Although coronary angiography remains the reference standard for diagnosing critical epicardial coronary artery disease (CAD), it has a number of limitations. Identifying functionally significant intermediate coronary narrowings, characterizing plaque morphology, detecting moderate diffuse intimal thickening, evaluating the results of percutaneous coronary intervention (PCI), and assessing the status of the microvasculature all remain challenging with coronary angiography alone.1-6 Technologic advancements have made several adjunctive diagnostic techniques available that allow the interventional cardiologist to address the limitations of coronary angiography and more thoroughly assess the coronary circulation.
In this chapter we will focus on the role of the coronary pressure wire to measure fractional flow reserve (FFR) and guide decisions regarding coronary revascularization. The background and derivation of FFR were already covered in Chapter 6. We will briefly review the concept of FFR and then detail how to measure FFR, outline the clinical indications for FFR assessment, and present data supporting its role in the cardiac catheterization laboratory.
The significance of intracoronary pressure gradients and their reflection of the severity of underlying CAD has been appreciated for many years.7 However, it was not until the advent of miniaturized pressure sensors that allowed a wire-based approach to measuring coronary pressure and not until the development and validation of the FFR index by Pijls and De Bruyne that intracoronary pressure measurements have become a routine procedure in many catheterization laboratories.8,9
FFR is defined as the fraction of maximal blood flow perfusing the myocardium in the presence of stenosis compared with the maximal blood flow that would reach the myocardium in the theoretical absence of the stenosis. Because the measurement is made at maximal vasodilation, resistance is minimized and flow becomes proportional to pressure. In a normal epicardial artery, there is little pressure decrement along the vessel, and distal pressure is roughly equal to proximal pressure. Therefore, in a diseased vessel, one can estimate the distal flow or pressure in the theoretical absence of the disease by measuring the proximal pressure. FFR then can be calculated easily in a diseased vessel by dividing the distal pressure, measured with a coronary pressure wire, by the proximal pressure, measured with the guiding catheter during peak hyperemia.8
Compared with other techniques for determining the functional significance of a coronary narrowing, measuring FFR has several inherent advantages. It has an absolute normal value of 1.0 and a narrow range of normal values extending from 0.94 to 1.0.10 Because the measurements are made at maximal vasodilation, the effects of resting hemodynamics are eliminated, making FFR an extremely reproducible index, independent of blood pressure and heart rate.11 Compared with measuring CFR to evaluate intermediate coronary lesions, FFR is advantageous because it is epicardial artery-specific and independent of the microcirculation (Table 24-1). As described in more detail below, FFR has a narrow cutoff range for identifying ischemia-producing lesions. Numerous studies have shown that an FFR less than 0.75 to 0.80 correlates strongly with the presence of ischemia on a variety of noninvasive stress tests and predicts clinical outcome with or without percutaneous coronary intervention (PCI).
Normal value of 1.0 in every vessel and every patient |
Narrow ischemic threshold at <0.75-0.80 |
Independent of hemodynamic changes |
Extremely reproducible |
Specific for the epicardial vessel |
Independent of the microvasculature |
Accounts for collateral flow |
The coronary pressure wire is a standard 0.014-in angioplasty guidewire with a high-fidelity pressure transducer mounted 3 cm from the tip of the wire, at the junction of the radiopaque and radiolucent segments. There are now multiple manufacturers of pressure wires, each of which have their own consoles which analyze and display the pressure recordings. The 2 oldest and most commonly used ones (St. Jude Medical Systems and Philips Healthcare) incorporate piezoelectric technology to measure intracoronary pressure. More recently introduced wires (Opsens and Boston Scientific) utilize optical sensors to measure pressure. The potential advantages of these newer wires are less propensity for pressure drift and improved wire handling characteristics. Finally, a micro catheter (Acist Medical Systems) with an optical pressure sensor mounted near its tip has been introduced and has the added advantage of allowing measurement of distal pressure by advancing the microcatheter over a workhorse wire. However, it has the theoretical disadvantage of increasing stenosis severity (because of its larger crossing profile compared to a 0.014-inch wire alone) and lowering FFR, particularly in smaller vessels and more severe lesions.
