The limitations inherent to angiography served as a technological springboard for the development of other devices in search of better visualization, quantification, and identification of the coronary vasculature and assessment of the efficacy of subsequent clinical therapeutics. Intravascular ultrasound (IVUS) was invented and introduced to clinical practice in the late 1980s as an invasive intravascular imaging device. As a front-runner in this field, IVUS has greatly contributed to the investigation of the pathophysiology of coronary artery disease in symptomatic patients and to the guidance of percutaneous coronary interventions (PCI).
Since the early 2000s, optical coherence tomography (OCT), which was first introduced in the ophthalmology field, has been applied to human intravascular imaging. Apart from these intravascular imaging systems, spectroscopy has also begun to gather attention as a device to determine plaque components. Spectroscopy provides adjunctive information when used with IVUS, though current clinical applications are limited to the detection of cholesterol within the vessel wall.
This chapter will provide information related to concrete usage and tips of adjunctive intravascular imaging devices as well as to review evidence and rationale for use as a procedural guide in the cardiac interventional setting.
After the first initial clinical experience by Yock et al. in 1988,1 IVUS has become established as an adjunctive diagnostic device as well as an essential research tool in the cardiac catheterization laboratory. Moreover, its development and findings have been a driving force in the expansion of the intravascular diagnostic field. With a miniaturized ultrasound probe equipped at a tip of an IVUS catheter, a high-frequency ultrasound beam ranging from 20 to 45 megahertz (MHz) is transmitted radially from the probe to a vessel wall and its reflection sound waves are reconstructed to depict a cross-sectional image. The higher frequency ultrasound beam (shorter wave length) than those utilized in noninvasive echocardiography (2-5 MHz, longer wave length) potentiates its high resolution to visualize vessel and intravascular structures, which leads to its low radial penetration of 4 to 8 mm from the IVUS catheter. To improve the resolution of IVUS images, adoption of ultrasound with higher frequency (55-60 MHz) than conventional IVUS is currently underway; this will possibly lead to better interpretation of gray-scale IVUS imaging by improving tissue and plaque differentiation.
There are several intermediate resolution/penetration ultrasound catheter devices for peripheral (15-20 MHz) or intracardiac (intracardiac echocardiography [ICE], 5-10 MHz) uses, specifically developed to guide catheter-based treatments of peripheral vessels and structural heart disease, or catheter ablation. Detailed descriptions of ICE can be found elsewhere in this textbook (see Chapter 13).
IVUS imaging systems consist of 3 components: an imaging catheter, a console for imaging reconstruction/display/recording/analysis, and a catheter interface unit with motorized transducer pullback capability. Specifically, 2 types of imaging acquisition processes are applied to commercially available IVUS catheters: a solid-state dynamic aperture and a mechanically rotating single transducer system (Table 25-1). Currently, only 1 company (Volcano Corp., San Diego, CA) provides the solid-state catheter system that has 64 phased-array transducers mounted around the catheter tip. It utilizes a rapid-exchange design and the guidewire is inserted through a circumferential array of transducer elements. On the other hand, mechanical catheter systems are available from 4 companies with slightly varying configurations (Boston Scientific Corp., Marlborough, MA; Infraredx Inc., Burlington, MA; Terumo Corp., Tokyo, Japan; and Volcano Corp./Royal Philips). In mechanical systems, there is only one transducer equipped at the tip of the catheter, which rotates inside of a protective sheath, and it incorporates a rapid-exchange design with the guidewire running alongside the imaging window; therefore, this system can have several inherent artifacts from its structure (eg, non-uniform rotational distortion, guidewire shadow). In general, however, image quality is superior to the solid-state system because of the higher frequency ultrasound (40-45 MHz vs 20 MHz) and the larger effective aperture of a transducer element used in the mechanical system. In addition, longitudinal lesion length assessment is more accurate in the mechanical system, because the transducer pullback is performed within the stationary protective sheath.
