Various etiologies of lung injury in the immunocompromised patient, all with the potential to contribute to severe PARDS
While sending cultures, in theory, seems simple, in practice, the decision to perform a bronchoscopy with bronchoalveolar lavage (BAL) can be challenging. There is a heightened concern for the risk of complications associated with bronchoscopy including bleeding, pneumothorax, hypoxemia, and new requirement for mechanical ventilation [13].
Despite the risk of complications, establishing a diagnosis can alter therapy and potentially improve outcomes. There is a wide range of cited diagnostic yield from a BAL in the immunocompromised population, anywhere from 27% to 85% [14–16]. In a large study of children post-HCT, the diagnostic yield of BAL was as high as 67.9% [17]. The diagnostic yield is also likely to improve with time as new laboratory techniques are being investigated to identify various organisms [18]. There does seem to be an improved diagnostic yield if the BAL is combined with transbronchial biopsy, but this technique is rarely employed in children and brings a higher risk for complications [14, 15, 19]. Studies in organ transplant recipients have found that, anywhere from 20% to 70% of the time, the results of a BAL lead to a change in therapy [20]. Even more importantly, if therapy is changed, there is the possibility to reduce mortality by 20% [21], although other studies have found no effect on mortality [16, 22]. Unfortunately, much of the data are conflicting, and the range of benefit is wide.
The risk of complications of bronchoscopy with BAL may be higher in the immunocompromised population. Complications such as bleeding in a population with low and potentially dysfunction platelets should be a concern for all clinicians and bronchoscopists involved in the care. In immunocompromised adults, the bronchoscopy complication rate is up to 21% and is even higher with transbronchial biopsy [14]. In children with cancer, the complication rate associated with bronchoscopy has been cited at 30% [23]. However, most of these complications are relatively minor and resolve without intervention. The larger concern, early in the course of respiratory distress, is the development of sustained hypoxemia and a new mechanical ventilation requirement. In various adult immunocompromised populations, there has been <1% increased risk in requiring intubation following bronchoscopy [24, 25]; however, this risk is increased in the post-HCT patient [16].
The conflicting data between benefit and the risk of complications from bronchoscopy leaves clinicians wondering if the risk is worth the benefit. There does seem to be data supporting early bronchoscopy with BAL. In a study of 501 adults post-HCT, the diagnostic yield was best if the BAL was completed within the first 24 hours of respiratory symptoms [26]. Because these patients are immunocompromised and at such high risk for infection, empiric antibiotics are frequently started. The use of antibiotics can affect the results of the BAL. Pediatric and adult studies have shown that the diagnostic yield of BAL decreases significantly following 72 hours of antibiotics [27, 28]. There is also data demonstrating a lower risk of complications when bronchoscopy is performed earlier in the course of respiratory distress [29, 30].
Chest Imaging
When considering imaging in an immunocompromised child with acute hypoxic respiratory failure, a chest radiograph (CXR) is often first in the diagnostic workup. This is especially true in the setting of fever and cough, when the clinical suspicion for pneumonia is high. If the radiograph identifies a focal infiltrate, further imaging with computerized tomography (CT) is likely not necessary. Empiric antibiotics can be started and decisions can be made about obtaining a BAL. In the setting of a normal CXR or a diffuse infiltrative process, a CT may offer useful diagnostic information. CXRs can be negative or unhelpful in the immunocompromised population despite clinical concern [31]. The CT can allow for better visualization of the lung parenchyma, offer considerations for a differential diagnosis of the underlying lung pathology, or help to identify a specific area for lung biopsy [32].
