Acyanotic cardiac defects are a group of diverse cardiac malformations that share a common physiology of increased pulmonary blood flow in the setting of normal systemic perfusion and oxygen saturation. Most of these defects produce varying degrees of shunting of blood from the left side of the heart to the right side. Patients with shunt lesions often present initially to the general pediatrician with problems ranging from failure to thrive and poor weight gain to respiratory distress and exercise intolerance. Alternatively, the patient may be asymptomatic but have an abnormal cardiovascular physical examination. Some of these defects, when left untreated, can affect long-term cardiovascular health. Therefore, this chapter will address the pathophysiology of shunt lesions, modes of presentation, indications for referral, and general treatment algorithms of shunt lesions.
Although specific defects within the group of shunt lesions present at different ages depending on the location and degree of shunting, the common pathophysiology involves increased pulmonary blood flow.
In utero, the placenta provides oxygenated blood to the fetus. The pulmonary vasculature is constricted, and blood flow is largely shunted away from the lungs across the patent foramen ovale and the patent ductus arteriosus. The first breaths of life initiate a sharp decline in pulmonary vascular resistance that then continues for the first few months of life.1 The degree of shunting through some of the shunt lesions, such as patent ductus arteriosus and ventricular septal defect, depend on the relative resistances between the pulmonary and systemic vascular beds. Therefore, infants with these types of shunt lesions present beyond the neonatal period but within the first few months of life when pulmonary vascular resistance is low, promoting more pulmonary blood flow.
In contrast, the degree of shunting in other defects, such as atrial septal defects, depends on right ventricular compliance. The right ventricle is relatively stiff and noncompliant in utero. Unlike the relatively brisk drop in pulmonary vascular resistance after birth, the right ventricle can take months to remodel. Therefore, patients with these types of defects present in late childhood or early adulthood.
Excessive pulmonary blood flow leads to respiratory symptoms of tachypnea and increased work of breathing. The exact mechanism of these symptoms is unknown, but it likely is explained by increased lymphatic flow. As pulmonary blood flow continues to increase, lymphatic channels in the lungs are overwhelmed and interstitial fluid accumulates, leading to peribronchial edema and inflammation.2 Tachypnea and retractions ensue. This increased work of breathing, in turn, makes it difficult to feed well and increases oxygen and caloric demands, leading to poor weight gain and failure to thrive. Unlike adults with low-output heart failure, infants and children with shunt lesions have high-output heart failure. Increased metabolic demand stimulates neurohormonal mechanisms and increases cardiac output. Hepatic veins dilate to accommodate the increased blood volume, and hepatomegaly results. Adrenergic drive is also increased and accounts for the tachycardia and diaphoresis that are often seen.3 An abnormal cardiac physical examination in the setting of these other signs and symptoms is an indication for prompt referral to a pediatric cardiologist (Table 7-1).
Symptoms |
|
Physical examination |
|
Cardiac examination unique to each defect |
|
Chest x-ray |
|
Electrocardiogram |
|
The goals of medical therapy are to minimize respiratory symptoms and optimize growth. Fortified feeds with additional calories and supplemental enteral nutrition with nasogastric feeds can improve weight gain. Close monitoring during these therapies is critical.
Diuretic medications reduce the interstitial fluid that has accumulated in the lungs and can ameliorate the respiratory symptoms. Furosemide is a loop diuretic that acts by inhibiting the sodium-potassium-chloride channel in the thick ascending limb of the loop of Henle. It can be prescribed at an initial dose of 1 mg/kg/dose twice daily and increased as needed, in conjunction with a pediatric cardiologist. Possible side effects of furosemide include dehydration, hypokalemia, and mild hypocalcemia.4 Additional diuretics can be used if this is inadequate therapy or not tolerated by the patient. Chlorothiazide is a thiazide diuretic that inhibits sodium and chloride reabsorption from the distal convoluted tubules. Side effects include dehydration and hypokalemia.4 Spironolactone is a mild diuretic that acts by inhibiting the effect of aldosterone. It is a potassium-sparing diuretic and, thus, can be used in conjunction with other diuretics to prevent hypokalemia. Spironolactone is considered to help with myocardial remodeling in patients with heart failure. Some pediatric cardiologists prescribe digoxin for patients with evidence of ventricular dysfunction and congestive heart failure. There are limited data supporting its use for ventricular remodeling. In some patients, enalapril may be used to decrease systemic afterload, particularly with ventricular septal defects and patent arterial ducts. Enalapril decreases the systemic vascular resistance, resulting in decreased left-to-right shunting in these lesions. This medication is also used to reduce significant left-sided atrioventricular (AV) valve regurgitation (Table 7-2).