Mechanically Rotating Single-Transducer System | Solid-State Dynamic Aperture System | ||||
---|---|---|---|---|---|
Features | Higher image resolution Protective sheath (air flushing requirement) Better longitudinal length assessment | Shorter preparation time Digital subtraction requirement to mask ring-down artifact Shorter length from tip to imaging core | |||
Artifacts | Air bubbles Guidewire artifact Non-uniform rotational distortion (NURD) | Ring-down artifact | |||
Manufacturer | Boston Scientific | Infraredx | Terumo | Volcano | Volcano |
Console | iLabTM | TVC CompositeTM | VISIWAVE® | s5TM | s5TM |
Catheter | OptiCrossTM | TVC InsightTM | ViewIT® Intrafocus® WR | Revolution | Eagle Eye® Platinum Eagle Eye® Platinum ST |
Frequency | 40 MHz | 40 MHz | 40 MHz | 45 MHz | 20 MHz |
Guide compatibility | 5 F | 6 F | 5 F | 6 F | 5 F |
Tissue or plaque characterization | iMapTM | near-infrared spectroscopy | integrated backscatter | N/A | ChromaFlo® Virtual HistologyTM |
In terms of preparing the IVUS catheter prior to use, there are differences between the mechanical system and the solid-state system owing to their designs. The mechanical system requires a saline flush to remove air bubbles between the protective sheath and the transducer before IVUS catheter insertion, whereas the solid-state system requires a step to mask ring-down artifact before insertion of IVUS catheter into the coronary artery, which is usually performed in the aorta or in the left main. It is generally recommended that residual air bubbles should be flushed outside of the body so as to avoid an air embolization. Similar to standard interventional procedures, intravenous heparin (5000-10,000 units) and intracoronary nitroglycerin (100-200 micrograms) should be administered beforehand. Thereafter, a 0.014-inch guidewire is advanced distally to the target vessel, followed by IVUS catheter insertion. Pullback IVUS scan should be started from at least 10 mm distally to the target lesion and to the aorta for complete target vessel interrogation. Automated pullback speeds of 0.5 mm/sec and 1.0 mm/sec are available. When assessing an ostial lesion, it is important to disengage the guide catheter slightly from the ostium so that the target lesion can be fully visualized.
Differentiating among the types of artifacts and addressing them properly are important aspects to achieve precise interpretation of IVUS images. Artifacts of IVUS mainly derive from IVUS design, feature of ultrasound, or inappropriate preparations (Fig. 25-1).
FIGURE 25-1
Examples of common IVUS artifacts. A. Acoustic shadowing is an image blank behind strong ultrasound reflectors. B. Air bubbles are the artifact that blurs an IVUS image, when residual air bubbles exist between an outer sheath and a transducer in mechanical IVUS systems. C. Blood speckles can be observed as an unexpectedly high-echoic area within a lumen, and, as a result, the border between lumen and intima may become obscure. D. Multiple ghost images called reverberations (white arrowheads), spaced radially at regular intervals, can be observed in a calcified lesion with a smooth surface (red arrowhead) or a lesion with a metal stent. E. Non-uniform rotational distortion (NURD) is an artifact that can be seen as a partial or entire distortion of the cross-sectional image, resulting from mechanical binding of the drive cable that rotates the transducer inside the protective sheath. F. Ring-down artifact can be observed as a ring adjacent to an IVUS catheter and may be caused by residual air bubbles within the protective sheath or the sheath itself in mechanical IVUS systems, or by interference of ultrasound in the solid-state system. G. Side lobe artifact is an artifact observed laterally to stent struts or a calcification. When a strong reflector exists, a side lobe ultrasound can create a lateral ghost image. H. A guidewire can be the cause of acoustic shadowing (see Part A) called guidewire artifact, stemming from the reflection of the ultrasound beam by the guidewire.

Acoustic shadowing (see Fig. 25-1A). Acoustic shadowing is an image blank behind a calcification, a metal stent, or a guidewire. IVUS beam cannot penetrate such strong ultrasound reflectors, generating the image blank observed behind these structures.
Air bubbles (see Fig. 25-1B). Air bubbles are the artifact that blurs an IVUS image. When residual air bubbles exist between an outer sheath and a transducer in mechanical IVUS systems, adequate ultrasound beam emission and image acquisition are inhibited. Repeat saline flushes are necessary to fully remove the residual bubbles.
Blood speckles (see Fig. 25-1C). Blood speckles can be observed as an unexpectedly high-echoic area within a lumen, and, as a result, the border between lumen and intima may become obscure. It is usually observed when using an ultrasound system with higher frequency (>40 MHz), or in lesions with stagnant coronary flow from severe stenosis where rouleaux formation of red blood cells occurs. An injection of saline or contrast will help to identify the lumen border.
Multiple reverberations (see Fig. 25-1D). When analyzing a calcified lesion with smooth surface or a lesion with a metal stent, multiple ghost images, spaced at radially regular intervals, can be observed. These ghost images are called multiple reverberations, and care should be taken to not misinterpret these findings as true structures. This phenomenon is caused by multiple reflections of the ultrasound beam between the transducer and a strong reflector.
Non-uniform rotational distortion (NURD) (see Fig. 25-1E). NURD is an artifact that can be seen as a partial or entire distortion of the cross-sectional image. This artifact is unique to the mechanical IVUS systems, resulting from mechanical binding of the drive cable that rotates the transducer inside the protective sheath. This is usually observed in a tortuous artery or from a kink in the imaging catheter. To avoid this artifact, it is important to straighten the IVUS catheter outside of body, or to unfasten the Y-connector to release torque.
Ring-down artifact (see Fig. 25-1F). Ring-down artifact can be observed as a ring adjacent to an IVUS catheter and may be caused by residual air bubbles within the protective sheath or the sheath itself in mechanical IVUS systems, or by interference of ultrasound in the solid-state system. This may be treated with additional saline flushing in mechanical systems, or digital mask adjustment in the solid-state system.
