Fig. 1
Structure of adrenomedullin. h-AM is formed by a ring structure of 52 amino acid residues held by a disulfide bond between Cys-16 and Cys-21; with a Tyr residue amidated in the C-terminal portion. These structural features are necessaries for its biological activity
AM immunoreactivity, AM mRNA and the peptide itself are found in the circulation and in lung, spleen, kidney, heart, adrenal gland and brain. AM has also been found to be secreted from endothelial and vascular smooth muscle cells [5, 42, 44, 104, 105]. AM is involved in the regulation of blood pressure and body fluid balance. In effect, systemic AM administration elicits potent, dose-dependent vasodilatatory effects, reduces blood pressure, increases urine output and urinary sodium excretion. On the other hand, AM is an antioxidant substance, since AM attenuates reactive oxygen species (ROS) production mediated by the stimulation of nicotinamide adenine dinucleotide phosphate-oxidase (NAD(P)H oxidase) [49, 50, 76, 92, 112, 113]. Additionally, AM may directly inhibit NAD(P)H oxidase activity eliciting protective effects [76]. Thus, it is clear that AM is capable to reduce free radicals and ROS production mediated by NAD(P)H oxidase stimulation, through inhibition of enzyme activity [76].
AM exerts multiples biological activities through the activation of two specific receptors, the AM type 1 (AM1) and type 2 (AM2) receptors, formed from the obligate co-expression of a class-B, G protein coupled receptor (GPCR), the calcitonin receptor-like receptor (CRLR) and receptor activity-modifying proteins (RAMPs) 2 or 3, respectively [4, 61, 74]. The calcitonin gene-related peptide 1 (CGRP1) receptor is formed of a complex between CRLR and RAMP1 [61]. Thus, the CRLR/RAMP1 heterodimer defines the CGRP1 receptor and the association between RAMP2 or RAMP3/CRLR forms a functional AM1 and AM2 receptor, respectively [54] (Fig. 2). AM1 receptors are highly selective for AM over CGRP and other peptides, while AM2 receptor binds both AM and AM2 (intermedin) with high affinities [43]. AM also has appreciable affinity for the CGRP1 receptor [36]. Thus, RAMPs regulates the ligand selectivity of CRLR [5]. CGRP1 receptor is effectively antagonized by CGRP(8-37); meanwhile AM1 receptor is more effectively blocked by a selective AM receptor antagonist, h-AM(22-52) or r-AM(20-50) than by CGRP(8-37). Unlike the pharmacological features of the AM1 receptor, AM2 receptor differs among species. In h-AM2 receptors, AM is more effectively blocked by a selective AM-receptor antagonist, but in r-AM2, the CGRP1 receptor antagonist are more potent than AM-blockers [54].


Fig. 2
Adrenomedullin type 1 (AM1) and type2 (AM2) receptors, formed from the obligate co-expression of the calcitonin receptor-like receptor (CRLR) and receptor activity-modifying proteins (RAMPs) 2 or 3, respectively. Thus, CRLR/RAMP2 heterodimer (red and purple) or CRLR/RAMP3 (blue and purple) preferentially bind AM. While the calcitonin gene-related peptide 1 (CGRP1) receptor is formed of a complex between CRLR and RAMP1 (green and purple) and preferentially bind CGRP [61]
AM receptors and binding sites for AM are found in several body regions [21]. The CRLR mRNA and peptide are predominantly expressed in the lung, blood vessels, liver, midgut, rectum, urethra, adrenal cortex, uterus, coronary artery endothelial and smooth muscle cells [37, 54, 72]. The distribution of RAMP1 expression have been reported in fat, thymus, spleen, uterus, pancreas and bladder; while RAMP2 and RAMP3 is expressed in the lung, kidney, heart, liver, spleen, uterus, ovary and placenta [21, 36, 54, 61, 67].
2 Adrenomedullin Receptor Subtypes in the Central Nervous System
AM and its receptors are locally produced and expressed in the central nervous system (CNS) and are particularly localized to the autonomic nuclei, including nucleus tractus solitarii (NTS), lateral parabrachial nucleus and rostral ventrolateral medulla, cerebral cortex, pituitary gland, thalamus, hypothalamus, brainstem, medulla oblongata, midbrain, amygdala and cerebellum [5, 42, 47, 58, 73, 86, 88, 107]. RAMP1 mRNA is found in the hippocampus, nucleus accumbens, caudate–putamen, cerebral cortex and cerebellum; RAMP2 mRNA is expressed in numerous brain areas, including autonomic nuclei such as the paraventricular nucleus, supraoptic nucleus, arcuate and ventromedial nuclei, as well as in the NTS, area postrema and dorsal motor nucleus of the vagus, hippocampus, olfactory bulb, choroid plexus and cerebellum; and RAMP3 mRNA is expressed in the cerebral cortex, thalamus and cerebellum [95, 100]. These findings suggest the existence of a central adrenomedullinergic system of physiological relevance.
