). Scarring of the systemic RV in patients who had previously undergone Mustard or Senning procedures for TGA was found to relate to adverse ventricular function and incidence of arrhythmias (24). Evidence is accumulating that abnormally extensive LGE correlates with, and may potentially predict, arrhythmia.
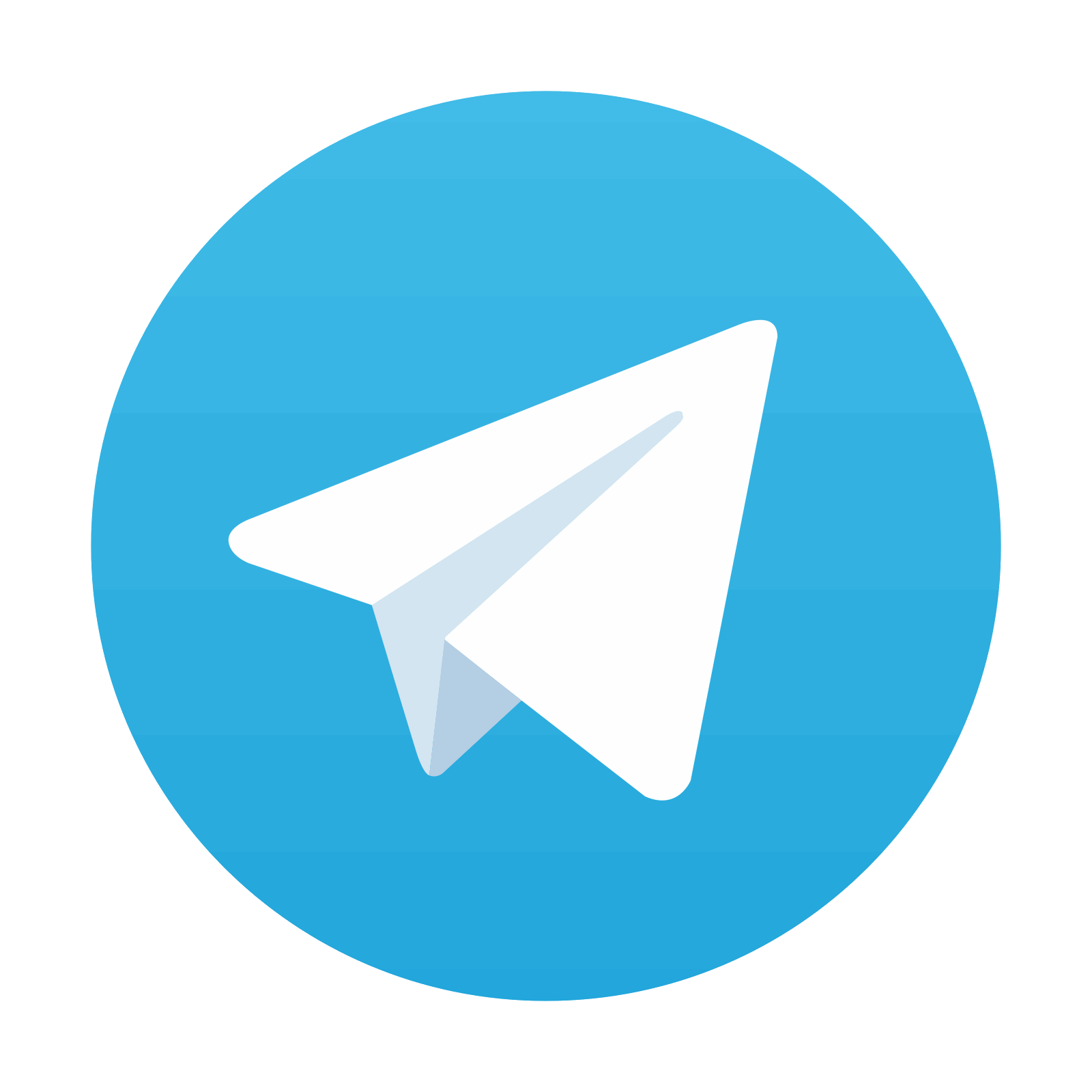
Stay updated, free articles. Join our Telegram channel

Full access? Get Clinical Tree
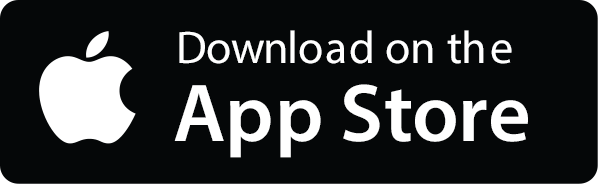
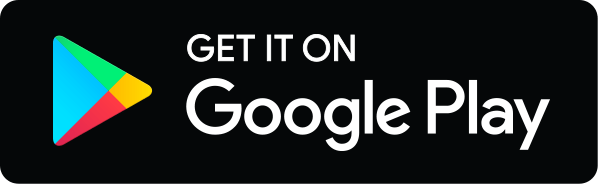