Background
There is currently controversy over whether echocardiography provides reliable estimations of pulmonary pressures. The objective of this study was to determine the factors influencing the accuracy and reliability of estimating right ventricular systolic pressure (RVSP) using echocardiography in patients with advanced lung disease or pulmonary arterial hypertension.
Methods
Between January 2001 and December 2012, 667 patients with advanced lung disease or pulmonary arterial hypertension underwent right heart catheterization and transthoracic echocardiography. Of those, 307 had both studies within 5 days of each other. The correlation and bias in estimating RVSP according to tricuspid regurgitation (TR) signal quality and reader expertise were retrospectively determined. Reasons for under- and overestimation were analyzed. The diagnostic performance of estimated RVSP, relative right ventricular size, eccentricity index, and tricuspid annular plane systolic excursion was compared for classifying patients with pulmonary hypertension (mean pulmonary artery pressure ≥ 25 mm Hg).
Results
Invasive mean and systolic pulmonary artery pressures were strongly correlated ( R 2 = 0.95, P < .001), with mean pulmonary artery pressure = 0.60 × systolic pulmonary artery pressure + 2.1 mm Hg. Among patients undergoing right heart catheterization and transthoracic echocardiography within 5 days, level 3 readers considered only 61% of TR signals interpretable, compared with 72% in clinical reports. Overestimation in the clinical report was related mainly to not assigning peak TR velocity at the modal frequency and underestimation to overreading of uninterpretable signals. When the TR signal was interpretable, the areas under the curve for classifying pulmonary hypertension were 0.97 for RVSP and 0.98 for RVSP and eccentricity index ( P > .05). When TR signals were uninterpretable, eccentricity index and right ventricular size were independently associated with pulmonary hypertension (area under the curve, 0.77).
Conclusions
Echocardiography reliably estimates RVSP when attention is given to simple quality metrics.
Highlights
- •
Sixty-one percent of TR signals were interpretable.
- •
RVSP estimated by echocardiography and catheterization were strongly correlated.
- •
Echocardiography showed good classification performance; no severe PH was missed.
- •
Echocardiography laboratory tends to overread TR signals.
- •
The main reason for overestimation is the inability to identify the complete TR signal envelope.
In the past 30 years, echocardiography has emerged as one of the most useful methods for noninvasively estimating pulmonary artery pressures. One of the main Doppler echocardiographic techniques to assess right ventricular (RV) systolic pressure (RVSP) relies on estimating the RV–right atrial pressure gradient from the tricuspid regurgitation (TR) maximal velocity using the modified Bernoulli equation, to which is added the estimated right atrial pressure (RAP). The original 1984 publication of Yock and Popp reported an excellent correlation between RVSP measured by right heart catheterization (RHC) and RVSP simultaneously estimated by echocardiography ( r = 0.93, P < .001). This was later confirmed by several other investigators.
However, in recent years, the accuracy and reliability of echocardiography for estimating RVSP have been challenged, especially in the field of advanced lung disease (ALD) and pulmonary hypertension (PH). In the study of Fisher et al . on 63 subjects with mild or no PH, a weak correlation between invasive and estimated RVSP was reported ( r = 0.23, P < .05), including one third of patients having a difference >10 mm Hg. Rich et al . more recently reported similar findings.
In the present study, we hypothesized that the heterogeneity in the findings noted in previous studies is likely related to the fact that different studies have not always considered TR signal quality. Moreover, there is still no consensus in the literature on which RVSP threshold should be used to classify patients with or without PH (thresholds ranging from 35 to 45 mm Hg). To address these questions, we retrospectively analyzed transthoracic echocardiograms from patients with ALD or idiopathic pulmonary arterial hypertension (PAH) who underwent RHC. Our first objective was to determine the relationship between mean pulmonary artery pressure (MPAP) and systolic pulmonary artery pressures (SPAP) in a large hemodynamic cohort to provide guidance for the selection of the best criteria for RVSP. Our second objective was to assess the accuracy of echocardiography in estimating pulmonary artery pressures and analyze the factors associated with under- or overestimation. Our final objective was to compare diagnostic performance for classifying PH using commonly used metrics of RV size and function, in patients with uninterpretable TR signals.