Respiratory Support for the Immunocompromised Patient
Noninvasive Respiratory Support
Summary of studies investigating noninvasive respiratory support in immunocompromised patients
Author/Year | Study design | Study population | Main findings |
---|---|---|---|
Adult studies | |||
Antonelli et al. 2000 [37] | RCT: NIV vs. supplemental O2 | 40 adults post-solid organ transplant | NIV had improvement in PaO2:FiO2, had a 20% intubation rate compared to 70% for supplemental O2, a shorter length of hospital stay (5 vs. 9 days) and a lower mortality (20% vs. 50%) |
Hilbert et al. 2001 [38] | RCT: Early NIV vs. supplemental O2 | 52 IC adults | NIV had lower intubation rate (46% vs. 77%) and lower ICU mortality (38% vs. 69%) |
Squadrone et al. 2010 [40] | RCT: Early CPAP vs. Venturi mask | 40 adults with hematologic malignancies | Those treated with early CPAP were less likely to be admitted to the ICU, less likely to be intubated and more likely to survive |
Azoulay et al. 2014 [45] | Retrospective multicenter cohort | 1004 adults with cancer and ARDS | 387 patients were treated with NIV with a failure rate of 71%, and NIV failure was an independent risk factor for mortality |
Lemiale et al. 2014 [46] | Post hoc of RCT | 211 adults with cancer | 139 patients treated with NIV, 38% failed NIV. NIV failure was not associated with higher mortality |
Lemiale et al. 2015 [33] | RCT: HFNC vs. Venturi mask | 100 IC adults | At 2 hours there was no difference in the rate of intubation, work of breathing, tachypnea, tachycardia, or in perceived patient comfort |
Lemiale et al. 2015 [39] | RCT: NIV (BIPAP and HFNC) vs. supplemental O2 | 374 IC adults | There was no difference in intubation or mortality between the two groups |
Harada et al. 2016 [35] | Retrospective cohort study | 56 adults with hematologic disease | There was an 80% failure rate of HFNC. Those who failed required mechanical ventilation or changed to a palliative care approach. HFNC was well tolerated. Pneumonia was a risk factor for failure |
Lemiale et al. 2017 [34] | Post hoc of RCT: HFNC vs. supplemental O2 | 127 IC adults | No difference in rate of intubation or mortality |
Pediatric studies | |||
Pancera et al. 2008 [42] | Retrospective cohort study | 120 children with cancer | There was a 26% failure rate of NIV and hemodynamic instability was a significant risk factor for NIV failure |
Piastra et al. 2009 [43] | Prospective observational study | 23 IC children | There was a 45% failure rate and of those that failed NIV 80% died |
Murase et al. 2012 [44] | Observational study | 92 children post liver transplant | NIV was used in 47 children postextubation. Those treated with NIV had a lower rate of reintubation (6.4% vs. 23.4%) and were discharged from the PICU faster |
Rowan et al. 2016 [3] | Retrospective multicenter cohort | 222 children post-HCT | 91 children treated with NIV prior to intubation. Those treated with NIV prior to intubation had an increased risk of mortality compared to those who were intubated directly (OR: 2.1, 95% CI: 1.2–3.6) |
Noninvasive positive pressure ventilation (NIV) has been used in this patient population. In theory, the application of NIV can reduce work of breathing, decrease the inspiratory load, aid in lung recruitment, and improve oxygenation. In a large randomized controlled trial of general adults, the development of ARDS was associated with NIV failure and the need for intubation [36]. More specific to the immunocompromised population, a promising small, randomized controlled trial was conducted in 40 adults post-solid organ transplantation [37]. The patients were randomized to either NIV or supplemental oxygen. Those randomized to NIV had an improvement in oxygenation, were less likely to get intubated (20% vs. 70%), had a shorter length of hospital stay (5 vs. 9 days), and a lower mortality rate (20% vs. 50%). A year later, another small trial randomized 52 immunosuppressed adults early in the course of respiratory distress to either NIV or supplemental O2 [38]. In this study, those placed on NIV had a decreased rate of intubation and a lower ICU and hospital mortality [38], suggesting that early application of NIV can be beneficial in the immunocompromised adult. Promising results from these two small studies led to a large multicenter study that included 374 immunocompromised adults randomized to either NIV or supplemental O2, but there was no difference in the rate of intubation or mortality between the groups [39]. Early use of continuous positive airway pressure (CPAP) has also been investigated in 40 adults with hematologic malignancies randomized to early CPAP or supplemental O2 via a Venturi mask [40]. Patients randomized to CPAP were less likely to be admitted to the ICU, less likely to need intubation, and more likely to survive [40].