Several studies support that hypertension may influence AM and its receptor component expression both in the periphery and in the CNS. In fact, AM concentration is increased in the plasma and tissues during hypertension [36, 74]. In addition, the expression pattern of RAMPs isoforms in a given cell may change in physiological and pathological conditions determining the responsiveness to AM and CGRP [5]. Hypertension induced by restraint stress reduced prepro-AM mRNA level in the hypothalamic paraventricular nucleus, supraoptic nucleus, NTS, dorsal motor nucleus of the vagus, area postrema and subfornical organ [88]. It has been reported changes in brain mRNA RAMP2 expression in response to blood pressure manipulations. In effect, decreased blood pressure induced by nitroprusside infusion elicited an increase in RAMP2 mRNA expression in the NTS, while increased blood pressure induced by phenylephrine infusion elicited a decrease in RAMP-2 mRNA expression in the paraventricular nucleus and NTS. This data provide anatomical and physiological evidence for a homeostatic role for AM in the brain and suggest that central AM may participate in the regulation of sympathetic activity [95]. Effectively, it has been shown that chronic stress induced by immobilization for seven consecutive days produced an enhanced expression of AM in the cerebrospinal fluid-contacting nucleus [106] and chronic footshock and noise stress for fifteen consecutive days produced an up-regulation of prepro-AM and RAMP3 expression in hypothalamus and pituitary gland [56]. Also, chronic stress caused an increase in CRLR expression in medulla oblongata and decrease in the midbrain and hypothalamus. While RAMP2 expression was increased in medulla oblongata and hypothalamus, suggesting that increased hypothalamic prepro-AM, RAMP2 and RAMP3 may be a protection mechanism for resetting hypertension induced by chronic stress and suggests that AM may be related to the development of the stress-induced hypertension [56].
3 Adrenomedullinergic System in Cerebellum Vermis
The emphasis for a role of the systemic circulating AM in the regulation of fluid and electrolyte balance, arterial pressure and the pathophysiology of cardiovascular disease have recently shift, and now is focused on the local tissue AM. Studies have demonstrated the importance of a tissue AM system. As essential requirement for a tissue AM system is that all of the components necessary for the biosynthesis of the active peptide reside within the tissue. This requires a demonstration that peptides mRNAs, its receptor components and biosynthetic enzymes are in detectable quantities, and that the peptide synthesis actually occurs locally. Although some of the components may be taken up into the tissue from the circulation, even in the presence of a local system, the de novo tissue generation of AM and its interaction with AM receptors on the same (autocrine) or adjacent (paracrine) cells defines the local system. In addition, it should be demonstrated that the biologically active product is regulated within the tissue independently of the systemic circulation, and the reduction or elimination of the action of the product results in a physiological response [9]. In regard to brain and specifically in the cerebellum, there is sufficient evidence indicating the existence of a local functional cerebellar adrenomedullinergic system.
In this respect, it has been demonstrated the presence of AM, AM receptors and their receptor components in cerebellum vermis [24, 30, 75]. AM immunoreactivity, AM binding sites and CRLR, RAMP1, RAMP2 and RAMP3 expression are detected in rat cerebellum [10, 86, 94, 102]. In fact, it has been demonstrated by light and electron microscopy AM-like immunoreactivity in cerebellar Purkinje cells and mossy terminal nerve fibers as well as neurons of the cerebellar nuclei [66, 86, 87]. In addition, the presence of RAMP1 and RAMP2 mRNA in Purkinje cells and RAMP3 mRNA in cerebellar granular cells has been shown [100]. Likewise, CRLR and RAMP1 were detected on the surface of the Purkinje cell bodies and in their processes [19, 65]. This evidence indicates that AM could participate in cerebellar functions as an autocrine/paracrine factor.