To measure FFR, it is traditionally necessary to connect the pressure console to the catheterization laboratory pressure monitoring system and to calibrate the guiding catheter and pressure wire. Newer wireless systems and integrated consoles allow the pressure wire to communicate with the cath lab system without external connectors and facilitate this process. After administering intravenous heparin (or another antithrombotic agent) and intracoronary nitroglycerin (100-200 micrograms), the pressure wire should be advanced out of the guiding catheter so that the pressure transducer is positioned at the ostium of the guiding catheter. At this location, both the pressure wire and the guiding catheter should display identical pressures. One should flush the guiding catheter with saline to ensure an accurate pressure recording. If this is not the case, the pressure wire can be equalized to the guiding catheter. This step can be performed with the catheter and wire in the aorta in the presence of ostial coronary disease. The pressure wire is then advanced to the distal part of the vessel and a vasodilating agent such as adenosine is administered. It is important to start with the sensor in the distal two-thirds of the vessel so that not only the lesion in question, but also any other atherosclerosis in the vessel can be interrogated. The FFR of the vessel is the lowest value achieved by dividing the simultaneously measured mean distal pressure by the mean proximal pressure during maximal steady state hyperemia (Fig. 24-1). Occasionally with intravenous adenosine variability in the delivery of and/or response to adenosine results in fluctuations in the Pd/Pa ratio. If this occurs, the lowest Pd/Pa should be taken as the FFR.12 At times, the pressure wire console will record an inaccurate FFR because of an artifact in the pressure tracing, such as can occur when the manifold is open in order to inject intracoronary adenosine. For this reason, it is always important for the operator to review the tracing and determine the correct FFR.
FIGURE 24-1
Example of fractional flow reserve (FFR) determination. The upper red tracing represents the mean and phasic pressure recorded from the guiding catheter and the lower green tracing represents the mean and phasic pressure recorded from the pressure wire in a patient with a physiologically significant stenosis of the left anterior descending artery. The nearly horizontal yellow line above the 2 tracings represents the continuous distal pressure divided by proximal pressure calculation, and the vertical line marks the peak gradient and represents the FFR of 0.72.

If it is not possible to manipulate the pressure wire down the desired vessel, one can remove the pressure wire after equalization and wire the vessel with a more maneuverable wire through an exchange microcatheter. Once the wire and microcatheter have been advanced to the desired location, the more maneuverable wire can be removed. The pressure wire can then be advanced through the microcatheter to the desired location and the microcatheter can then be pulled back into the guiding catheter. At this point, FFR can be measured in the usual fashion. Alternatively, one can use the Navvus microcatheter (ACIST RXi) to measure pressure over the more maneuverable workhorse wire.
Achieving maximal hyperemia is critical in order to accurately measure FFR. If hyperemia is submaximal, the gradient across the stenosis in question will be underestimated and FFR will be overestimated. The reference standard agent for achieving peak vasodilatation is intravenous adenosine. Ideally, it is administered through a central vein or large bore peripheral line. Peak hyperemia typically occurs within 1 minute and is signaled by the onset of chest pain (not due to ischemia) and or shortness of breath. These symptoms are generally well tolerated, particularly if the patient is forewarned and can be helpful in that they assure the operator that hyperemia has occurred. Because the hyperemia lasts as long as the infusion continues, intravenous adenosine is advantageous in that it allows more careful determination of the peak gradient. In addition, it affords the operator the ability to slowly pull back the pressure wire proximally in order to identify the area of stenosis. This can be particularly useful if there is diffuse disease or multiple lesions in the vessel (Fig. 24-2). Finally, in the setting of ostial left or right coronary lesions, it allows the operator time to remove the guide catheter from the ostium of the vessel in order to accurately measure FFR.
FIGURE 24-2
A. Example of a slow pullback of the pressure wire during maximal hyperemia with a focal step-up in pressure indicative of a focal high grade coronary lesion. B. Example of a slow pullback of the pressure wire during maximal hyperemia with a gradual step-up in pressure indicative of moderate diffuse coronary disease.


Intracoronary adenosine is another common method for measuring FFR which in the United States is less expensive and easier to administer. Its major drawback is its short peak effect, which is roughly 10 to 15 seconds. In addition, because it is given via the coronary artery, the potential exists for incomplete administration down the coronary artery and partial injection or reflux into the aorta. Recently, it has been shown that higher doses than previously used are necessary to achieve peak hyperemia, but typically 100 micrograms in the right coronary artery and 200 micrograms in the left coronary are adequate.13 If there is any doubt regarding adequate hyperemia, it is important to use either intravenous adenosine or an alternative agent, such as intracoronary papaverine.
Using intracoronary papaverine to measure FFR is advantageous because its peak effect is longer lasting than intracoronary adenosine, roughly 45 seconds, which makes more accurate determination of FFR possible and which allows time to perform a slow pullback of the pressure sensor. In addition, papaverine is inexpensive and easy to prepare and administer. Its major drawback is its potential arrhythmogenic effect. In an older series, the incidence of a serious arrhythmia (ventricular tachycardia or torsades de pointes) was approximately 1%.14 Fortunately, these arrhythmias were usually self-limited. Papaverine can precipitate in ionic contrast medium, which may induce the arrhythmia.
