Chapter 36 Acute Respiratory Distress Syndrome
ARDS usually occurs in previously healthy people. Characteristically, there is a latent period between the insult and the development of the full-blown clinical syndrome, which usually is 18 to 24 hours in duration. After this interval, tachypnea, labored breathing, and cyanosis are observed, and arterial blood gas analysis confirms hypoxemia. The abnormalities in lung mechanics and oxygenation are assessed once the patient is intubated and receiving mechanical ventilation. The chest radiograph classically shows diffuse, bilateral, interstitial alveolar infiltrates (Figure 36-1). Resolution of the infiltrates, if it occurs at all, is much slower than with cardiogenic pulmonary edema.
Pathophysiology, Histopathology, and Etiology
The loss of epithelial integrity in ARDS has several consequences. First, under normal conditions, the epithelial barrier is much less permeable than the endothelial barrier; thus epithelial injury can contribute to alveolar flooding. Second, the loss of epithelial integrity and injury to type II cells serve to disrupt normal epithelial fluid transport, impairing the removal of edema fluid from the alveolar space. Third, injury to type II cells reduces the production and turnover of surfactant. Fourth, loss of epithelial barrier can lead to sepsis in patients with bacterial pneumonia. Finally, in severe alveolar epithelium injury, pulmonary fibrosis can develop. Independent of the clinical disorders associated with ARDS (Box 36-1), it is useful to think of the pathogenesis of ARDS as a result of two different pathways: a direct insult to lung cells and an indirect insult occurring as a result of an acute systemic inflammatory response. The host’s inflammatory response to the initial direct (pulmonary) or indirect (nonpulmonary) insult is a key factor in determining the development and progression of the acute injury to the lung. Despite ongoing elucidation of the role of cellular and humoral components of the inflammatory responses in the lung, the precise sequence of events leading to lung damage is still unknown. As with any form of inflammation, ALI during ARDS represents a complex process in which multiple cellular signaling pathways can propagate or inhibit damage to the lung.
Box 36-1
Most Common Clinical Disorders Associated with Development of Acute Respiratory Distress Syndrome (ARDS)
Definition, Incidence, and Severity
In an attempt to overcome some of these problems, Murray and colleagues proposed an expanded definition of ARDS that takes into account various pathophysiologic features of the syndrome. Their definition uses a “lung injury score” (LIS) to characterize the acute pulmonary damage by considering four components: assessment of the chest radiograph, degree of hypoxemia (determined as the ratio of arterial partial pressure of oxygen to the fraction of inspired oxygen, PaO2/FIO2), level of PEEP, and the value of lung compliance, when available (Table 36-1). The final injury score is obtained by dividing the total score by the number of components that were used. A score of 0 indicates no lung injury, a score of 1 to 2.5 indicates mild to moderate lung injury, and a score greater than 2.5 indicates severe lung injury or ARDS. The LIS is not specific for ARDS, however, and has not been validated, because it is not clear whether patients with identical LIS have similar degrees of lung injury. Furthermore, patients with a major component of cardiogenic edema may be mislabeled as having ARDS, and a postoperative patient with moderate atelectasis and mild fluid overload may fit the LIS criteria for ARDS.
Table 36-1 Lung Injury Scoring System
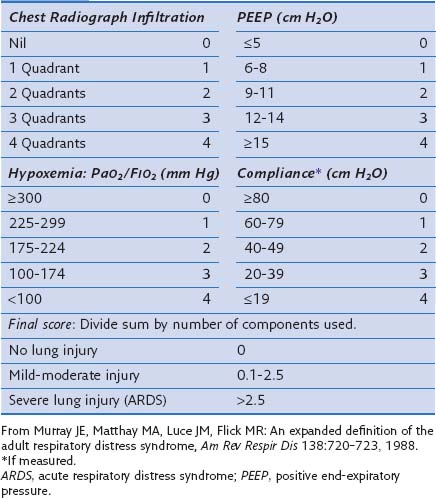
According to these guidelines, ALI exists when the PaO2/FIO2 ratio is 300 mm Hg or less regardless of the level of PEEP and FIO2, and ARDS is present when the PaO2/FIO2 ratio is 200 mm Hg or less regardless of the PEEP setting and FIO2 (Box 36-2). Although this definition formalized the criteria for the diagnosis of ARDS and is simple to apply in the clinical setting, it has been challenged over the years in several studies. Such definitions have limitations: The physiologic thresholds do not require standardized ventilatory support, and the use of PEEP can improve oxygenation indices sufficiently to convert the patient’s status from meeting the definition of ARDS to not meeting the ARDS definition and also can change the physiology in the lung such that the patient does not meet the criteria for ARDS. Therefore, the ARDS criteria may be met when the PaO2 is measured with zero PEEP but not when measured at a PEEP of 5 or 10 cm H2O, making patient comparisons difficult. Furthermore, most of the randomized controlled studies did not use the same definition for ARDS, nor did they evaluate the same ventilatory approaches. Diversity among ARDS definitions is apparent in a large number of studies (Table 36-2).