Moreover, the evidence suggests that the expression and function of adrenomedullinergic system components could be altered during growth. In fact, RAMP1, RAMP3 and CRLR expression in rat cerebellar vermis increased with age, without changes for AM and RAMP2 expression [24, 30]. Similar findings have been shown for CGRP1 receptor (CRLR/RAMP1) expressed in cerebellar cortical neuron and glial, which suffer of marked changes during cerebellum development. Indeed, autoradiographic and immunofluorescence studies followed by con-focal analysis in rat cerebellum demonstrated a lower CGRP1 receptor density in Purkinje cells and molecular cells during development and their increase during maturity, suggesting that CGRP1 and its receptor may promote a coordinated development of cerebellar glial cells, an effect driven mainly by the CGRP released by climbing fibers [64].
Likewise, AM activates several signaling pathways and regulates ROS metabolism centrally [91, 101, 103]. In cerebellum AM activates extracellular signal-regulated kinases (ERK), increases cAMP production probably through the activation of protein kinase A (PKA); increases cyclic guanosine monophosphate (cGMP) production and nitric oxide (NO) accumulation. These effects are mediated through the activation of AM1 receptor, since AM specific receptor blocker, AM(22-52), blunted AM action, meanwhile AM-induced increase of cAMP production is mediated through stimulation of AM2 and CGRP receptors [26–30] (Fig. 3). In this sense, Endo and Launey [20] found that NO-cGMP-protein kinase G (PKG) pathway plays an important role in the activation of ERK1/2 in Purkinje cells of rat cerebellum. This high levels in the cerebellar NO/cGMP [63], the enzyme guanylil cyclase (GC) [68], cGMP [22] and cGMP-dependent protein kinase in cerebellar Purkinje cells [57] suggest that the NO/cGMP signaling pathway could act as a neurotransmitter in the cerebellum. Futhermore, nitric oxide synthase (NOS) and soluble GC are colocalized and activated in various regions of the cerebellum [16]. In contrast to cAMP, which is homogeneously distributed in different brain areas, cGMP is 10 to 50 times more concentrated in the cerebellum than in other brain areas. cGMP is believed to exert its effects through cGMP-dependent protein kinase (cGPK), the activity and expression is specifically detected in the cerebellum, mainly concentrated in the Purkinje cells [57].
AM has been described as an endogenous antioxidant substance as it is cytoprotective against organ damage [69, 92]. In fact, AM regulate cerebellar metabolism of ROS, since AM decreased thiobarbituric acid reactive substances (TBARS) production, and catalase (CAT), glutathione peroxidase (GPx) and superoxide dismutase (SOD) basal activity in cerebellar vermis of male Sprague Dawley rats [23, 31] as it was also reported in other organs such as kidney and liver [14, 114]. This decrease in enzyme activity in cerebellar vermis suggests that AM is capable of reducing ROS production, because the reduction of antioxidant enzymes activity was accompanied by a decrease in lipid peroxidation. Likewise, this effect is mediated by PKA, since pretreatment with PKI-(6-22) amide, a PKA inhibitor, was able to reverse the inhibitory effect of AM on the basal activity of antioxidant enzymes (Fig. 3), as has been reported by other studies in several tissues [92, 112]. Moreover, AM(22-50) and CGRP(8-37) blunted AM-induced decrease of antioxidant enzymes activity indicating that this effect is mediated through stimulation of both AM and CGRP1, receptors. These data support the role of AM in the regulation of cerebellar antioxidant enzyme activity and suggests the existence of a functional local cerebellar adrenomedullinergic system of physiological relevance.