A newer hyperemic agent, regadenoson, has been recently introduced. It is an agonist specific for the adenosine A2A receptor and has the potential advantage of inducing less bronchoconstriction than adenosine. A recent study demonstrated equivalent hyperemia compared to intravenous (IV) adenosine with excellent reproducibility.15 An advantage is that it can be given as a single peripheral IV bolus. A potential drawback is that the hyperemic effect lasts between 45 seconds and 10 minutes, but its duration is unpredictable. A number of other agents are available for achieving hyperemia and are listed in Table 24-2.
Agent | Route | Dose | Comments |
---|---|---|---|
Adenosine | IV infusion | 140 μg/kg/min | Reference standard. Side effects include dyspnea and chest pain. Prolonged hyperemia allows pressure wire pullback. |
Adenosine | IC bolus | >100-200 μg | Easy to use, inexpensive, and no significant side effects. Transient heart block at high doses. Hyperemia lasts only 10-15 seconds. |
Adenosine | IC infusion | 240-360 μg/min | Inconvenient set-up. Fewer side effects compared to IV infusion. Prolonged hyperemia allows pullback. Not well-validated. |
Regadenoson | IV bolus | 400 μg | Convenient, single IV bolus. Expensive. Side effects similar to IV adenosine but less severe and briefer. Hyperemia lasts 20 seconds-10 min. |
Papaverine | IC bolus | 10-20 mg | Easy to use, inexpensive. Rare, but significant side effect of polymorphic VT. Hyperemia lasts 30 seconds, allowing pullback. |
Nitroprusside | IC bolus | 0.3-0.9 μg/kg | Easy to use, inexpensive. Major side effect is hypotension. Hyperemia lasts 50 seconds allowing pullback. Not well-validated. |
Dobutamine | IV infusion | 50 μg/kg/min | Inconvenient as it takes time for onset an offset. Side effects include palpitations and hypotension. Not well-validated for FFR. |
Nicorandil | IC bolus | 2 mg | Not available in United States. Fewer side effects compared to IV adenosine. Hyperemia lasts 30 seconds. Not well-validated. |
If the FFR is abnormal and PCI is necessary, the operator can disconnect the pressure wire from the interface coupler and a balloon catheter or stent can be advanced over the pressure wire in the usual fashion. During balloon inflation the pressure wire can be reconnected and the coronary wedge pressure, a reflection of collateral flow, can be recorded.16 After completing the PCI, the pressure wire can be reconnected and FFR remeasured. Finally, it is important after measuring FFR, whether or not PCI has been performed, to reposition the pressure sensor at the ostium of the guiding catheter before removing the wire completely, to ensure that equal pressures are recorded from the guiding catheter and the pressure wire (ie, that no “drift” in either pressure recording system has occurred). If there is a difference greater than 0.05, then one should consider reequalizing the pressure wire and remeasuring FFR.
Indications for measuring FFR are listed in Table 24-3. The most common indication for measuring FFR is to determine the physiologic significance of an intermediate coronary lesion. As many more patients present to the catheterization laboratory without a prior noninvasive assessment for myocardial ischemia and with an awareness of the limitations of angiography alone to identify functionally important moderate lesions, the role of a relatively simple method, such as measuring FFR, for interrogating this challenging subset has expanded. Pijls and colleagues performed a landmark trial comparing FFR with 3 noninvasive stress tests.1 They showed that an FFR less than 0.75 is 100% specific for predicting myocardial ischemia; the sensitivity was 88% and the predictive accuracy was 93%. Since this seminal report, clinical experience and subsequent studies have found a “grey zone” for FFR between 0.75 and 0.80. If the FFR falls in this range, one should use clinical judgment to decide if revascularization is warranted. If the patient has typical symptoms and a proximal lesion in a large vessel that is amenable to stenting, then revascularization should be performed. If the patient has no symptoms, atypical symptoms, and/or the lesion is located in a small or distal vessel, then medical therapy may be more appropriate. If the FFR is greater than 0.80, it is extremely unlikely that significant ischemia is present. FFR is not a dichotomous variable but a continuous one reflecting the fact that lower FFR values correspond with higher event rates in patients treated medically. This concept is an important one that has recently been highlighted.17
Coronary lesion of unclear significance |
Multiple lesion assessment in multivessel coronary artery disease (CAD) |
Indeterminate left main coronary stenosis |
“Jailed” sidebranch evaluation |
After percutaneous coronary intervention |
