Adenovirus-Mediated Gene Delivery for Promoting Collaterals in Patients with Coronary and Peripheral Artery Disease
Jai Pal Singh
For patients with severe ischemic coronary artery disease (CAD) and peripheral arterial disease (PAD), the major mode of treatment at present is the restoration of blood flow by means of surgery or mechanical devices such as balloon angioplasty, stenting, and, more recently, drug-eluting stent implantation. An additional or perhaps alternate approach to treat these patients is by the induction of collateral development through therapeutic angiogenesis. This treatment will particularly benefit those patients with cardiovascular risk factors in whom collateral development is deficient (1, 2, 3, 4, 5), patients with diffused and distal disease, and those without option for further surgical interventions. Therapeutic collateral formation can occur through a combination of angiogenesis, the sprouting of capillaries from the existing vessels; vasculogenesis, the de novo formation of vessels from the precursor cells or angioplasts; and arteriogenesis, the enlargement of existing arteries by remodeling. Arteriogenesis is considered a more relevant biological mechanism to achieve the formation of vessels large enough to meet the blood supply needed for distal tissue perfusion (6).
A large body of experimental data from animal models and early clinical investigations support the hypothesis for angiogenic therapy. Collateral formation and the resulting protection from subsequent ischemia have been documented in humans (1). Cardiovascular risk factors such as hypercholesterolemia, hypertension, diabetes, obesity, and smoking are associated with a reduced ability to develop collaterals (2, 3, 4, 5). In animal models, the formation of new blood vessels is stimulated following ischemia. Furthermore, the angiogenic response to ischemia can be enhanced by the administration of angiogenic proteins or genes. In preclinical models, the enhancement of angiogenesis is associated with a functional improvement in ischemic heart (7, 8, 9, 10, 11) and hind limbs (12,13). Data from early clinical trials also have provided tantalizing evidence for the improvement of cardiac or limb blood flow and function following angiogenic therapy (14, 15, 16, 17, 18, 19, 20, 21). These early investigations have produced encouraging results that demonstrate improved vascularity, tissue perfusion, and functional benefit following the delivery of fibroblast growth factor (FGF) and vascular endothelial growth factor
(VEGF) (15, 16, 17, 18, 19, 20, 21). However, subsequent larger studies have failed to confirm the efficacy of FGF-2 and VEGF therapy (15,21). The lack of efficacy could have been attributed to a number of factors including inadequate dose of growth factor, the short duration of treatment, the patients selected for therapy, the study design, and the clinical endpoint used in the study. The early angiogenesis clinical trials were to some extent reminiscent of the restenosis trials, in which the agents were delivered at subtherapeutic doses for an apparently inadequate duration. By overcoming the pharmacokinetics-pharmacodynamics issues by using the drug-eluting stent, a highly successful therapy for restenosis has been developed. Like restenosis, a successful angiogenic therapy may require high concentrations of a drug at the target tissue for an extended period.
(VEGF) (15, 16, 17, 18, 19, 20, 21). However, subsequent larger studies have failed to confirm the efficacy of FGF-2 and VEGF therapy (15,21). The lack of efficacy could have been attributed to a number of factors including inadequate dose of growth factor, the short duration of treatment, the patients selected for therapy, the study design, and the clinical endpoint used in the study. The early angiogenesis clinical trials were to some extent reminiscent of the restenosis trials, in which the agents were delivered at subtherapeutic doses for an apparently inadequate duration. By overcoming the pharmacokinetics-pharmacodynamics issues by using the drug-eluting stent, a highly successful therapy for restenosis has been developed. Like restenosis, a successful angiogenic therapy may require high concentrations of a drug at the target tissue for an extended period.