Side lobe artifact (see Fig. 25-1G). Side lobe artifact is an artifact observed laterally to stent struts or a calcification, which can possibly lead to an inaccurate assessment of a stent area because of pseudo-protruding struts to the lumen. When a strong reflector exists, a side lobe ultrasound can create a lateral ghost image.
H) Guidewire artifact (see Fig. 25-1H). A guidewire can be the cause of acoustic shadowing (see Fig. 25-1A), stemming from the reflection of the ultrasound beam by the guidewire, resulting in the blank image behind it. This artifact is only seen in the mechanical IVUS system, because a guidewire runs alongside the transducer.
Arterial three-layer structures (intima, media, and adventitia) correspond to high-low-high echoic findings by IVUS (Fig. 25-2).2 It is usually difficult to distinguish adventitia from extravascular matrix. In some cases, the media may appear artifactually thin because of “blooming”—an intense reflection from the intima. In other cases, the media can contrarily appear thick because of signal attenuation from the intima and the weak reflectivity of the internal elastic membrane (IEM). Particularly in younger normal subjects, the vessel wall may have a single-layer appearance, since the intima is thinner than the resolution of IVUS.3 IEM and external elastic membrane (EEM), just adjacent to the media, cannot be observed by IVUS. In some cases, the media may be destroyed partially in association with development of atherosclerosis, and it no longer appears as a distinct layer.
FIGURE 25-2
Typical cross-sectional IVUS image and its interpretation. A. A cross-sectional IVUS image with schematic diagram. B. Demonstrates the classic high-low-high, three-layered appearance. C. Histologic correlations with intima, media, and adventitia. The media has lower ultrasound reflectance owing to less collagen and elastin as compared with the neighboring layers. Because the intimal layer reflects ultrasound more strongly than does the media, there is a spillover in the image, which results in a slight overestimation of the thickness of the intima and a corresponding underestimation of the medial thickness.

In order to determine image orientation within the artery, recognition of perivascular structures (eg, branches, veins, pericardium, and myocardium) are of clinical importance, especially during interventional procedures or in repeated recordings over time (Fig. 25-3).4 An IVUS image is reconstructed as a cross-sectional image as if the interpreters look down into a coronary artery in a proximal-to-distal direction. As an example in a typical case, a diagonal branch is located at approximately 90° to 120° clockwise to a septal branch. In contrast to side branches, veins are susceptible to compression during the cardiac cycle and do not merge with arteries when assessed longitudinally. While the pericardium appears to be a high echoic thick layer with pericardial space between an interrogated vessel and pericardium, the myocardium is viewed on an opposite side of the vessel as a low echoic homogeneous structure.
FIGURE 25-3
Perivascular structures. A. Diagonal and septal branch can be observed in the left anterior descending artery. A diagonal branch is located at approximately 90°-120° clockwise to a septal branch. B. While the pericardium appears to be a high echoic thick layer with pericardial space between an interrogated vessel and pericardium, the myocardium is viewed on an opposite side of the vessel as a low echoic homogeneous structure. C. Right ventricular (RV) vein overlying the right coronary artery. D. Great cardiac vein (GCV) observed from the left circumflex artery. In contrast to side branches, veins are susceptible to compression during the cardiac cycle and do not merge with arteries when assessed longitudinally.

With an intrinsic distance calibration, diameter and cross-sectional area (CSA) can be measured in a cross-sectional IVUS image. Measurements will be repeated in the tightest cross-section within the lesion, and in proximal and distal reference segments. Reference segments are usually selected as the most “normal-looking” cross-section within 10 mm from the interrogated lesion without any major intervening side branches. The average area of proximal and distal reference is often used. Vascular remodeling, originally identified and proposed in autopsy specimens, can also be evaluated by IVUS. When serial IVUS images are available, vascular remodeling can be defined as a ratio of follow-up EEM CSA to baseline EEM CSA. When it is not available, it can be defined using a following formula: Remodeling index=lesion EEM CSA/reference EEM CSA. If the lesion EEM CSA exceeds the reference EEM CSA, remodeling index becomes >1.0, which is defined as positive remodeling of the vessel. On the other hand, negative remodeling is defined as a remodeling index <1.0. Instead of this cutoff, positive remodeling of >1.05 and negative remodeling of <0.95 can be employed.5
After determination of the interface between the lumen and the leading edge of intima, lumen measurements can be performed. In addition to lumen CSA, minimum, maximum, and mean diameters as well as eccentricity can be obtained. Because the interface between the adventitia and extravascular matrix is usually obscure, EEM area is used as an alternative to vessel area. EEM area is defined as the area enclosed by the outermost interface between media and adventitia. Circumferential EEM may not be measured precisely, especially at a bifurcation or calcified lesion. Because IEM is not well delineated by IVUS, the area between the leading edge of the lumen and EEM is defined as atheroma, based on the fact that the media represents only a very small fraction of the atheroma CSA. Accordingly, the term “plaque plus media” is used to represent plaque in a cross-section. In terms of stent measurements, metallic stent struts are strong reflectors of ultrasound, and thus, high gain setting should be avoided to reduce side-lobe artifacts. Typical IVUS measurements for lumen, plaque, and stent are summarized in Figure 25-4 and Table 25-2.