Medication | Usage | Mechanism of Action | Dosage | Side Effects |
---|---|---|---|---|
Furosemide | Diuresis | Loop diuretic (inhibits Na-K-Cl channel in thick ascending loop of Henle) | Neonates: 1-4 mg/kg/dose once or twice daily; children: 1-6 mg/kg/d divided twice daily | Hypokalemia, hypocalcemia, nephrocalcinosis, hyponatremia, dehydration, ototoxicity |
Chlorothiazide | Diuresis | Thiazide diuretic (inhibition of sodium and chloride reabsorption from distal convoluted tubule) | 20 mg/kg/d divided twice daily | Hypokalemia, hypochloremic metabolic alkalosis, hyperglycemia, dehydration |
Spironolactone | Potassium-sparing diuretic; cardiac remodeling | Potassium-sparing diuretic (aldosterone inhibition to increase excretion of sodium, chloride, and water) | 1-3 mg/kg/d every 12-24 hours | Hyperkalemia, hypernatremia, hyperchloremic metabolic alkalosis, gynecomastia |
Digoxin | Congestive heart failure; antiarrhythmic | Inhibits ATPase to inhibit conduction through the sinus and atrioventricular nodes; increases influx of calcium into the cellular cytoplasm; increases cardiac parasympathetic activity and arterial baroreceptor activity, which decreases central sympathetic outflow | PO neonates: Load 20-30 μg/kg; maintenance 5-10 μg/kg/d; PO 1 month-2 years: Load 40-60 μg/kg; maintenance 10-12 μg/kg/d; PO 2-5 years: Load 30-40 μg/kg, maintenance 7.5-10 μg/kg/d; PO 5-10 years: Load 20-30 μg/kg, maintenance 5-10 μg/kg/d; PO >10 years: Load 10-15 μg/kg, maintenance 2.5-5 μg/kg/d | Tachyarrhythmia, bradycardia, confusion; diplopia, yellow vision |
Enalapril | Systemic afterload reduction; ventricular remodeling | ACE inhibitor (blocks conversion of angiotensin I to angiotensin II) | Initial: 0.1 mg/kg/d divided twice daily Maintenance: titrate up to 0.5 mg/kg/d | Hypotension, syncope, cough, hyperkalemia, loss of taste reception |
Indomethacin | Closure of a patent ductus arteriosus | Cyclooxygenase inhibitor | <48 hours (all weights) or 2-7 days (<1250 g): time 0, 0.2 mg/kg/dose; 12 hours, 0.1 mg/kg/dose; 24-36 hours, 0.1 mg/kg/dose 2-7 days (>1250 g) or >7 days (all weights): time 0, 12 hours, 24-36 hours, 0.2 mg/kg/dose | Necrotizing enterocolitis, renal failure, thrombocy- topenia, oliguria |
Caloric fortification | Failure to thrive | As needed |
Although it may seem counterintuitive, oxygen therapy can be harmful in these conditions. Oxygen acts as a pulmonary vasodilator, causing a decrease in the pulmonary vascular resistance and leading to increased pulmonary blood flow and worsening of symptoms in the setting of isolated shunt lesions.
If there are enough symptoms to warrant medical management with medication and nutrition, the patient should be followed closely by the primary physician and cardiologist. In cases where the patient does not adequately respond to medical therapy, surgical or catheter-based interventions should be considered as discussed later for individual defects.