In the general pediatric population, there is a high failure rate of NIV when PARDS is present [41]. While there are no randomized controlled trials, there are studies describing the use of NIV in children with immunocompromised conditions. In a retrospective study of 239 pediatric patients with cancer, 120 children were treated with NIV [42]. Of these, 25.8% failed NIV and required intubation. Hemodynamic instability was a significant risk factor for NIV failure. In 2009, Piastra et al. published an observational study of 23 consecutive immunocompromised children treated with NIV [43]. Of these children, 45% failed NIV and required intubation, and of those requiring intubation, only 20% survived. In 2012, Murase et al. published a report on the use of NIV post-liver transplantation to prevent reintubation. Of the 92 patients, NIV was used in 47. Those treated with NIV had a lower rate of reintubation (6.4% vs. 23.4%) and were discharged from the PICU faster [44]. More recently, a multicenter study of 222 children post-HCT found that children who were placed on NIV prior to intubation had two times the odds of mortality [3]. However, this study had limitations because it did not include children who were successfully treated with NIV. In a multicenter study of 1004 adults, 30% were treated with NIV [45]. Of these, 70% failed NIV and failure was independently associated with a higher mortality. Another study of adults with cancer found no difference in mortality for those who received invasive mechanical ventilation as their first-line respiratory support compared to those treated with NIV prior to intubation [46]. Although those who failed NIV had a higher mortality of 65.3% compared to those who were intubated first with a mortality of 50%, this finding did not meet statistical significance. In summary, the use of NIV may improve outcomes, particularly if used early in the course of respiratory distress. In 2015, the pediatric acute lung injury consensus conference (PALICC) specifically recommended that immunocompromised children may benefit from an early trial of NIV in an attempt to avoid intubation [41], but care should be taken not to delay intubation. This same group also states that NIV is not recommended for severe disease, which may limit applicability to many immunocompromised patients. Furthermore, NIV is likely not a good support modality for those with hemodynamic instability or multiorgan dysfunction.
Invasive Mechanical Ventilation
While there are multiple studies in both pediatrics and adults that discuss ICU outcomes and critical care interventions that are associated with outcomes, there is very little data regarding how to specifically manage the ventilator in the immunocompromised patient. It is clear that the immunocompromised patient is at high risk for the development of PARDS; it happens early and it is generally severe [9]. In fact, many immunocompromised children have significant hypoxia at the time of PICU admission [47]. The early institution and focus on lung-protective ventilation strategies is essential. General adult ARDS data demonstrate improvement with protective strategies that focus on low tidal volume, limitation of plateau and driving pressures, and reliance on high PEEP to reduce the delivered FiO2 [48–50].
PALICC gave recommendations for mechanical ventilation strategies in the general PARDS patient [51]. The first recommendation, derived from adult data, recommends limitations of tidal volume to ≤8 ml/kg. Tidal volume goals have not been rigorously studied in pediatrics, let alone in children with immunocompromised conditions. Some observational studies in children with PARDS, including some with immunocompromised conditions, demonstrated improved survival in those who were ventilated with higher tidal volumes [6, 52]. In a larger retrospective study of pediatric HCT patients requiring mechanical ventilation, the median tidal volume utilized was approximately 7 ml/kg and tidal volume was not associated with survival [47].
Inspiratory pressures have also been investigated. PALICC recommended limitation in inspiratory pressures with a goal to maintain plateau pressures at or below 28 cmH2O [51]. High inspiratory airway pressures are associated with mortality in children with PARDS [6, 52], and a cohort of 222 pediatric HCT patients found similar results. A peak inspiratory pressure (PIP) > 31 cmH2O was independently associated with mortality [47], and this association was cumulative, with increasing PIP being associated with increasing odds of mortality.
High levels of peak end-expiratory pressure (PEEP) are likely needed to maintain lung recruitment and prevent atelectrauma. While not investigated in pediatric clinical trials or in the isolated immunocompromised population, data extrapolated from adults suggest that increased PEEP in severe ARDS is associated with lower hospital mortality [53, 54]. PALICC recommended that high levels of PEEP may be needed in severe PARDS [51]. The unique consideration in the immunocompromised population is that these children are at risk for noninfectious lung disease, such as bronchiolitis obliterans, that can lead to pulmonary fibrosis and nonrecruitable lung [55, 56]. Therefore, the clinician must carefully consider the underlying etiology and the potential for lung recoverability when applying increasing levels of PEEP. Early in the presentation, often before a clear diagnosis is made, the application of high levels of PEEP in the immunocompromised child with severe PARDS is likely warranted and may provide clue to the clinician as to the recruitability of the lung. However, it is unclear if this high PEEP strategy is actively being applied to children with immunocompromised conditions. In a retrospective twelve-center cohort of children post-HCT, many of which had severe PARDS, use of PEEP was modest in the first five days of mechanical ventilation, with median levels ranging between 7 and 9 cmH2O for both survivors and nonsurvivors [47]. When investigating the use of PEEP compared to the use of FiO2 in this same cohort, there seemed to be more reliance on higher FiO2. A high PEEP/low FiO2 strategy is associated with survival in adults [57]. In a general pediatric study, failure to comply with this PEEP strategy was associated with PARDS mortality [58]. This was also demonstrated in a pediatric HCT cohort with respiratory failure. While there was very little compliance with this strategy in the first few days of mechanical ventilation, compliance with this strategy was associated with improved survival [47].