Fig. 3
Adrenomedullin receptor signaling pathways in rat cerebellar vermis. AM reduces SOD, CAT and GPx enzyme activity mediated by PKA, via activation of CGRP and AM receptor subtypes. Meanwhile, AM increases ERK activity, NO and cGMP production via activation of AM1 receptor; and increases cAMP production through CGRP1 and AM receptor subtypes (AC adenylil cyclase, AM adrenomedullin, ATP adenosine triphosphate, CAT catalase, cAMP cyclic adenosine monophosphate, cGMP cyclic guanosine monophosphate, ERK extracellular signal-regulated kinases, GC guanylil cyclase, GPx glutathione peroxidase, Gs soluble protein G, GTP guanosine triphosphate, NO nitric oxide, NOS nitric oxide synthase, p-ERK phospho-extracellular signal-regulated kinases, PKA protein kinase A, SOD superoxide dismutase)
4 Cerebellar Adrenomedullinergic System During Hypertension
There is evidence that cerebellum participates in blood pressure regulation and of changes of AM and its receptor components expression in several regions of CNS during hypertension. In support for that, in the pioneer work of Pastorello et al. [75] who reported the distribution and levels of AM receptor binding sites in the brain of 16-week-old normotensive Wistar Kyoto (WKY) and spontaneously hypertensive (SHR) rats, using 125I-hAM(13-52) as radioligand. As previously reported by Juaneda et al. [48], it was demonstrated that AM receptors are discretely and differentially distributed in the rat cerebellum cortex. However, higher levels of AM binding sites were detected in the granular cell layer of the cerebellum of SHR when compared with WKY rats [75] (Fig. 4). The increase in cerebellar AM receptors during hypertension could be interpreted as an up-regulation mechanism of cerebellar binding sites to compensate the increase in blood pressure in SHR rats; or they could represent a primary alteration which would result in a secondary alteration in the autonomic regulatory mechanisms in cerebellum with the consequent increase in blood pressure.


Fig. 4
Autoradiographic localization of 125 h-AM(13-52) binding in coronal sections of rat cerebellum. Sections of WKY (Panel A) and SHR (Panel B) rat cerebellum. Quantification of 125h-AM(13-52) binding (Panel C). T Total binding, NS Non-specific binding (Modified and reprinted with permission from Pastorello et al. [75])
Figueira and Israel [30] showed the notion of a dysregulation of AM cerebellar system during hypertension in 16-week-old WKY and SHR rats in the cerebellar vermis. Quantification of AM, CRLR, RAMP1, RAMP2 and RAMP3 expression using western blot analysis, demonstrated an up-regulation of cerebellar vermis CGRP1 (CRLR + RAMP1) and AM2 (CRLR + RAMP3) receptors and a down-regulation of AM1 (CRLR + RAMP2) receptor during hypertension associated with a decreased AM expression (Fig. 5). The reduction in AM expression in vermis of SHR rats could be responsible for the up-regulation of AM2 receptors and binding sites observed by autoradiography [24, 30].


Fig. 5
Effect of hypertension on adrenomedullin and its receptor components in cerebellar vermis. AM expression and of its receptor components CRLR, RAMP1, RAMP2 and RAMP3 are altered in SHR rats when compared with WKY rats. In SHR rats AM and RAMP2 expression are reduced while CRLR, RAMP1 and RAMP3 expression are increased. Cerebellar vermis expression of AM (Panel A), RAMP2 (Panel B), RAMP1 (Panel C), RAMP3 (Panel D) and CRLR (Panel E) in 8 and 16 week-old WKY and SHR rats. Results are expressed as mean ± S.E.M. AM, RAMP1, RAMP2, RAMP3 and CRLR expression was normalized with that of β-actin (N = 10). *p < 0.0001 vs. WKY 8 week old. #p < 0.0001 vs. WKY 16 week old (Modified and reprinted with permission from Figueira and Israel [30])
It is known that CRLR/RAMP2 complex constitutes the pathway for AM biological activity, and it is believed that altered expression of RAMPs is associated with alterations of AM response [36]. Therefore, RAMP2 is mainly expressed in the basal state while expression levels of RAMP3 remain relatively low [36]. In pathological conditions like hypertension, there are phenotype changes of RAMPs expression, from a high response (high expression of RAMP2) to a low response to AM (high expression of RAMP3) [30, 36]. Therefore, the decreased expression of RAMP2 and AM suggest that these changes may constitute a mechanism which contributes to the development of hypertension, and supports the notion that this peptide is involved in the regulation of blood pressure in cerebellum. Furthermore, up-regulation of CRLR, RAMP1 and RAMP3 expression would promote the interaction of AM with CGRP1 and AM2 receptors, rather than AM1 receptors, thereby favoring the compensatory mechanism to increased blood pressure. Alternatively, these changes could be the initial disturbance that would result in dysregulation of the mechanisms controlling blood pressure, since these changes are present from the early stages of life (8 weeks) of hypertensive rats [30]. In fact, lowering blood pressure with an oral antihypertensive treatment during eleven days, reversed AM and its receptor components expression to those levels found in normotensive rats [33], suggesting that hypertension induces adaptive changes to compensate a rise of blood pressure (Fig. 6). Meanwhile, the antihypertensive treatment, to restore AM and RAMP2 expression would increase AM1 receptor expression and would promote AM responsiveness via AM1 receptor. Therefore, reversion with peripheral administration of an antihypertensive may represent a strategy to compensate the primary abnormality in the control of blood pressure and opens the possibility of a novel mechanism in antihypertensive therapy suggesting that cerebellum may play a role in the regulation of arterial blood pressure.