The atrial septum develops from a series of invaginations beginning in the fifth week of gestation.5 The septum primum forms during the fifth week of gestation as tissue that grows toward the endocardial cushions.5,6 The space between is called the ostium primum. Small perforations develop in the superior portion of the septum primum, forming the ostium secundum, which allows for flow across the atrial septum in the fetus.5 The septum secundum forms during the seventh week of gestation as tissue that evaginates from the posterior wall of the atrium, leaving a space between the septum primum and secundum called the fossa ovalis5,7 (Figure 7-1). In utero, there is right- to-left shunting through this patent foramen ovale (PFO). Following birth, the increase in pulmonary blood flow results in increased pulmonary venous return to the left atrium. The resultant increased left atrial pressure closes the flap valve of the PFO, eliminating the shunt.5,7
Figure 7-1

Atrial septal anatomy. A. Embryologically, the septum primum (first septum) divides the atrium by migrating to the ventricles. The gap preceding the septal migration is the ostium primum. B. Once the septum primum completes its journey, fenestrations (holes) appear and generally coalesce into a single ostium secundum. C. A second septum (septum secundum) grows down on the right atrial side of the septum primum and covers the entire septal area except for a large oval hole that forms in its distal portion (the foramen ovale). D. If there is redundancy of either the septum primum (as shown) or both septae, then that defines a septal aneurysm. (Reproduced, with permission, from McPhee SJ, Papadakis MA. Current Medical Diagnosis and Treatment 2011. 50th ed. New York, NY: McGraw-Hill; 2011.)
Atrial septal defects (ASDs) are among the more common congenital heart defects, occurring in 1 per 1500 births, with a female preponderance of 2:1 and accounting for 6% to 10% of all cardiac lesions.6,8,9 The classification of ASDs refers to their location within the atrial septum (Figure 7-2). Ostium secundum ASDs are the most common, comprising 80% to 90% of all ASDs. These defects are located in the septum primum. There are a few rare genetic defects associated with these defects. Holt-Oram syndrome is caused by a mutation in the TBX5 gene and is associated with anomalies of the upper extremities and the presence of an ASD.6 An association has also been found between AV conduction delay, noted by PR prolongation on electrocardiogram, and the presence of an ASD that is localized to the NKX2.5 gene. 6
Ostium primum defects are less common, representing 2% to 3% of ASDs.5 Primum defects are located anterior to the fossa ovalis between septum primum and endocardial cushions.6 These defects are along the spectrum of endocardial cushion defects and can be found with an isolated cleft mitral valve or as part of an AV septal defect (see below), where they are often associated with trisomy 21.5,6,10
Sinus venosus ASDs result from incomplete septation between the pulmonary veins, the vena cavae, and the right atrium.5,6 These defects are posterior and superior to the fossa ovalis and are often associated with anomalous pulmonary venous drainage of the right pulmonary veins. They collectively account for 5% to 10% of ASDs.8,11
The rarest type of ASD is the coronary sinus septal defect. This defect results from a lack of septation of the coronary sinus from the left atrium (unroofed coronary sinus), allowing for a communication between the left and right atria via the coronary sinus.5 It is often seen in conjunction with a persistent left superior vena cava.
As previously discussed, the foramen ovale is a normal fetal structure that typically closes after birth. However, autopsy studies have shown that as many as 17% to 35% of adults may have an open or probe-patent foramen ovale.5 These small residual communications may be noted on echocardiograms performed for other reasons. They are not felt to be of any hemodynamic significance and typically do not cause symptoms or changes in the physical examination.6,7 However, patients with a history of an embolic stroke may be evaluated for the presence of a PFO. It is possible that during an episode of transiently increased right atrial pressure, an embolus in the systemic veins could shunt right to left through a PFO (a “paradoxical shunt”), resulting in an embolic event.6
The direction of blood flow across an ASD is determined by the relative ventricular compliances. In the immediate newborn period, the right and left ventricles are both stiff, resulting in little shunting across the defect. As the newborn grows, the right ventricle becomes more compliant than the left ventricle and pulmonary vascular resistance decreases, resulting in increasing left-to-right flow across the ASD.7,12 The increased blood flow across the ASD results in a volume load to the right side of the heart, including the right atrium, right ventricle, and pulmonary arteries.
Most infants with ASDs are asymptomatic, because the left-to-right shunt is not very significant early in life. Infants with genetic syndromes or chronic lung disease of prematurity are 2 groups of patients who are more likely to be symptomatic from an ASD in the first months of life.7 Some infants or young children with an ASD may have frequent respiratory tract infections. But even in childhood, most ASDs do not cause symptoms because the right ventricle tolerates the volume load well.6 Instead, children typically present to the cardiologist because of an abnormal cardiac physical examination.