FIGURE 25-4
Examples of quantitative IVUS assessment. Quantitative IVUS measurements for non-stented (A-D) and stented (E-H) segments. Area measurements are performed with computer planimetry. External elastic membrane (EEM) area is defined as the area enclosed by the outermost interface between media and adventitia (B), while lumen area (C and G) is determined by tracing the leading edge of the blood/intima border. Plaque + media area (D) is calculated as the difference between EEM and lumen areas. Stent area (F) is measured by tracing the leading edge of the stent struts, and neointimal area (H) is calculated as the difference between stent and lumen areas. For EEM, lumen, and stent, the maximum (solid arrows) and minimum (dotted arrows) diameters are determined. For plaque + media and neointima, the maximum (solid arrows) and minimum (dotted arrows) thickness are measured.

Lumen Measurements | Plaque Measurements | Stent Measurements | |
---|---|---|---|
Cross-sectional area (CSA) | Lumen CSA: The area bounded by the intimal border. | Plaque plus media (or atheroma) CSA: EEM CSA – lumen CSA. | Stent CSA: The area bounded by the stent diameter. |
Diameter measurement | Minimum or maximum lumen diameter: The shortest or longest diameter through the center point of the lumen. | Minimum or maximum plaque plus media (or atheroma) thickness: The shortest or longest distance from the intimal leading edge to the EEM along any line passing through the center of the lumen. | Minimum or maximum stent diameter: The shortest or longest diameter through the center point of the stent. |
Eccentricity and Symmetry | Lumen eccentricity: (Maximum – minimum lumen diameter)/maximum lumen diameter. | Plaque plus media (or atheroma eccentricity): (Maximum – minimum plaque plus media thickness)/maximum plaque plus media thickness. | Stent symmetry: (Maximum – minimum stent diameter)/maximum stent diameter. |
Other measurements | Lumen area stenosis: (Reference lumen CSA – minimum lumen CSA)/reference lumen CSA. The reference segment used for the formula should be specified (proximal, distal, or average). | Plaque (or atheroma) burden: Plaque + media CSA/EEM CSA. | Neointimal CSA: Stent CSA – lumen CSA. Stent expansion: The minimum stent CSA compared with the predefined reference lumen CSA, which can be the proximal, distal, largest, or average reference lumen CSA. |
In addition to cross-sectional assessments, longitudinal lesion length is an important aspect of a quantitative assessment. In mechanical IVUS systems, longitudinal lesion length can be measured automatically or manually by pulling back a transducer inside the protective sheath, whereas the solid-state system requires the pullback of the catheter itself. Care should be taken in assessing a tortuous lesion, where constant transducer pullback is not warranted. In addition, lesion length can be affected by the longitudinal catheter movement due to heartbeats. With the Eagle Eye® Platinum solid-state catheters (Volcano Corp.), 3 radiopaque markers at 1-cm intervals on the catheter shaft proximal to the transducer may be utilized to estimate the lesion length based on the angiogram.
With conventional gray-scale IVUS, plaques are typically classified into 4 types: (1) soft plaque; (2) fibrous plaque; (3) calcified plaque; and (4) mixed plaque. However, this classification is fundamentally different from the histology, thus necessitates careful interpretation. Soft (echolucent) plaque refers to a low echoic plaque (lower than the adventitia) which derives from high lipid content and contrary low collagen and elastin (Fig. 25-5A). Fibrous plaque refers to an intermediate echoic plaque (echogenicity between the soft plaque and the calcified plaque) and represents the majority of atherosclerotic lesions (Fig. 25-5B). Usually, echogenicity increases according to the increment of fibrous tissue content. Calcified plaque has higher echogenicity than the adventitia and is accompanied by acoustic shadowing (Fig. 25-5C). Calcified plaque can be classified based on depth and its circumferential extent. Depending on the location of the leading edge of calcification, calcified plaque is categorized as superficial (within 50% of plaque plus media) or deep (deeper than 50% of plaque plus media). Because IVUS may not accurately delineate the border of calcification, and the angle is dependent of the distance from the center of the lumen, the extent of calcium may be semi-quantitatively expressed as 4 quadrants; <90°, 90° to 180°, 180° to 270°, and >270°. If the extent of calcium is more than 90°, vessel contour should not be assessed. Calcification less than 90° is called spotty calcification, which is recognized as a feature of unstable plaque in acute coronary syndrome. It is worth noting that highly dense fibrous plaques may have acoustic shadowing and similar echogenicity with calcified plaques due to the high content of fibrous tissue. Some plaques consisting of multiple components are called “mixed plaques” (Fig. 25-5D), and are classified as fibrocalcific or fibrofatty plaques.