Fig. 6
Effect of lowering blood pressure on AM and its receptor components expression in cerebellar vermis of WKY and SHR rats. AM expression and of its receptor components CRLR, RAMP1, RAMP2 and RAMP3 are altered in SHR rats when compared with WKY rats. In SHR rats, AM and RAMP2 expression are reduced while CRLR, RAMP1 and RAMP3 expression are increased. Oral antihypertensive treatment with valsartan during eleven days, reversed AM and its receptor components expression to those levels found in normotensive rats and reestablished the AM responsiveness (AM adrenomedullin, SHR spontaneously hypertensive rats, WKY Wistar Kyoto rats)
5 Role of Brain Adrenomedullin in Blood Pressure Control
AM is a peptide which exerts important roles in the regulation of cardiovascular function. At peripheral level, AM administration reduces blood pressure due to a decrease in peripheral vascular resistance [5, 38, 40]. It has been demonstrated that AM exerts its vasodilator action by an endothelium-dependent and independent mechanism. In this regard, most studies have indicated that AM induces endothelium-independent vasodilation by activating CGRP receptors with subsequent activation of cAMP production in vascular smooth muscle cells. Also, AM can activate potassium channels in smooth muscle cells causing hyperpolarization [81]. Additionally, AM through their specific receptors on endothelial cells induces endothelium-dependent vasodilation [39], since AM activates endothelial NOS (eNOS) by at least two mechanisms. First, AM increases intracellular calcium levels, which stimulates eNOS activity [91], and secondly, AM activates phosphatidylinositide 3-kinase/Akt (PIK3/Akt) pathway, which phosphorylates eNOS and increases the enzyme activity even at low calcium concentrations [70]. In fact, Hayakawa et al. [39] found that AM relaxed rat aorta, pre-contracted with phenylephrine, in a dose-dependent manner, observing that endothelium denudation attenuated vasodilator response, suggesting that NO-cGMP signaling pathway is involved in the mechanism of AM-induced vasorelaxation in aorta. Furthermore, AM is capable of inhibiting the production of vasoconstrictors such as endothelin-1 [5].
AM effects at the CNS cannot be predicted based on the effects induced by this peptide peripherally [38]. Indeed, AM exerts several effects in the CNS. Central AM administration results in various neuroendocrine responses such as the inhibition of arginine vasopressin secretion induced by hypovolemic and osmotic stimuli, and an increase of oxytocin secretion by activating hypothalamic oxytocin-producing cells [85, 111]; likewise intracerebroventricular (icv) administration of AM elevated plasma adrenocorticotropic hormone (ACTH) levels [89]. In addition, central administration of AM inhibits water drinking and salt appetite [99] and increases urinary water, sodium and potassium excretion, in a dose-dependent manner in conscious hydrated rats [17, 45]. Central administration of AM increases atrial natriuretic peptide release [12, 98]. Furthermore, microinjection of AM into the area postrema and the rostral ventrolateral medulla causes a hypertensive effect; while in the paraventricular nucleus of the hypothalamus produces hypotension [1, 46, 108]. This evidence indicates that central AM may play an important role in body fluid homeostasis and central regulation of the cardiovascular system.
In this regard, administration of AM into the area postrema [1, 110] or icv caused an increase in blood pressure in a dose-dependent manner [45, 83, 84], an effect mediated through CGRP receptor [80, 96]. While, Samson [84] suggested that the central AM pressor action is mediated through specific AM receptors, since administration of AM in the lateral and fourth ventricles elevates blood pressure in conscious rats, and this effect was not blocked by the antagonist of CGRP receptor. Furthermore, Xu and Krukoff [107] showed that AM administration into the rostral ventrolateral medulla increased blood pressure and heart rate in dose-dependent manner, through AM specific receptors and mediated by glutamate involving N-methyl-D-aspartate (NMDA) and non-NMDA receptors; additionally NO synthetized from neuronal NOS (nNOS) contributes with the vasopressor effect induced by AM through a signaling pathway associated with soluble GC. This data indicate that AM in the rostral ventrolateral medulla causes a hypertensive effect potentiating glutamatergic and nitrergic neurotransmission, since both glutamatergic and nitrergic inhibition blunted AM induced effects in the rostral ventrolateral medulla [107].