Methods
Study Population
After obtaining institutional review board approval, we retrospectively reviewed the charts of all patients aged ≥18 years referred to the Stanford University ALD center for RHC between 2001 and 2012 ( n = 704). Eligible subjects had either ALD (chronic obstructive pulmonary disease, interstitial lung diseases, idiopathic pulmonary fibrosis, or cystic fibrosis) or PAH. For our first objective (hemodynamic correlations), all subjects with complete RHC were included ( n = 667); for our second objective, subjects with RHC and complete echocardiography within 5 days were included ( n = 307).
Right Heart Catheterization
A balloon catheter was introduced through the right femoral vein or internal jugular vein after local anesthesia. Patients could be premedicated with 1 to 3 mg of diazepam 30 min before the procedure. The following measurements were obtained: RAP, RVSP, SPAP, diastolic pulmonary artery pressure, MPAP, pulmonary artery wedge pressure, and cardiac output. To estimate the potential effect of premedication on blood pressure, systolic and diastolic blood pressures were measured at baseline before sedation and during the invasive procedure. PH was defined as MPAP on RHC of ≥25 mm Hg.
Echocardiography
Resting TTE was performed using the Philips 7500 or iE33 system (Philips Medical Systems, Andover, MA) with 5-MHz transducers by experienced, credentialed cardiac sonographers. RVSP was estimated from the maximal RV–right atrial pressure gradient, using the modified Bernoulli equation : RVSP = 4 × (maximal TR velocity) 2 + RAP, where maximal TR velocity was measured using continuous-wave Doppler. Windows used to measure maximal TR velocity included parasternal long- and short-axis and RV-modified apical four-chamber views. Only the window with the best quality and highest signal was chosen for peak velocity. TR signals acquired after an ectopic beat or after wide respiratory motion were excluded. In the 26 patients with atrial fibrillation, the third beat after two consecutive relatively equal RR intervals was used, previously reported as the “index-beat method.” Patients with severe dense triangular and early peaking TR were excluded, because SPAP could not be appropriately estimated from the TR signal. RAP was estimated in the subcostal view according to the inferior vena cava (IVC) size and collapsibility following a normal sniff: 3 mm Hg if IVC < 15 mm and collapse ≥ 50%, 5 mm Hg if IVC between 15 and 21 mm and collapse ≥ 50%, 15 mm Hg if IVC ≥ 21 mm and collapse between 10% and 50%, 20 mm Hg if IVC ≥ 21 mm and collapse < 10%, and 10 mm Hg in all other cases.
In this study, we assessed interpretations of the same studies by comparing measurements from the echocardiography laboratory clinical report with offline reassessment by both a level 3–trained cardiologist certified by the National Board of Echocardiography and a level 2–trained cardiologist, who were blinded to RHC values. We also assessed the potential effect of training by the level 2 reader, who blindly reinterpreted all measurements. Level 2 and 3 readers measured maximal Doppler velocities at the peak modal frequency, adjusting Doppler gain to identify the main complete envelope in order to avoid overestimation of maximal velocities. The quality of the TR signal was classified, as shown in Figure 1 A, according to envelope visibility (type 1, complete envelope; type 2, partial envelope but prone to extrapolation; and type 3, unreliable envelope or no signal). TR severity was assessed as absent, mild, moderate, or severe according to American Society of Echocardiography guidelines, integrating the following metrics. Severe TR was defined in the presence of a vena contracta ≥ 7 mm, reversed systolic hepatic vein flow, proximal isovelocity surface area radius > 9 mm, and very large central jet or eccentric wall impinging jet. Mild TR was defined by all of the following parameters: visual small jet, proximal isovelocity surface area radius < 5 mm, and systolic predominance of the hepatic vein flow. Moderate TR was defined as TR not corresponding to the definition of either mild or severe TR. Finally, the beam-to-flow angle between the Doppler cursor and the TR jet was assessed and defined as acceptable when <40°. Contrast methods were not routinely used.