FIGURE 25-5
Examples of qualitative plaque characteristics assessment by gray-scale IVUS. With conventional gray-scale IVUS, plaques are typically classified into 4 types: A. soft plaque; B. fibrous plaque; C. calcified plaque; and D. mixed plaque. Soft (echolucent) plaque refers to a low echoic plaque (lower than the adventitia) which derives from high lipid content and contrary low collagen and elastin. Fibrous plaque refers to an intermediate echoic plaque (echogenicity between the soft plaque and the calcified plaque) and represents the majority of atherosclerotic lesions. Calcified plaque has higher echogenicity than the adventitia and is accompanied by acoustic shadowing. Calcified plaque can be classified based on depth and its circumferential extent. Depending on the location of the leading edge of calcification, calcified plaque is categorized as superficial (within 50% of plaque plus media) or deep (deeper than 50% of plaque plus media).

As IVUS-guided intervention matures, there is growing attention to acquiring more detailed plaque characterization. To date, 3 different tissue characterization techniques, which employ computer-assisted analysis of raw radiofrequency (RF) signals in the reflected ultrasound beam, are commercially available: Virtual HistologyTM (VH) (Volcano Corp.), iMAPTM (Boston Scientific Corp.), and Integrated Backscatter (Terumo Corp.). A comparison of the 3 tissue characterization techniques and representative images are shown in Table 25-3 and Figure 25-6.
FIGURE 25-6
Examples of advanced IVUS tissue characterization. To date, 3 different tissue characterization techniques, which employ computer-assisted analysis of raw radiofrequency (RF) signals in the reflected ultrasound beam, are commercially available: A. Virtual HistologyTM (VH) (Volcano Corp.); B. iMAPTM (Boston Scientific Corp.); and C. Integrated Backscatter (Terumo Corp.).

Virtual HistologyTM (VH) | iMAPTM | Integrated Backscatter (IB) | |
---|---|---|---|
Manufacturer | Volcano | Boston Scientific | Terumo |
Console | s5TM | iLabTM | VISIWAVETM |
Catheter | Eagle Eye® Platinum (20 MHz) | OptiCrossTM (40 MHz) | ViewITTM (40 MHz) |
Tissue classification Method | Classification based on a dedicated spectral radiofrequency analysis tree algorithm using multiple radiofrequency signal characteristics developed from ex vivo coronary datasets | Classification based on the degree of spectral similarity between the backscattered signal and a reference library of spectra from preserved histological data | Classification based upon integrated backscatter values, calculated as the average power of the backscattered ultrasound signal from a sample tissue volume |
Color-mapped presentation | Fibrous (green) Fibrofatty (yellow) Necrotic core (red) Dense-calcium (white) | Fibrotic (green) Lipidic (yellow) Necrotic (red) Calcified (blue) | Fibrous (green) Mixed lesion (yellow) Lipid (blue) Calcification (red) |
Features | No guidewire artifact Low resolution | Guidewire artifact High resolution | Guidewire artifact High resolution |
Clinical applications of tissue characterization techniques have been mainly focused on the prediction of future coronary events or slow-flow phenomenon after an interventional procedure by stratifying high-risk coronary plaques. In particular, among the three techniques, the VH-IVUS system has demonstrated the ability to predict future coronary events in a large-scale prospective multicenter trial (Providing Regional Observations to Study Predictors of Events in the Coronary Tree: PROSPECT) where 697 patients with acute coronary syndrome underwent three-vessel gray-scale and VH-IVUS after PCI. The 3-year cumulative rate of major adverse cardiac events was 20.4%, with nearly half of these events being associated with nonculprit lesions, most of which appeared to be mild by baseline angiography. On multivariate analysis, nonculprit lesions with recurrent events were significantly associated with 3 characteristics: (1) plaque burden of 70% or greater; (2) minimal luminal area of 4.0 mm2 or less; and (3) VH-determined thin-cap fibroatheroma.6 In another study of investigating 190 consecutive patients with acute coronary syndrome (ACS), a no-reflow phenomenon was observed in 24 patients after stent implantation. VH-detected absolute and % necrotic core (NC) areas at the minimum lumen area (MLA) site as well as volumetric parameters of these variables were significantly greater in patients with no reflow. In the multivariable analysis, % NC volume was the only independent predictor of no reflow (OR=1.126, 95% CI: 1.045-1.214, P = 0.002).7
There are several inherent limitations to these tissue characterization techniques: limited spatial resolution (100-250 μm) for detection of thin fibrous caps, no classification for thrombus or stent struts, and misclassification of plaque beyond severe calcification due to limited ultrasound signal penetration.