On the other hand, AM administration in the paraventricular nucleus has been shown to cause a decrease in blood pressure [93] which is mediated by NO and gamma-aminobutyric acid (GABA), since blockade of GABAA receptor and inhibition of NO production attenuated the decrease in blood pressure induced by AM. These results suggest that AM in the paraventricular nucleus activates cells producing NO, increasing GABAergic neurotransmission and lowering blood pressure [107]. Furthermore, it has been shown that icv administration of AM stimulates NO production in the hypothalamus and activates neurons which produce NO in the paraventricular nucleus [89]. Likewise, microinjection of sodium nitroprusside, a NO donor, in the paraventricular nucleus lowers blood pressure and heart rate [116], suggesting that NO in this nucleus is one of the candidates mediating the hypertensive effect of AM. In addition, GABA exerts a tonic inhibitory effect on sympathetic nervous system in paraventricular nucleus [13, 59]; in fact, the selective antagonist of GABAA receptor, bicuculline, blocked AM-induced hyperpolarization of paraventricular nucleus magnocellular neurons, suggesting that GABA can contribute to AM hypotensive effect within the paraventricular nucleus [34].
6 The Cerebellum as a Cardiovascular Regulator
The physiological mechanisms involved in AM actions in cerebellum are elusive and not yet clarified and could be multiple and complex. Moreover, there is little information about the role of the cerebellum in cardiovascular regulation.
There is anatomical evidence of a possible role for cerebellum in the regulation of cardiovascular function obtained from animal models and humans which show that vestibular cerebellar system and their cerebellar connections contribute to cardiovascular control during movement and posture alteration [15]. Various brain regions involved in cardiovascular control receive nerve signals from the cerebellum specifically from the fastigial nucleus and posterior cerebellar cortex [6–8, 18]. Furthermore, anatomical evidence supports the role of fastigial nucleus in cardiovascular function. Indeed, it has been identified various cardiovascular modules, such as fastigial nucleus, anterior vermis, posterior vermis, uvula (lobe IX), nodulus (lobe X); because stimulation of these structures involves changes in blood pressure, respiratory rate and vascular resistance [71]. In effect, after integrating the current research in the role of cardiovascular regulation of the cerebellum, Nisimaru [71] proposed the existence of five cardiovascular modules in the cerebellum dedicated to cardiovascular control. The first, a discrete rostral portion of the fastigial nucleus and the overlying medial portion of the anterior vermis (lobules I, II and III), this module controls the baroreflex. The second is formed by the anterior vermis which constitutes a microcomplex with parabrachial nucleus. The third is formed by caudal fastigial nucleus and posterior portion of vermis (lobes VII and VIII), controlling the vestibule sympathetic reflex. The fourth is integrated by the medial portion of the uvula with the NTS and parabrachial nucleus. The fifth module includes the lateral edge of the nodulus, and the uvula together with parabrachial nucleus and vestibular nuclei, which constitutes a cardiovascular microcomplex that controls the magnitude and/or timing of sympathetic nerve responses and stability of the mean arterial blood pressure during changes of head position and body posture. The lateral nodulus-uvula appears to be an integrative cardiovascular control center involving both the baroreflex and the vestibule-sympathetic reflex (Fig. 7) [71].
The major functions of these multiple microcomplexes are considered to be adaptative controls of the vestibule-sympathetic reflex and the baroreflex. This system combines feedback and feedforward control systems. The feedforward pathway from vestibular labyrinth to the vestibular nucleus (VN) and the feedback pathway from baroreceptors to the NTS and parabrachial nucleus converge on the common controller part in the rostral ventrolateral medulla. This combined control system maintains the mean arterial blood pressure against head movements and any perturbation of blood pressure under adaptative control by the five cardiovascular microcomplexes [71].