Several approaches are being investigated for the sustained delivery of angiogenic or arteriogenic agents for therapy. Sustained-release formulations of angiogenic cytokines have shown promising results in preclinical studies (22, 23, 24). Although, small clinical trials with sustained release formulations of FGF and VEGF proteins also have shown improvement in perfusion and cardiac functions (25, 26, 27), further studies are needed to demonstrate the therapeutic potential of sustained-release angiogenic agents. At present, a more widely used approach to test the efficacy of angiogenic agents is through the delivery of genes encoding the angiogenic protein. Clinical testing of VEGF, Del-1, and hepatocyte growth factor (HGF) as plasmid DNA is underway (19,28,29). Although safety is an important advantage in gene delivery by plasmid, the low transfection efficiency and therefore low level of transgene expression remains a major limitation. Gene delivery by adenoviral vectors allows high transfection efficiency and transgene expression for about 2 weeks (30). VEGF, hypoxia inducible factor-1α (HIF-1α), and FGF-4 currently are under investigation for adenoviral-mediated gene delivery for collateral development (31, 32, 33, 34).
DEVELOPMENT OF ADENOVIRAL TRANSGENE FOR THERAPY
The delivery of a nonreplicating adenoviral vector containing the gene of interest allows the generation of high local concentrations of the desired therapeutic protein over an extended period. Adenoviral transfection occurs through the Coxsackie-Adenoviral Receptor (CAR) (35) and through αvβ3 and αvβ5 integrins (36). The expression of high levels of these receptors in the cardiac and skeletal muscle and vasculature allows efficient transfection. The adenovirus can transfect both dividing and nondividing cells. The extrachromosomal viral DNA providing gene expression for about 2 weeks appears suitable for angiogenic therapy (30,37). The first-generation replication-deficient viral vector was generated by deletion of the E1 region of the viral genome, which encodes for the immediate early genes required for viral DNA replication and the expression of viral late proteins (Fig. 26.1). However, the first-generation vector produces significant inflammatory response in vascular cells (38,39). The inflammatory response is significantly lower with the second-generation vector, in which the E1 and E4 regions are deleted (40). Further reduction in immune response has been achieved in the most recent generation of vectors, known as helper-dependent viral vectors (gutless vectors), from which the majority of the viral genome was deleted. Although the inflammatory response to the gutless viral vector was lower, the magnitude of gene expression was also lower, presumably due to the reduced transfection efficiency of the helper-dependent viral vector (41).
The general plan for the development of adenoviral gene therapy is shown in Figure 26.2. A suitable vector containing the angiogenic-arteriogenic gene first is prepared. The vector containing the transgene then is tested for plaque forming units (pfu), which measure the active virus particles transfecting the cells. Various concentrations of the vector then are tested for expression of the target gene in a cell system. The HEK 293 cells are a commonly used system for testing the level of protein production following vector transfection. The gene product can be measured by messenger RNA (mRNA) levels or protein levels using antibodies or an enzyme-linked immunosorbent assay (ELISA) test. It is advisable to test the biological activity of the protein as well. The adenoviral vector then is tested for in vivo expression of the transgene in the target tissue, in this case the skeletal or cardiac muscle. The level of mRNA expressed at different doses of the viral vector is determined by quantitative reverse transcription polymerase chain reaction (RT-PCR). For secreted proteins, plasma levels also may serve as an indicator of transgene expression in vivo. The time course of transgene expression is determined to establish its compatibility with the therapeutic need. The results from these studies are used to develop an efficacy-testing strategy in the ischemia models. The widely used preclinical models for PAD are mouse hind limb and rabbit hind limb ischemia models (12). For coronary ischemia, a porcine model is considered more suitable for testing of gene therapy. The pig
coronary model not only allows the evaluation of the efficacy of the target gene but also the delivery catheter (10,11). Following the demonstration in preclinical models of the efficacy of the adenoviral vector containing a transgene, the vector is then characterized for safety, pharmacokinetics, and method of delivery.
coronary model not only allows the evaluation of the efficacy of the target gene but also the delivery catheter (10,11). Following the demonstration in preclinical models of the efficacy of the adenoviral vector containing a transgene, the vector is then characterized for safety, pharmacokinetics, and method of delivery.