IVUS-guided PCI with both bare metal stents (BMS) and drug-eluting stents (DES) has demonstrated to be associated with better long-term outcomes compared to angiography-guided PCI. In the literature-based meta-analysis including nine studies, Casella et al. showed that IVUS-guided PCI with BMS reduced target lesion revascularization, major adverse cardiac events, and binary restenosis at 6 months, although deaths and nonfatal MI was similar between the two groups.8 In the recently published meta-analysis of IVUS-guided DES implantation including three randomized trials and 12 observational studies with 24,849 patients, Jang et al. found significant reduction of all-cause mortality (odds ratio [OR]=0.64, 95% confidence interval [CI] 0.51-0.81, P < 0.001), major adverse cardiac events (OR=0.79, 95% CI 0.69-0.91, P = 0.001), and stent thrombosis (OR=0.59, 95% CI 0.42-0.82, P = 0.002).9 It must be emphasized that careful and accurate interpretation of IVUS images is mandatory to maximize the benefit of IVUS-guided intervention. One additional aspect of IVUS-guided PCI is the potential to minimize the amount of contrast media during the procedure, which may also impact short- and/or long-term outcomes of our patients.
In terms of preinterventional assessment, IVUS enables the interventionalist to determine the optimal device, its size, and other observations related to predicting favorable outcomes for a given procedure (Fig. 25-7). For example, if the IVUS catheter does not pass the target lesion, stent delivery and deployment may be affected. In this case, lesion preparation with balloon pre-dilatation or rotational ablation with the Rotablator® (Boston Scientific, Marlborough, MA) may be utilized, depending on the angiographic findings. After passing the target lesion, both qualitative and quantitative lesion morphology should be assessed. Additionally, when examining a tortuous artery, a pseudo-stenosis caused by the accordion phenomenon should be kept to avert a misdiagnosis of a stenosis. On the IVUS image, a crescent-shaped lesion can be observed due to the folding of vessel wall. After removal of the IVUS catheter and guidewire, the lesion should be re-evaluated with an angiogram.
Common preinterventional qualitative assessment includes 1) high-risk features for distal embolization, 2) calcified plaque, and 3) plaque morphology with high risk of side branch occlusion. It is reported that plaque volume, a lipid pool-like image, ultrasound attenuation, and thrombus detected by IVUS are thought to be contributing factors to slow flow and no reflow. A lipid pool may be observed as an echolucent (or low echoic) zone inside the plaque in cases with high-quality images. However, detection of a lipid pool only by gray-scale IVUS imaging has low accuracy. Echo-attenuated plaque can be identified by absence of the ultrasound signal behind the plaque that was either hypoechoic or isoechoic to the adventitia, despite absence of calcium10, and is usually found in patients with acute coronary syndrome (Fig. 25-8A). One group recently reported that histopathologically, 91.4% of echo-attenuated plaques corresponded to either a fibroatheroma (FA) with an NC or pathological intimal thickening with a lipid pool; almost all segments with superficial echo attenuation indicated the presence of an FA with an advanced NC.10 Another group proposed that ultrasound attenuation with a longitudinal length of >5 mm and plaque rupture were significantly associated with no-reflow phenomenon.11 IVUS findings for a thrombus vary depending on the morphology; however, it is described as an intraluminal mass with a layered, lobulated, or pedunculated appearance (Fig. 25-8B). Adjunctive intracoronary drug administration or using a distal protection device may be warranted in this setting. In treating the heavily calcified lesion, especially circumferential calcification, called “napkin ring appearance” (Fig. 25-8C), application of the Rotablator® device may be considered as an adjunctive therapy. The IVUS catheter position within a cross section will be useful to predict the impact of guidewire bias for the ablation procedure. After the ablation of calcium, multiple reverberations may be observed due to the smooth surface of the calcification. With respect to side branch occlusion, 2 IVUS findings were reported to have a predictive value. By observing a side branch ostium from a main vessel (Fig. 25-8D), presence of an atherosclerotic plaque at the side branch ostium was associated with 35% occlusion rate, whereas only 8% of the side branches without the ostial plaque resulted in occlusion.12 However, this finding may not be reliable, since an observation of the side branch ostium from the main vessel is not necessarily the same as the direct observation of the side branch ostium. One study investigating left main bifurcation lesions with IVUS pullback from the main vessel and the side branch showed that IVUS evaluation of the side branch ostium from the main vessel is only moderately reliable.13 Another finding is the “eyebrow” sign, which can be identified in the longitudinal view as a carina with a spiky morphology (Fig. 25-8E).14 Protection with a guidewire or predilatation may be utilized to avoid the side branch occlusion. Coronary aneurysm can be observed in preinterventional IVUS imaging or in postinterventional long-term follow-up IVUS imaging, as a consequence of an interventional procedure (Fig. 25-8F). True coronary aneurysm is defined as more than 50% of lumen area enlargement than the proximal reference with an intact vessel wall. In contrast, pseudoaneurysm shows a loss of vessel wall integrity and damage to adventitia or perivascular tissue.