Fig. 7
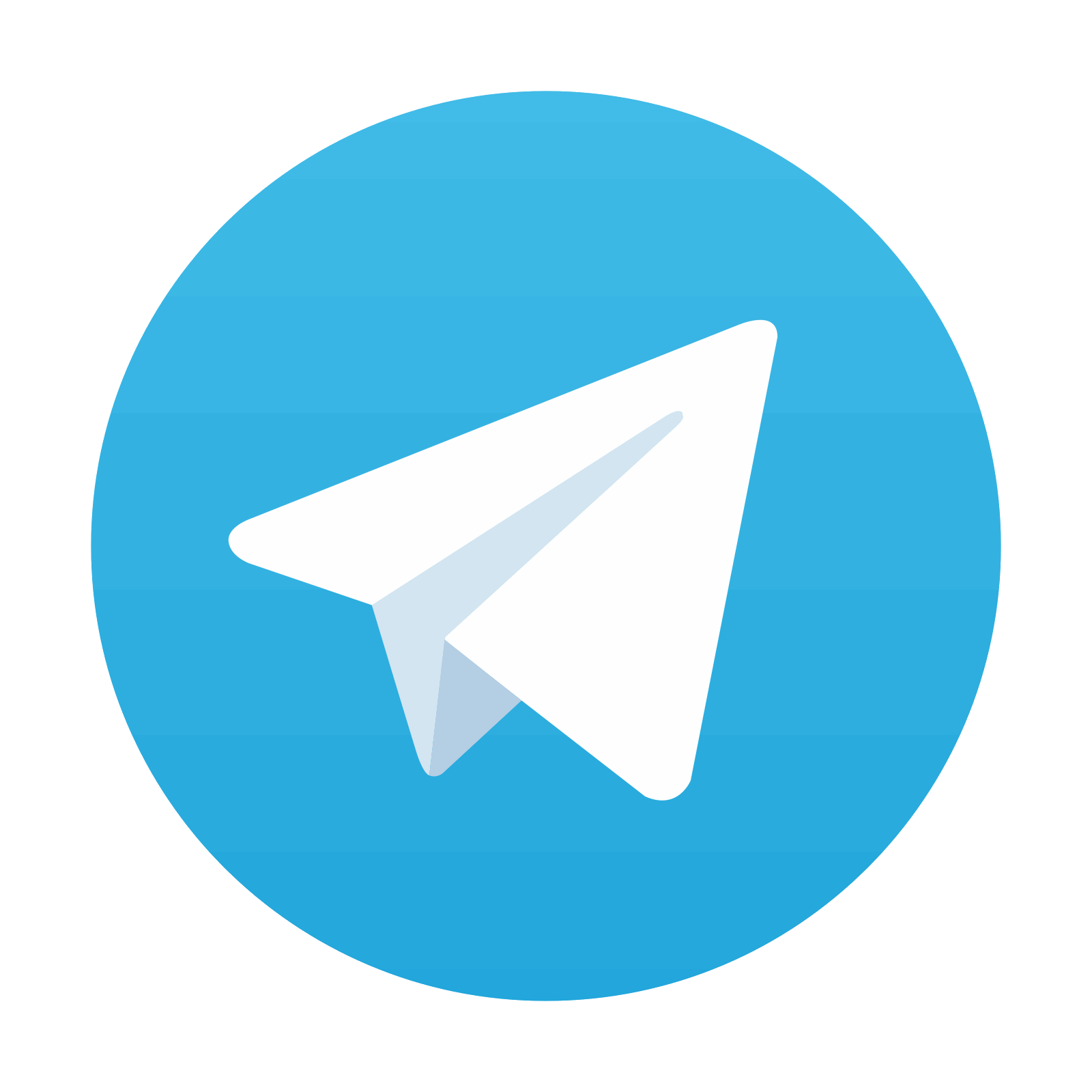
Cerebellar cardiovascular modules. PBN Parabraquial nucleus, NTS Nucleus of tratus solitarii, VN Vestibular nucleus [71]. Studies in different animal species have shown that electrical stimulation of fastigial nucleus produces a pronounced pressor response, which may be due to connections of this nuclei with other important and recognized cardiovascular modules [7, 8, 71]. Meanwhile, electric stimulation