Nonculprit lesions at the time of acute myocardial infarction |
Investigate for angiographically silent diffuse disease |
To determine the safety of employing FFR to guide the operator’s decision to defer PCI, Bech and colleagues, in the DEFER trial, measured FFR in 325 patients with chest pain, no prior stress test, and intermediate coronary lesions who had been referred for PCI.18 One hundred and forty-four of these patients had an FFR less than 0.75, underwent PCI as planned, and formed a reference group. One hundred and eighty-one had an FFR of 0.75 or greater and were randomized to deferral of PCI (n = 91) or performance of PCI (n = 90). The deferral group had a greater freedom from major adverse cardiac events at 2 years, although not statistically significant, compared with the performance group: 89% versus 83%, respectively. The reference group had a significantly worse event-free survival compared with the deferral group (78% vs 89%, P = 0.03), presumably because they had more significant CAD. Five-year follow-up from this study was reported and found that the rate of cardiac death or myocardial infarction (MI) in the deferral group was less than half of the rate in the performance group (3.3% vs 7.9%, P = 0.21).19 Recently, the 15-year follow-up from this trial has been published, revealing no difference in long-term mortality between the two groups, but a significantly lower rate of MI in the patients assigned to deferral of PCI (2.2% vs 10%, P = 0.03).20 This trial clearly showed that it is safe to defer PCI in stable patients with an intermediate lesion and an FFR of 0.75 or greater. Numerous other registries have confirmed the findings in the DEFER trial.21
The FFR versus Angiography for Multivessel Evaluation (FAME) trial established the role of routine FFR measurement for guiding PCI in patients with multivessel CAD amenable to PCI.22 In the FAME trial, 1005 patients with coronary lesions of >50% diameter stenosis in 2 or 3 major epicardial vessels which were amenable to PCI with drug-eluting stents were randomized to either the standard of care, angiography-guided PCI, or to FFR-guided PCI. Angiography-guided PCI was performed based on the noninvasive clinical data and the angiographic appearance of the lesions. For the FFR-guided PCI arm, FFR was measured across every stenosis and only if the FFR was ≤0.80 was PCI performed on the particular stenosis.
There were approximately 3 lesions identified per patient in both groups, but the angiography-guided PCI group received significantly more stents per patient, 2.7 ± 1.2 versus 1.9 ± 1.3, P < 0.001. In addition, significantly more contrast media was administered to the angiography-guided PCI patients, 302 ± 127 versus 272 ± 133 mL, P < 0.001. Importantly, the duration of the procedure was identical between the two groups—although measuring FFR adds time to the procedure, avoiding unnecessary PCI saves time. Despite the fewer stents, the percentage of patients free of angina at 1 year was numerically higher in patients randomized to FFR guidance (81.3% vs 77.9%, P = 0.20).
The primary endpoint of the FAME trial was the 1-year rate of death, MI or repeat revascularization, which occurred in 18.3% of the angiography-guided PCI patients as compared to 13.2% of the FFR-guided PCI patients, P < 0.02 (Fig. 24-3). This significant reduction resulted from 30% to 40% relative risk reductions in each component of the composite endpoint. In addition, the rate of death and MI was reduced significantly in the FFR-guided PCI group (7.3% vs 11.1%, P = 0.04). Subsequently, the 2-year results of FAME were reported and revealed a persistent reduction in the composite of death, MI and repeat revascularization (17.9% vs 22.4%, P = 0.08).23 Importantly, the hard endpoints of death and MI remained significantly lower in the FFR-guided PCI patients (8.4% vs 12.9%, P = 0.02). The Kaplan-Meier curves at 1 and 2 years showed that the benefit attributed to FFR guidance occurred early, due to fewer procedural-related events, and continued during follow-up, due to fewer late events. Recently, the 5-year follow-up from the FAME trial has been published and found continued separation of the curves with respect to major adverse cardiac events between the two groups, without any significant late catch-up in events in the FFR-guided PCI group.24
The FAME trial further highlighted the limitations of coronary angiography alone for identifying lesions responsible for ischemia.25 Approximately 35% of the lesions graded between 50% and 70% narrowed had an ischemic FFR, while roughly 20% of the lesions between 71% and 90% narrowed had nonischemic FFR values (Fig. 24-4). This would suggest that all lesions between 50% and 90% narrowed could benefit from FFR measurement to accurately determine their functional significance.