A predefined value of RVSP >40 mm Hg was considered as abnormal in this study on the basis of the previous studies of Chemla et al . and considering the RV outflow tract gradient. Over- and underestimation of echocardiography-based RVSP were defined as a relative difference >20% or <20% of the RHC value. The following routinely acquired RV size and function metrics were also assessed: end-diastolic basal RV/left ventricular (LV) diameter ratio (>0.67 defining an abnormal ratio and measured in the apical four-chamber view), LV systolic eccentricity index (EI; >1.2 defining an abnormal ratio, measured in the short-axis view), and tricuspid annular plane systolic excursion (TAPSE; <17 mm abnormal). Pulmonary acceleration time was not included, because it was available in only 53% of patients.
Statistical Analysis
Quantitative data are expressed as mean ± SD if normally distributed or as medians and interquartile ranges (IQRs) if not normally distributed; qualitative variables are presented as numbers and percentages. The relation between invasive MPAP and SPAP was assessed by regression analysis in the overall population with available complete RHC data. Comparisons between echocardiographic and invasive RVSP were performed by linear regression analysis (and are presented as Pearson coefficients with 95% CIs) and were then compared using Bland-Altman plots of the difference between RVSPs and the mean value of the two RVSPs (with 95% limits of agreement defined as ±1.96 × SD of the difference). Systematic differences between two measurements or two groups were assessed using Student’s t test. The diagnostic performance of echocardiographic estimates of RVSP to identify the presence of PH (defined as invasive MPAP ≥ 25 mm Hg) was assessed by logistic regression and is expressed as the area under the curve (AUC). Intra- and interobserver variability for echocardiography measurements was determined using two-way mixed single-measure intraclass correlation coefficients. P values < .05 were considered to indicate statistical significance. Statistical analyses were performed using SPSS version 14.0 (SPSS, Inc, Chicago, IL). This study was conducted in agreement with the Declaration of Helsinki and was approved by the Stanford University Institutional Review Board.
Results
Study Population
For the entire cohort referred for RHC, the mean age was 51 ± 13 years, and 54% were women. In the subgroup of patients who underwent RHC and echocardiography within 5 days of each other, the mean age was 50 ± 13 years, 59% were women, and two thirds had PH. Seventy-one percent had ALD, and 29% had PAH. The median time delay between echocardiography and RHC was 2 days (IQR, 1–3 days). Characteristics of the study population are presented in Table 1 .
Variable | Total population ( n = 307) | Interpretable signals ( n = 172) | Uninterpretable signals ( n = 135) | P |
---|---|---|---|---|
Clinical characteristics | ||||
Age (y) | 49.5 ± 13.3 | 49.9 ± 13.2 | 49.0 ± 13.4 | .56 |
Men | 125 (41%) | 61 (36%) | 64 (47%) | .07 |
ALD | 219 (71%) | 104 (60%) | 115 (85%) | <.01 ∗ |
PAH | 88 (29%) | 68 (40%) | 20 (15%) | <.01 ∗ |
RHC | ||||
HR (beats/min) | 81.1 ± 15.8 | 82.4 ± 15.7 | 79.8 ± 15.9 | .15 |
SBP (mm Hg) | 117.6 ± 18.1 | 115.8 ± 17.3 | 119.9 ± 19.0 | .05 ∗ |
MPAP (mm Hg) | 36.5 ± 17.8 | 41.4 ± 18.7 | 30.3 ± 14.3 | <.01 ∗ |
MPAP ≥ 25 mm Hg | 206 (67%) | 128 (74%) | 78 (58%) | <.01 ∗ |
RVSP (mm Hg) | 56.9 ± 27.3 | 65.2 ± 28.4 | 46.7 ± 22.1 | <.01 ∗ |
PCWP (mm Hg) | 11.2 ± 6.6 | 10.8 ± 5.4 | 11.8 ± 7.8 | .19 |
Hemodynamic Relationship between MPAP and SPAP
A strong correlation was noted between invasive MPAP and SPAP ( R 2 = 0.95, P < .001). The linear equation that best described this relation was MPAP = .60 × SPAP + 2.1 mm Hg ( Figure 2 ). The MPAP threshold of 25 mm Hg corresponded to an SPAP of 38 mm Hg. The same equation was observed in the subgroup of subjects undergoing RHC and echocardiography within 5 days.