Table 36-2 Definitions of Acute Respiratory Distress Syndrome in Several Published Reports
Published Study | Criteria |
---|---|
Montgomery et al: Am Rev Respir Dis 132:485–489, 1985 | PaO2/FIO2 <150 mm Hg |
PCP <18 mm Hg | |
Villar et al: Am Rev Respir Dis 140:814–816, 1989 | PaO2 ≤75 mm Hg on FIO2 ≥0.5 |
PCP <18 mm Hg | |
Bone et al: Chest 96:849–851, 1989 | PaO2/FIO2 ≤150 mm Hg (with ZEEP) |
OR PaO2/FIO2 ≤250 mm Hg with PEEP | |
PCP ≤18 mm Hg | |
Amato et al: N Engl J Med 338:347–354, 1998 | Lung injury score ≥2.5 and PCP <16 mm Hg |
Stewart et al: N Engl J Med 338:355–361, 1998 | PaO2/FIO2 <250 mm Hg on PEEP of 5 cm H2O |
Brochard et al: Am J Respir Crit Care Med 158:1831–1838, 1998 | Lung injury score >2.5 |
Villar et al: Intensive Care Med 25:930–935, 1999 | PaO2/FIO2 ≤150 mm Hg on PEEP ≥5 cm H2O |
ARDSNet: N Engl J Med 342:1301–1308, 2000 | AECC |
Gattinoni et al: N Engl J Med 345:568–573, 2001 | PAO2/FIO2 ≤200 mm Hg on PEEP ≥5 cm H2O |
PCP ≤18 mm Hg | |
Villar et al: Crit Care Med 34:1311–1318, 2006 | PAO2/FIO2 ≤200 mm Hg on PEEP ≥5 cm H2O and FIO2 ≥0.5 |
Meade et al: JAMA 299:637–645, 2008 | PAO2/FIO2 <250 mm Hg |
Mercat et al: JAMA 299:646–655, 2008 | PAO2/FIO2 ≤200 mm Hg PCP ≤18 mm Hg |
AECC, American-European Consensus Conference; PCP, pulmonary capillary pressure; PEEP, positive end-expiratory pressure; ZEEP, zero PEEP.
Genetics
In case-control studies of patients with severe sepsis and ARDS, several investigators have explored variants of the gene encoding the lipopolysaccharide-binding protein (LBP) and serial measurements of the LBP in serum to relate them with risk gene variants. It has been reported that (1) a four-SNP risk haplotype of the LBP gene is associated with mean serum LBP concentrations within the first week of the disease process; (2) LBP levels at 48 hours are much higher in patients with ARDS than in those with ALI; and (3) a subsequent increase of LBP levels at 48 hours is associated with a four-fold increase in mortality rate. A positive association with ARDS susceptibility and/or outcome has been reported for several other genes, including surfactant pulmonary-associated protein B (SFTPB), angiotensin-converting enzyme (ACE), tumor necrosis factor (TNF), vascular endothelial growth factor (VEGF), IL-10, pre-B cell–enhancing factor (PBEF), chemokine CXC motif ligand 2 (CXCL2), mannose-binding lectin-2 (MBL2), myosin light chain kinase (MLCK), nuclear factor κ light polypeptide gene enhancer in B cells (NFKB1), coagulation factor V (F5), and type 2 deiodinase (DIO2) (Table 36-3). These genes are involved mainly in the response to external stimulus and cell signal transduction.