FIGURE 25-8
Examples of preinterventional qualitative IVUS assessment. A. Echo-attenuated plaque is characterized by absence of the ultrasound signal behind the plaque that was either hypoechoic or isoechoic to the adventitia, despite absence of calcium, and is usually found in patients with acute coronary syndrome. B. IVUS findings of a thrombus vary depending on its content and age; however, acute thrombus often presents as an intraluminal mass with a layered, lobulated, or pedunculated appearance. Adjunctive intracoronary drug administration or using a distal protection device may be warranted in this setting. C. In treating a heavily calcified lesion, especially with circumferential calcification called “Napkin ring appearance,” plaque modification with rotational atherectomy may be considered as an adjunctive therapy. D. Presence of an atherosclerotic plaque at the side branch ostium may be associated with an increased risk of occlusion after main vessel stenting. Accurate assessment requires direct imaging of the side branch. E. “Eyebrow” sign, defined in the longitudinal view as a carina with a spiky morphology, was also reported to predict side branch encroachment by main vessel intervention. F. Coronary aneurysm can be observed in preinterventional IVUS imaging, or in postinterventional long-term IVUS follow-up as a consequence of an interventional procedure.

As stated previously, optimal device selection in terms of type and size are achieved with data acquired from quantitative IVUS assessment of the target lesion. Information obtained from earlier IVUS studies include the mechanism of luminal gain after percutaneous transluminal coronary angioplasty (PTCA) or stent deployment consist of vessel expansion (EEM expansion) and decrement of plaque volume (axial redistribution, compression, and distal embolization).15
CLinical Outcomes with Ultrasound Trial (CLOUT) by Stone et al. was the first clinical investigation which demonstrated the safety and usefulness of IVUS-guided angioplasty compared to angiography alone, in which a balloon sizing protocol of the average of the lumen and media-to-media diameters at the reference segment for cases without extensive calcification was employed.16 A subsequent randomized, multicenter clinical trial (Balloon Equivalent to STent [BEST] study) comparing IVUS-guided angioplasty vs stenting employed a balloon sizing protocol of media-to-media diameter measured at the lesion.17 This aggressive balloon strategy resulted in a 56% reduction of stent usage (crossover of 44% patients from angioplasty strategy to stenting) with similar 6-month angiographic and clinical outcomes. In a contemporary DES trial of complex lesions, known as the Angiography Versus IVUS Optimization (AVIO) trial, the size of the post-dilatation balloon was selected based on the average of the media-to-media diameters at multiple sites within the stented segment.18 Precise vessel size measurement is also critically important for size selection of self-expanding or because these devices are not amendable once underdeployed in the lesion.