RVSP Estimation According to Reader Expertise and TR Signal Quality
RVSP was reported more frequently in the clinical reports (72%) than by level 3 (61%) or level 2 readers (56%) ( P < .05). Figure 3 shows TR signal quality classification according to etiology. In patients with ALD, TR signals were more frequently either uninterpretable or only prone to extrapolation. In contrast, TR signals were considered interpretable in the majority of patients with PAH (75%).
Relationship between Invasive and Echocardiography-Based RVSP Estimation
There were good to excellent correlations between RVSP estimated by RHC and echocardiography: r = 0.84 (IQR, 0.79–0.87) for clinical reports, r = 0.86 (IQR, 0.82–0.90) for level 2 readers, and r = 0.96 (IQR, 0.95–0.97) for level 3 readers ( P < .001 for all). The correlation for level 3 readers was significantly higher than for level 2 readers or clinical reports ( P < .001). Correlation between invasive and noninvasive RVSP estimations mentioned in the clinical report did not differ significantly when selecting only patients defined as interpretable by level 2 ( r = 0.85; IQR, 0.80–0.89) or level 3 ( r = 0.87; IQR, 0.81–0.91) readers. When selecting only patients who underwent both procedures within 3 days ( n = 287), correlations were as follows: r = 0.84 (IQR, 0.80–0.88) for clinical reports, r = 0.89 (IQR, 0.85–0.92) for level 2 readers, and r = 0.95 (IQR, 0.93–0.96) for level 3 readers ( P < .001 for all). Bland-Altman plots of the correlation between RVSP by RHC and all three levels of echocardiography readers for the total population demonstrated good accuracy (with almost no bias, especially for level 2 readers and clinical reports) but wide limits of agreements (1.96 × SD up to 33 mm Hg for clinical reports), suggesting low precision ( Figure 4 ). Systolic blood pressure during RHC was 6% lower than systolic blood pressure during echocardiography (median, 115 mm Hg [IQR, 104–131 mm Hg] vs 122 mm Hg [IQR, 110–133 mm Hg]; P = .001).
Reasons for Under- and Overestimation of RVSP
Reassessed by another level 3 reader, the level 3 reader underestimated RVSP in 8.6% of patients and overestimated in 5.4% ( Figure 5 ). Absence of a well-defined TR envelope (88%), time delay between the two examinations, and discrepancies between RAP estimation of >10 mm Hg (although rare, accounting for three patients) were the three main reasons for underestimation, whereas overestimation was associated with poorer TR signal quality ( Figure 1 B) and a greater delay between RHC and echocardiography.