of anterior vermis, the medial cortical regions of lobules I, II and III, posterior vermis (lobules VII and VII) and uvula (lobule IX) induces bradycardia, blood pressure fall and transient inhibition of sympathetic renal activity [3, 7, 71, 79]
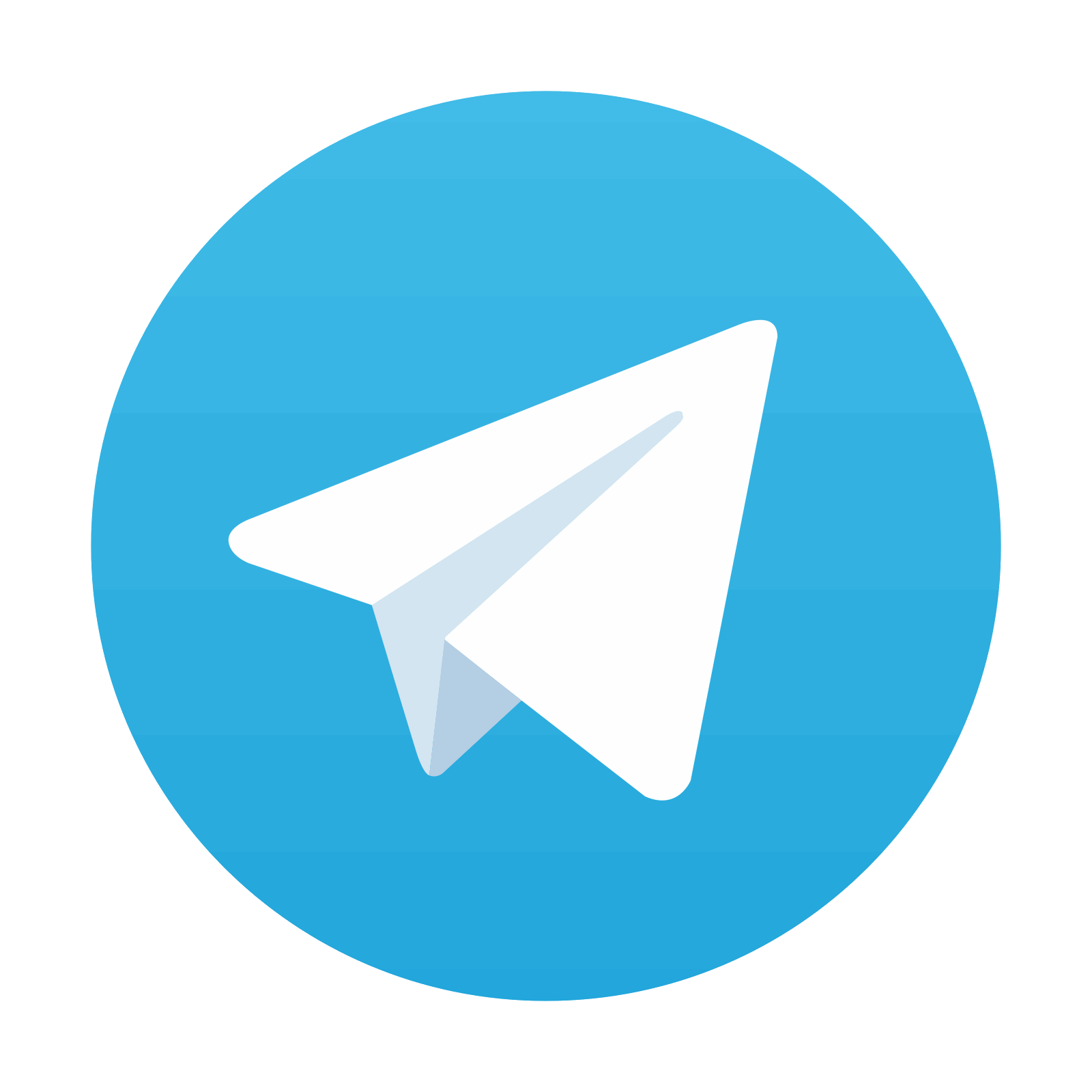
Stay updated, free articles. Join our Telegram channel

Full access? Get Clinical Tree
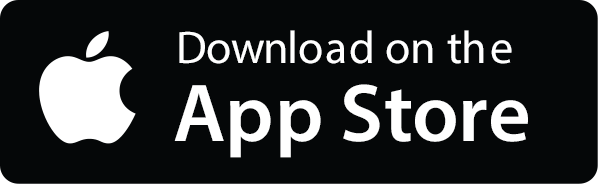
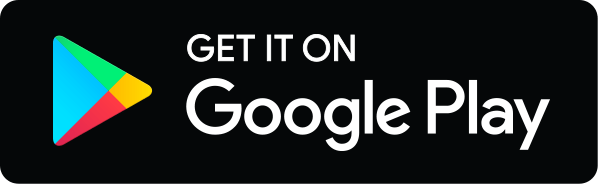