Table 36-3 Positive Genetic Association Studies With Susceptibility to and/or Outcome of Acute Respiratory Distress Syndrome (ARDS)*
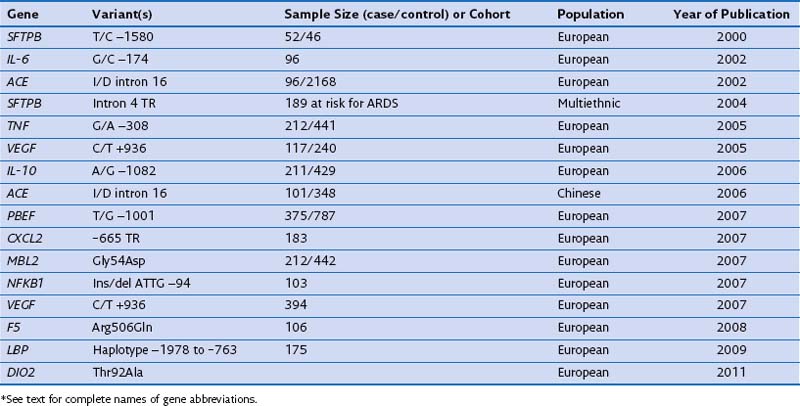
Ventilator-Induced Lung Injury
Ventilators are intended to deliver air or oxygen at tidal volumes and frequencies sufficient to provide adequate alveolar ventilation, to reduce the work of breathing, and to enhance oxygenation (see also Chapter 32). However, mechanical ventilation is a nonphysiologic process, and complications are associated with its application, including increased risk for pneumonia, impaired cardiac performance, and lung injury. During mechanical ventilation, pressures, gas volumes, ventilatory rates, and concentrations of inspired oxygen often are applied at levels that exceed those normally experienced by healthy lungs during spontaneous breathing. VILI is not a new concept—it is the designation historically applied to macroscopic injuries associated with alveolar rupture due to overdistention resulting from application of high inspiratory pressures. Clinical manifestations include interstitial emphysema, pneumothorax, pneumomediastinum, and pneumoperitoneum. The concept of VILI has shifted somewhat from pressure-induced (really volume-induced) injury to increased vascular permeability, accumulation of lung fluid, “atelectrauma,” and inflammation induced by mechanical ventilation.
In 1998 Tremblay and Slutsky coined the term biotrauma to describe the pulmonary and systemic inflammatory response triggered by lung cell distention, alveolar disruption, and/or necrosis after the application of mechanical ventilation. Although a Consensus Conference in 1994 recommended that plateau pressure generally should be limited to 35 cm H2O, little change in ventilator practice occurred until publication of an ARDS Network study demonstrating that a lung-protective strategy using a tidal volume of 6 mL/kg of predicted body weight and moderate levels of PEEP decreased mortality in patients with ALI. This study confirmed that VILI was not just an interesting experimental entity but also was an important clinical entity. This recognition led to the widespread, albeit not universal, use of lung-protective strategies in patients with ALI. Unequivocal evidence from both experimental and clinical data has proved that mechanical ventilation can cause or aggravate ALI. Many of the pathophysiologic consequences of VILI mimic those of ARDS. A number of specific forms of injury caused by the trauma of mechanical ventilation have been identified: barotrauma, volutrauma, atelectrauma, and biotrauma. Current experimental and clinical evidence supports a link between VILI and the development of extrapulmonary organ dysfunction, by mechanisms similar to those whereby most severe cases of sepsis are clinically manifested (Figure 36-2).