In infancy, the physical examination is often normal. Children can have a hyperdynamic cardiac impulse and a prominent RV heave, secondary to the volume load on the right side of the heart and right ventricular dilation.6 Auscultation reveals a fixed, widely split S2 and/or a low-frequency systolic ejection murmur at the left upper sternal border from augmented flow across the pulmonary valve. With large shunt volumes, a diastolic rumble may be audible at the left lower sternal border, secondary to the increased volume across the tricuspid valve.6
Chest x-ray may reveal an enlarged cardiac silhouette, a dilated pulmonary artery, and increased pulmonary vascular markings.6,12 These findings are more prominent with a larger left-to-right shunt and increase with age. An electrocardiogram may show evidence of right atrial enlargement with tall, peaked p waves. In addition, an incomplete right bundle-branch block pattern may be seen as an rSR′ pattern in lead V1.6 Most often, patients remain in normal sinus rhythm in the presence of an ASD, although older untreated patients may have significant right atrial dilation from chronic left-to-right shunting with resultant supraventricular tachyarrythmias.6
The mainstay for diagnosis of an ASD is echocardiography. Transthoracic echocardiographic images can demonstrate the size and location of the defect within the atrial septum, as well as the direction of shunt through the defect.13 Transthoracic echocardiography also allows for definition of the pulmonary vein and mitral valve anatomy and assessment of right atrial and right ventricular dilation.6,7,13 Echocardiography serves an important role in determining which ASDs are amenable to catheter-based intervention versus surgical intervention.
ASDs rarely cause symptoms of heart failure in childhood in the absence of other pulmonary or genetic abnormalities. However, if a patient demonstrates poor growth or symptoms of tachypnea and respiratory distress with activity or feeds, diuretics may be warranted6 (see Table 7-2). Subacute bacterial endocarditis (SBE) prophylaxis is not indicated in the setting of an ASD.14
Secundum ASDs can often close spontaneously. Previously published studies report that 92% of defects diagnosed in infancy close before 1 year of age. Defects are most likely to close if they are less than 7 mm in diameter.6,13 Newly diagnosed infants and toddlers are usually followed. If the secundum ASD persists and echocardiography demonstrates volume load to the right side, these patients are usually referred for closure around the age of 3 to 4 years.6 In older children, an ASD is often closed soon after diagnosis if there is evidence for significant right ventricular dilation. The defect should be closed sooner if a patient develops symptoms of congestive heart failure refractory to the medical interventions outlined earlier.6,11 If left untreated, patients are at risk for increasing pulmonary arterial pressures and the development of pulmonary hypertension in the third or fourth decade of life. Pulmonary vascular disease occurs in 5% to 10% of untreated ASDs and is more commonly seen in females.6 There is also a risk of supraventricular tachyarrhythmias secondary to the dilation of the right atrium as the patient ages.6
Transcatheter closure of ostium secundum ASDs has been used with increasing frequency and is now the most commonly used therapy for the appropriate defect. There are multiple catheter devices available, whose description is beyond the scope of this chapter (Figure 7-3). Some ostium secundum ASDs are not amenable to transcatheter closure because of the size of the patient, the size of the defect, or the location of the defect within the atrial septum.9 In these patients, surgical closure is recommended.
Figure 7-3

Two commonly used devices for percutaneous closure of secundum atrial septal defects (ASDs). A. The Amplatzer Septal Occluder attached to its delivery cable. The device is made of an alloy, nitinol, shaped into 2 flat discs and a middle “waist,” with polyester fabric inserts designed to help close the hole and provide a foundation for growth of tissue over the device. B. A frontal fluoroscopic image of the Amplatzer Septal Occluder after deployment. C. The GORE HELEX Septal Occluder is composed of expanded polytetrafluoroethylene patch material supported by a single nitinol wire frame shaped to bridge the ASD. D. A frontal fluoroscopic image of the GORE HELEX Septal Occluder after deployment.
Ostium primum defects, sinus venosus defects, and unroofing of the coronary sinus will not spontaneously close and ultimately require elective surgical intervention. These defects are not amenable to catheter-based intervention.
A PFO rarely causes symptoms or significant right heart dilation and, thus, does not typically require intervention unless it is diagnosed in the context of a cryptogenic stroke.