The classification of PARDS severity determined by PALLIC was associated with increasing mortality and morbidity in the most severe immunocompromised children (the child post-HCT) [9]. This is not surprising with the use of oxygenation index (OI) and/or oxygen saturation index (OSI) as the foundation for classifying PARDS severity. OI, OSI, and PaO2/FiO2 are highly associated with mortality. In pediatric lung injury, OI is associated with mortality and was found to be an even better predictor of mortality in the immunocompromised [59]. In a single-center cohort of pediatric HCT patients, increasing OI was associated with an increasing risk for mortality [60]. This was also demonstrated in U.S. and European multicenter cohorts of children post-HCT [47, 61]. In the US study, controlling for other variables, OSI was independently associated with increasing mortality. With OSI levels consistent with severe PARDS, the odd for morality increased (OR = 11.1, p < 0.0004) [47]. Similarly, the European study found cumulative OI to be highly associated with mortality [61].
Nonconventional Mechanical Ventilation
There is limited data to support or negate nonconventional mechanical ventilation use in the immunocompromised child. Because these children can develop severe PARDS, high-frequency oscillatory ventilation (HFOV) and airway pressure release ventilation (APRV) may be considered as support modalities. In a multicenter study of HFOV use in children, those who were immunocompromised had the highest mortality, and an OI >35 had the best predictive power for HFOV-related mortality in this group [62]. A small single-center study of 12 children with cancer or post-HCT found that the use of HFOV can improve gas exchange. Seven of these 12 patients survived to ICU discharge [63]. A larger single-center study of 60 immunocompromised children was published, describing the use of HFOV and APRV [64]. In this cohort, the overall mortality was 63%. Improvements in oxygenation as measured by PaO2/FiO2 or OI at 24 hours on the nonconventional mode of ventilation were associated with survival. Recently, a larger multicenter cohort of 85 children post-HCT with severe PARDS who were treated with HFOV demonstrated a PICU survival of only 23.5%. This study suggested that earlier HFOV, within the first 2 days of invasive mechanical ventilation, may have a survival benefit [65]. Survivors were transitioned earlier (day 0 vs. day 2, p = 0.002). Also, no one who was transitioned after 1 week of mechanical ventilation survived.
Extracorporeal Membrane Oxygenation
Extracorporeal membrane oxygenation (ECMO) is being increasingly considered in the immunocompromised population, but its benefits remain unclear. Studies have consistently found that immunocompromised status is a significant risk for ECMO mortality [66–69]. Despite this, several case reports exist to support the successful use of EMCO in the immunocompromised population. In 2009, Gow et al. published data from the extracorporeal life support organization on 107 children with malignancies [70]. The majority of children were placed on EMCO for pulmonary support. Survival to hospital discharge was 35%. A study of 14 adults with hematologic malignancies and acute respiratory failure found 50% survival to hospital discharge [71]. There were five major bleeding episodes in this cohort. A similar survival of 44% was found in a pediatric cohort of 14 neutropenic patients with malignancy [72]. The bleeding complication was high at 55%. In a recent cohort of adults post-HCT, the overall ECMO survival was 19% [73]. Survival was only 4% if the patient was within 240 days posttransplant. The authors concluded that the very high mortality rate does not support the use of ECMO in adults within 240 days of HCT. While it is likely that certain immunocompromised children would benefit from ECMO support, certain subsets, such as those post-HCT, have very poor survival. Significant and critical discussions, including prognosis of the underlying immunocompromised condition, bleeding risk, and anticipated time until bone marrow recovery, are necessary prior to deciding to place an immunocompromised child on EMCO for PARDS.