Angiographically “normal” reference segments can show 35% to 51% of plaque burden when evaluated by IVUS, and therefore, assessment of true lesion length by IVUS dictates the exact length of stent necessary to appropriately scaffold a lesion. To date, several IVUS studies of DES have identified greater reference plaque burden (inflow/outflow disease) as an independent predictor of subsequent stent edge restenosis or thrombosis.19-21 The Impact of Stent Deployment Techniques on Clinical Outcomes of Patient Treated with the Cypher Stent (STLLR) trial also demonstrated that geographic miss (defined as the length of injured or stenotic segment not fully covered by the DES) had a significant negative impact on both clinical efficacy and safety at 1 year following sirolimus-eluting stent implantation.22 Thus, stent length is commonly recommended to be long enough to cover the entire lesion. Importantly, however, longer stent length has also been reported to be independently associated with DES restenosis and thrombosis. One practical approach was proposed in a single-center registry, where unique stepwise IVUS criteria (plaque burden <50% as the primary target zone) determined the optimal landing zone for DES.23 Spot stenting may also be an alternative option, which has shown favorable clinical outcomes in the DES era.24
Detailed assessment of left main coronary artery disease is one of the most clinically important applications of IVUS, since left main disease is directly related to patient mortality. Angulations, calcification, or spasm in this location often lead to poor catheter engagement and confound angiographic interpretation. Several studies have shown that high percentages of patients that appear normal on angiography have left main coronary artery disease detected by IVUS.25-27 Conversely, another IVUS study has demonstrated that only less than half of angiographically ambiguous left main stenosis have a significant stenosis.28 This was especially true for ostial left main coronary disease where only 36% of the lesions had a significant stenosis and 41% had plaque burden <50% when assessed by IVUS. Furthermore, a recent study also reported a slight overestimation of fractional flow reserve (FFR) for the functional assessment of left main disease when a significant downstream stenosis coexists in the proximal left anterior descending or circumflex artery.29 Plaque distribution in left main bifurcation lesions can also be evaluated more accurately with IVUS compared with conventional angiographic classifications.30
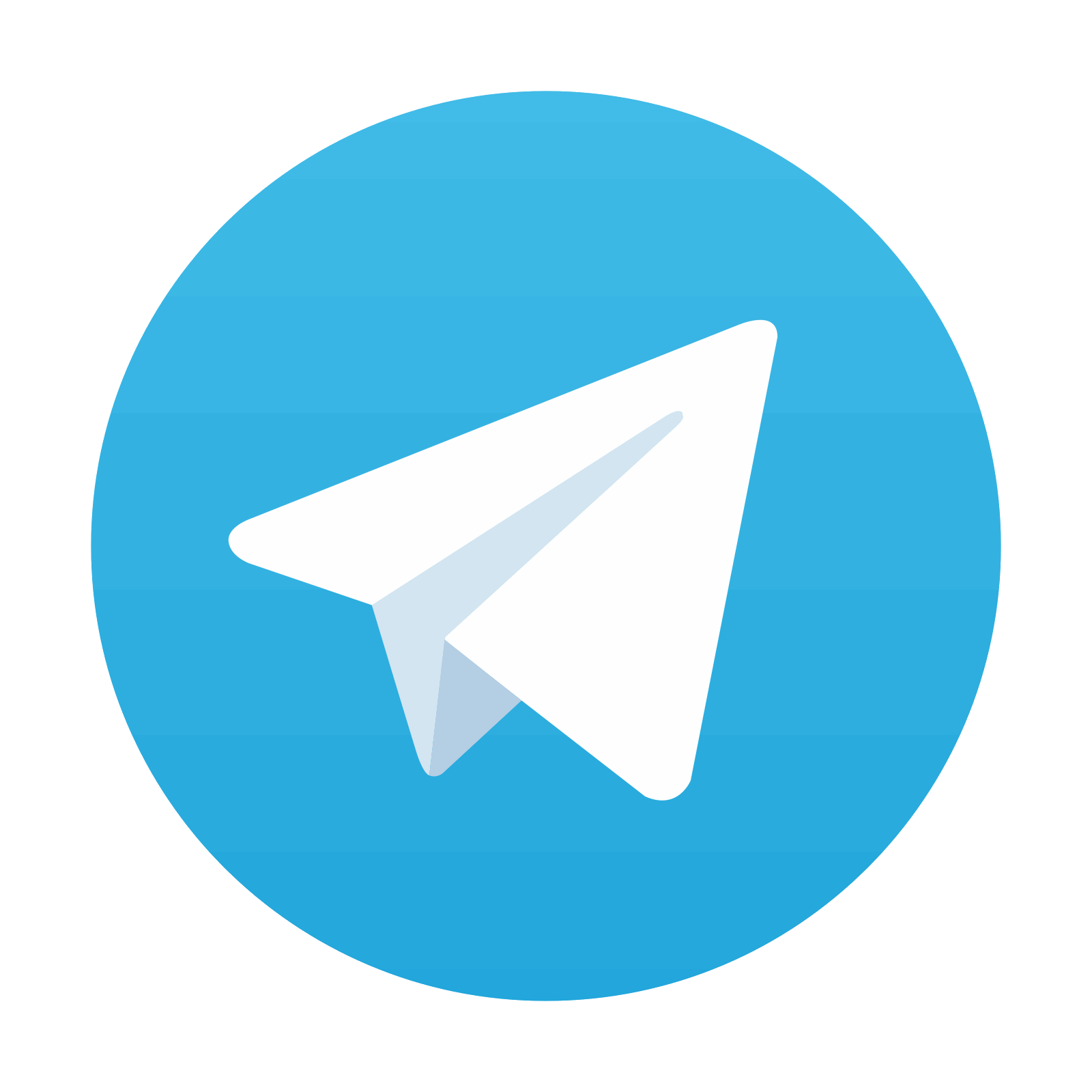
Stay updated, free articles. Join our Telegram channel

Full access? Get Clinical Tree
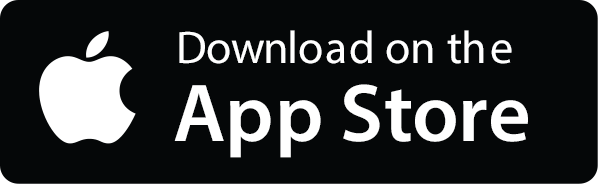
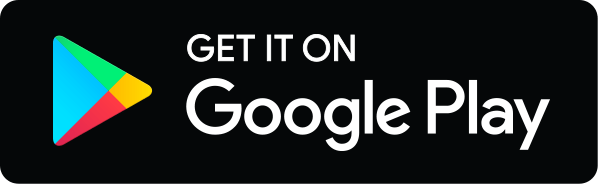