Both catheter-based and surgical techniques can successfully close nearly all ASDs with a very low rate of serious complications. Nevertheless, there are a few considerations that require attention during the follow-up period after ASD closure. After surgical ASD closure, there is a risk for postpericardiotomy syndrome consisting of accumulation of pericardial fluid and inflammation. Symptoms of respiratory distress, fever, fatigue, or emesis within the first weeks after intervention should prompt an evaluation for pericardial effusion.9,12 Typically, this complication is treated with anti-inflammatory medication or steroids. In rare cases, pericardial fluid drainage is required. Patients who undergo repair of sinus venosus ASDs are at increased risk of sinus node dysfunction, superior vena cava obstruction, and pulmonary venous obstruction.11 In addition, patients are at risk of developing supraventricular tachyarrhythmias, including atrial fibrillation, a risk that increases with age at closure.9,15
After catheter-based intervention, there is a risk of device embolization, which usually occurs in the first 24 hours after the procedure.6,9 There is also a risk of thrombus formation on the device, with an incidence of 1.2%.9 Commonly, anticoagulation with aspirin therapy is recommended for at least 6 months after device placement. Some patients develop AV conduction delay or atrial ectopy that typically resolves in the days to weeks after device placement.9 Finally, there have been rare isolated reports of late device embolization and device erosion through the atrial or aortic wall.9 Patients require 6 months of SBE prophylaxis after device placement (Table 7-3).
Medical management | Asymptomatic: None Symptomatic: Diuretics, caloric fortification | |
Indications for intervention | Elective ASD closure in childhood (after age 3-4 years) if asymptomatic with evidence of right-sided volume load by examination or echocardiogram; earlier intervention if symptomatic | |
Treatment options | Surgical closure via midline sternotomy | Catheter-based device closure (depends on location of defect and size of patient) |
Postintervention issues | Pericardial effusion, arrhythmias, residual ASD | Residual ASD; small risk of device embolization, atrial arrhythmias, disruption of adjacent structures (SVC/pulmonary vein obstruction, etc), and thrombus |
Follow-up | Frequent assessments after surgery and continued cardiology follow-up if there is a residual defect or if there are associated defects | Aspirin and endocarditis prophylaxis for first 6 months after procedure; yearly follow-up with cardiologist |
The ventricular septum is a complex structure that forms through a sequence of events in the fetus between 4 and 7 weeks of gestation and involves fusion of several tissues including endocardial cushion–derived mesenchyme, primary atrial septum, and muscular components of atrial and ventricular septae.16 The muscular intraventricular septum forms by infolding of the ventricular muscle within the primitive cardiac tube. This muscular septum aligns with the conal septum, which is positioned between the 2 outflow tracts.16 Lastly, the membranous septum closes, which is adjacent to the anteroseptal commissure of the tricuspid valve.17 Ventricular septal defects (VSDs) can occur anywhere in the septum.
VSD accounts for 20% of congenital heart disease, making it the most common form of congenital heart disease after bicuspid aortic valve.18 The incidence of isolated VSDs has increased over time, but this increase may be related to improved ultrasound technology and increased diagnosis in the prenatal and antenatal periods. There is a slight female predominance of 56%.18 More than 75% of small defects close within the first 2 years of life.17,19 VSDs are the most common heart defect in many chromosomal anomalies, including patients with trisomies 13, 18, and 21.18 VSDs are also associated with Holt-Oram syndrome.
VSDs are classified according to their location within the ventricular septum (Figure 7-4). Spontaneous closure rate and the presence of associated defects depend on the defect type. Conoventricular defects account for 80% of all VSDs.18,19 Other terms include membranous or perimembranous defects. These VSDs lie between the outlet and inlet portion of the right ventricle, adjacent to the tricuspid valve and the aortic valve. These defects can be restricted by overlapping tricuspid valve tissue or by prolapse of the aortic valve cusp into the defect.19 Some smaller defects can close spontaneously.