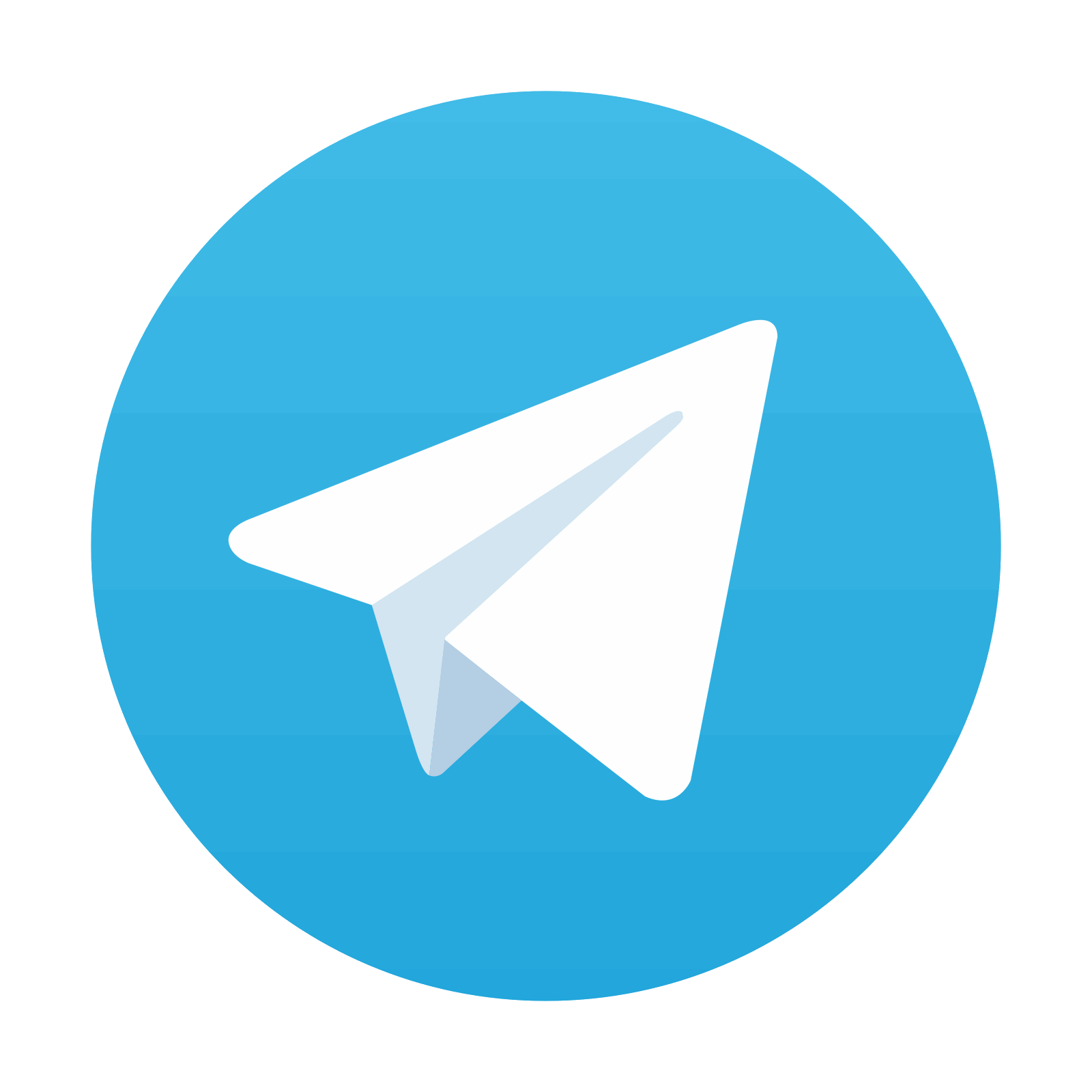
Stay updated, free articles. Join our Telegram channel

Full access? Get Clinical Tree
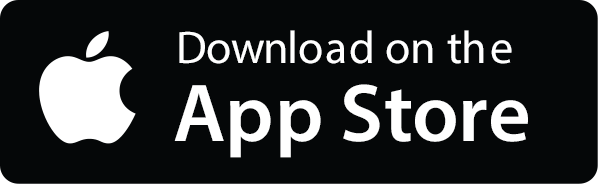
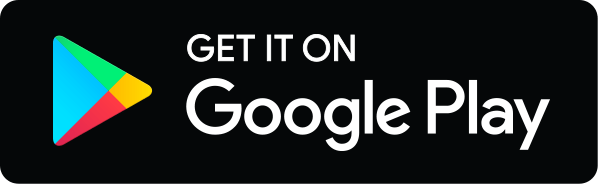