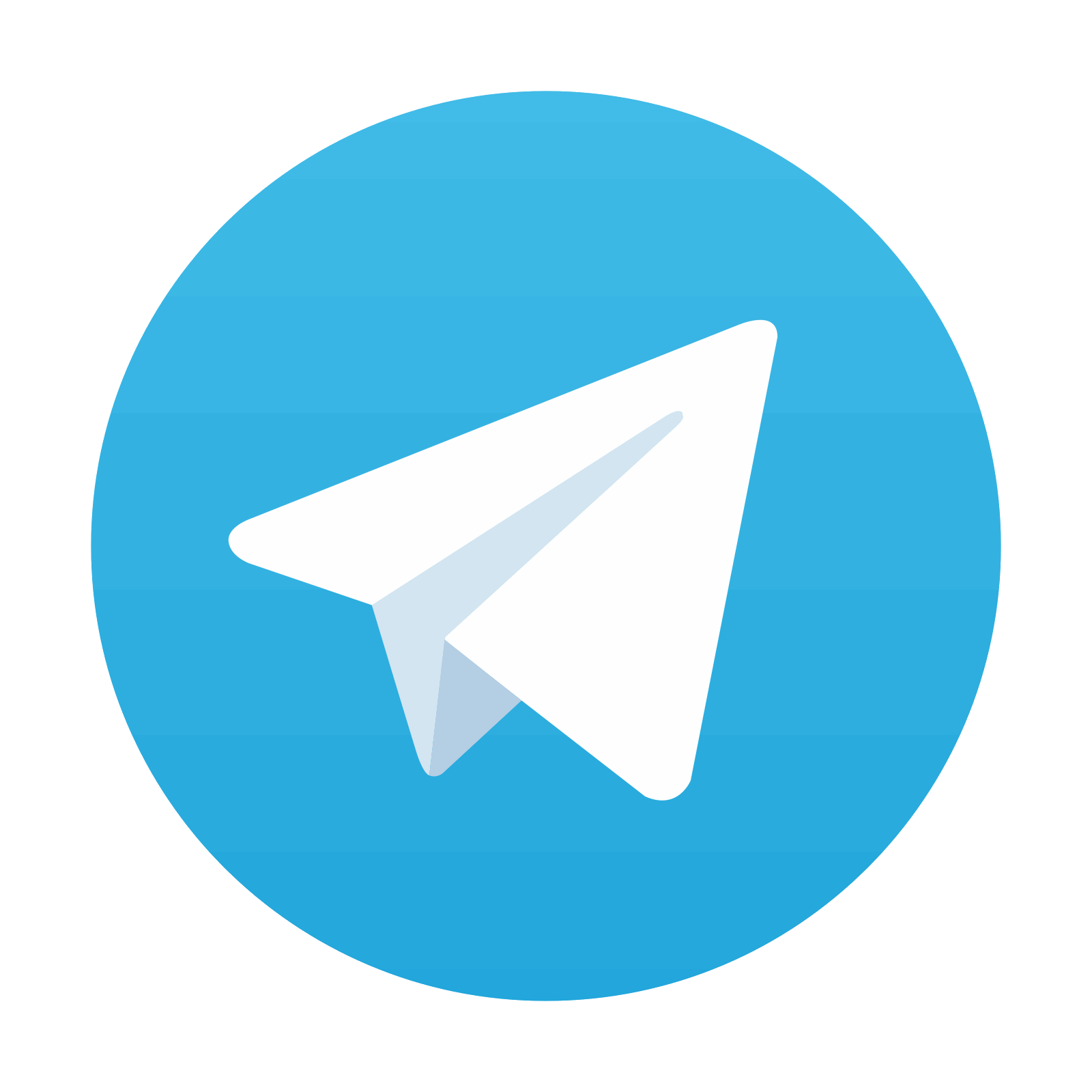
Stay updated, free articles. Join our Telegram channel

Full access? Get Clinical Tree
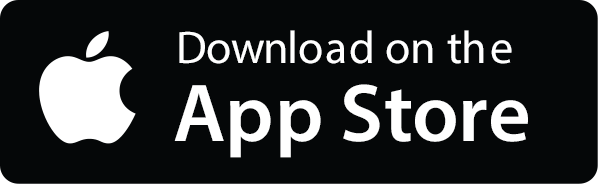
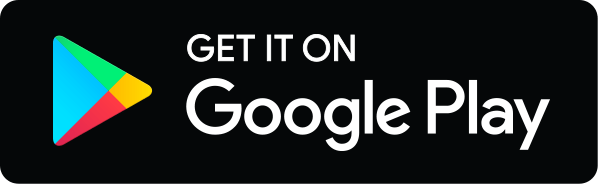