Pathophysiology of Ventilator-Induced Lung Injury
Lung volumes for all mammals scale with a common function based on body mass. In spontaneously breathing mammals, tidal volumes are approximately 6 to 7 mL/kg of body weight (Figure 36-3), yet historically, tidal volumes of 12 to 15 mL/kg were used in mechanically ventilated patients with acute respiratory failure, and peak alveolar pressures were allowed to increase above 40 cm H2
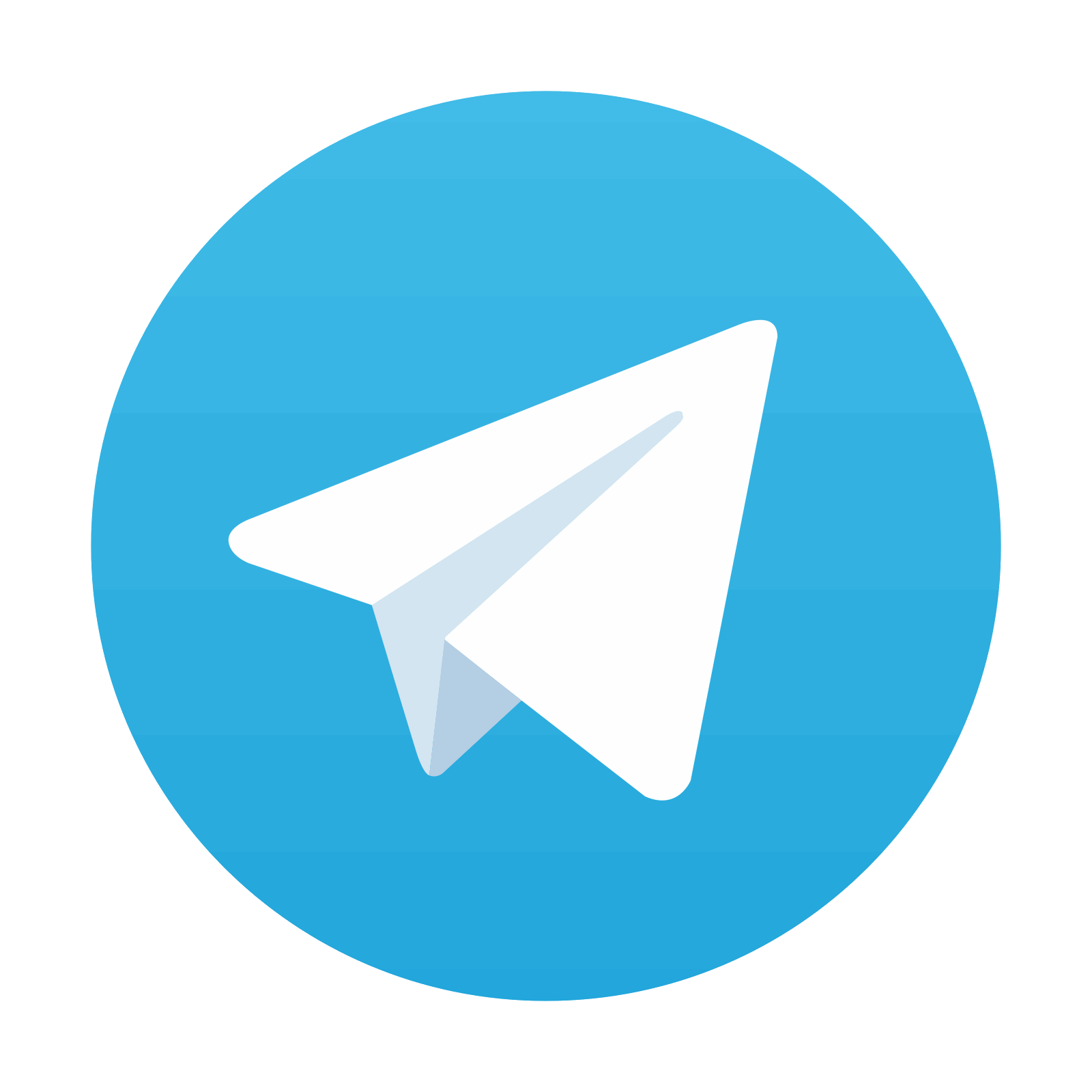
Stay updated, free articles. Join our Telegram channel

Full access? Get Clinical Tree
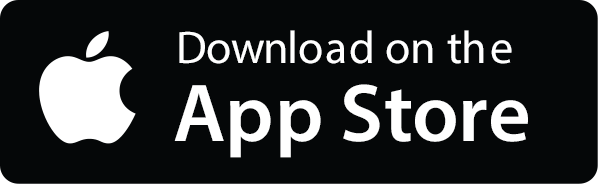
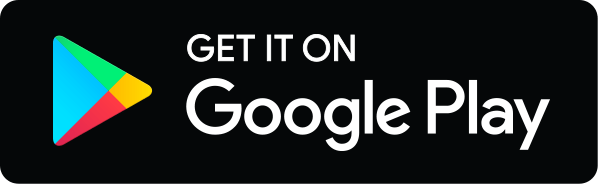