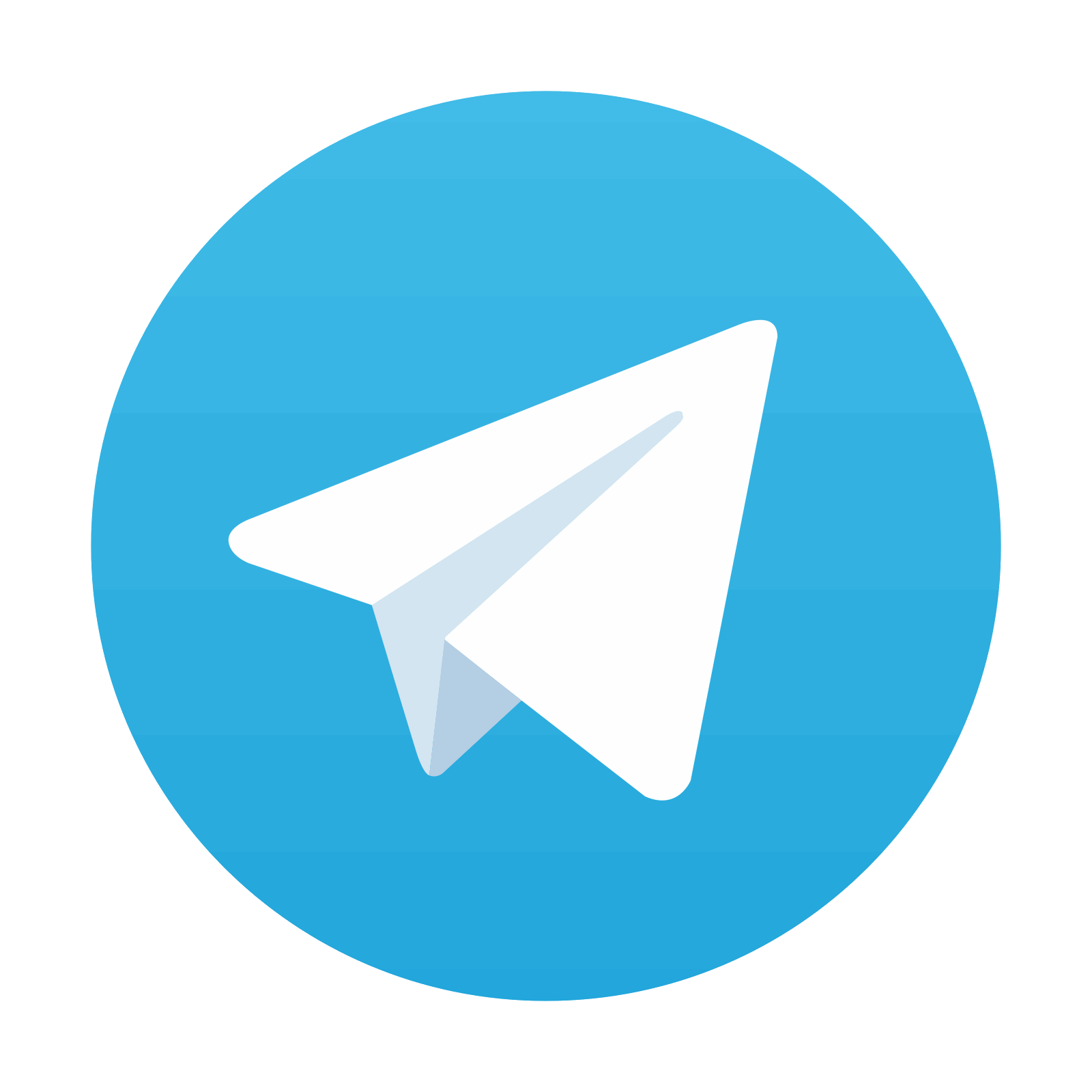
Stay updated, free articles. Join our Telegram channel

Full access? Get Clinical Tree
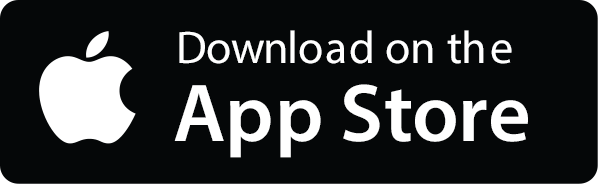
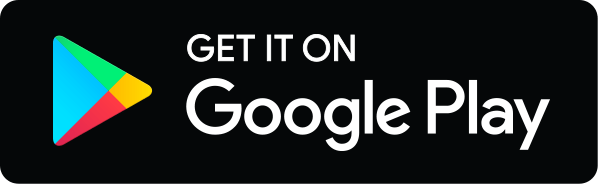