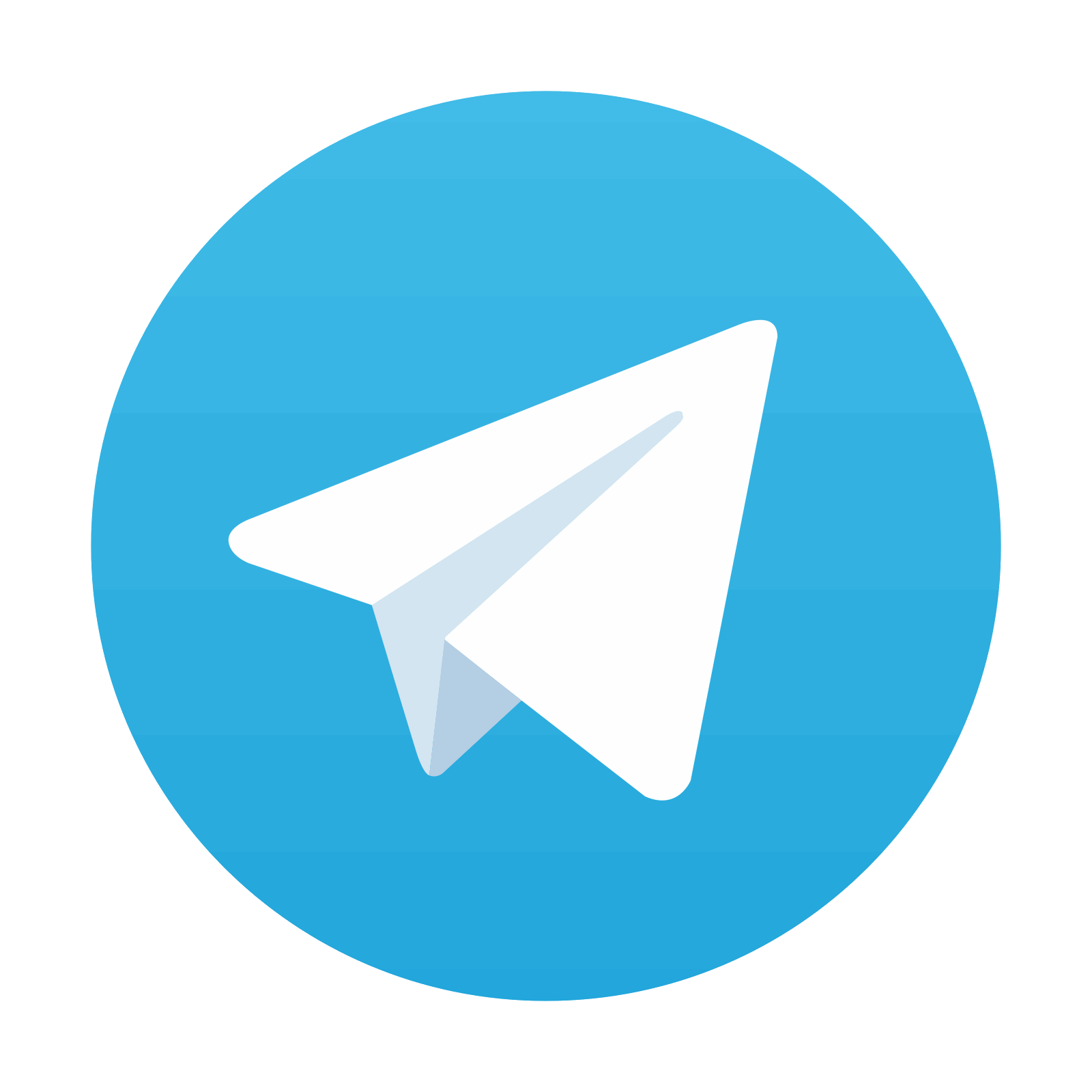
Stay updated, free articles. Join our Telegram channel

Full access? Get Clinical Tree
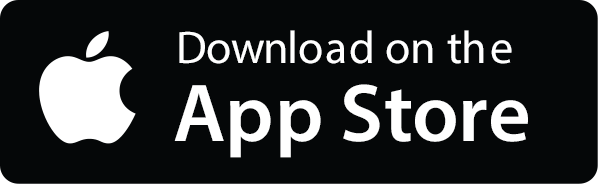
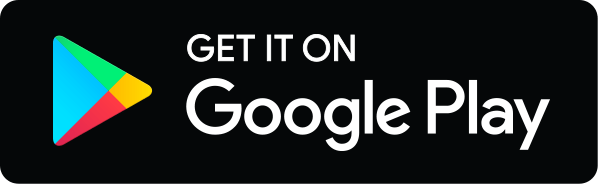