Conclusion
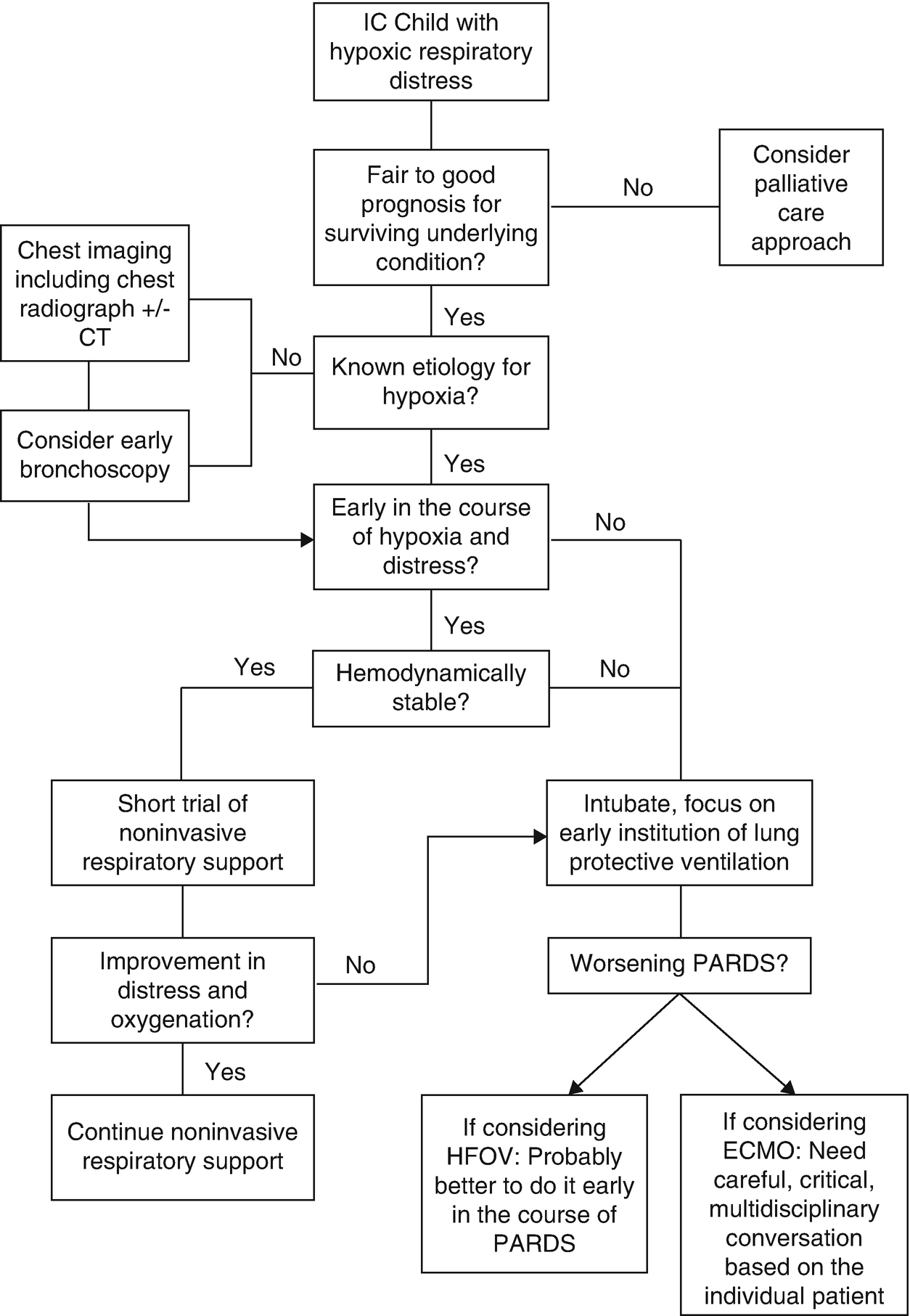
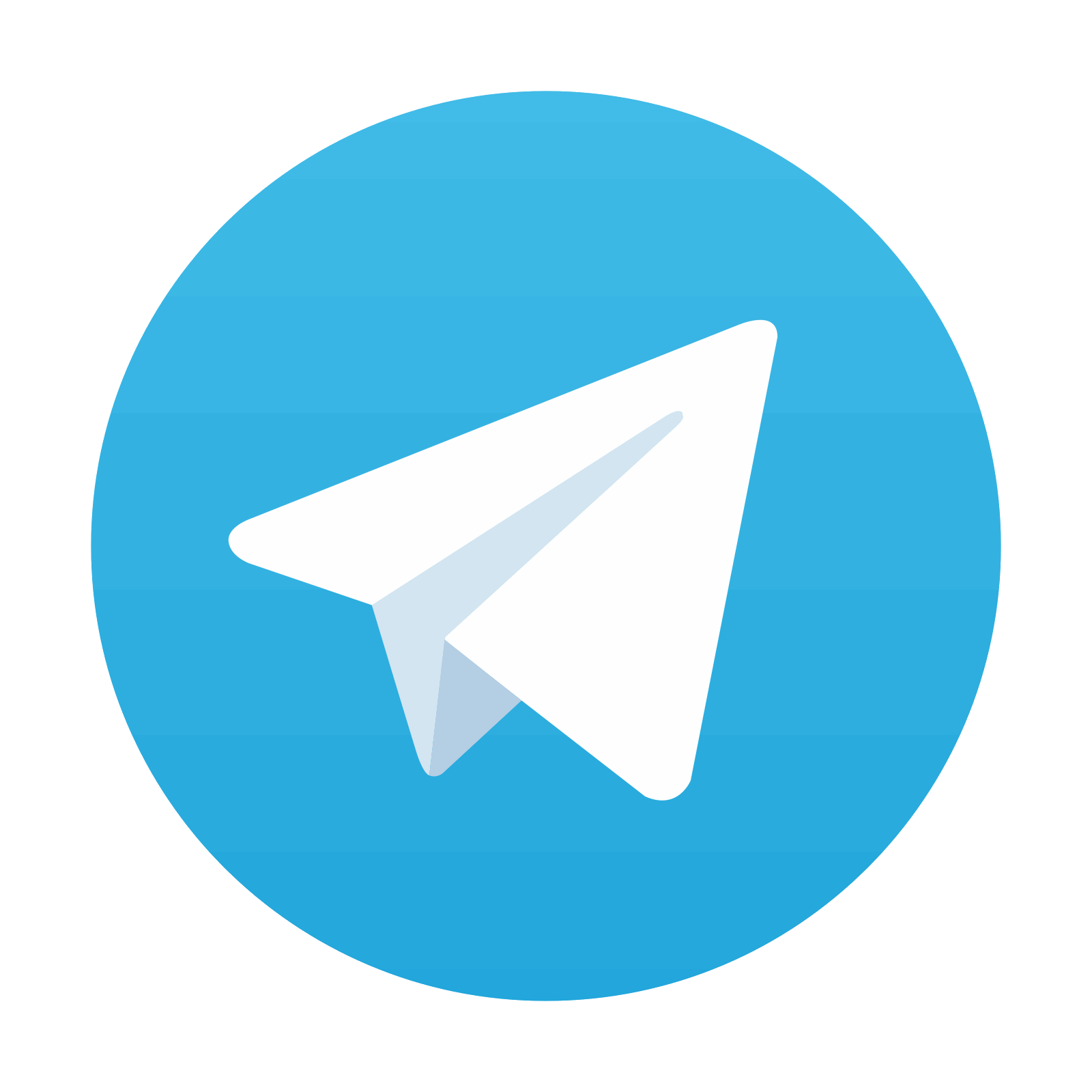
Stay updated, free articles. Join our Telegram channel

Full access? Get Clinical Tree
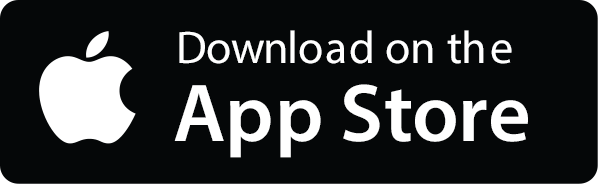
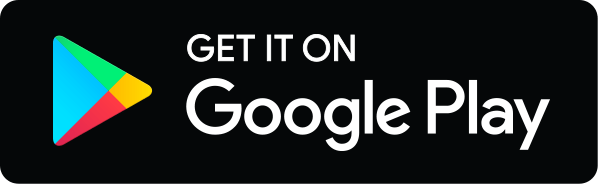