Chapter 33 Acute Renal Failure and Renal Support
Renal dysfunction is relatively common following cardiac surgery and is associated with a substantial increase in mortality rates. The incidence of postoperative renal dysfunction (PRD) varies according to the way in which it is defined and the population being studied. In unselected groups of cardiac surgery patients with PRD, mortality rates of 3.4% to 17% have been reported.1–4 The need for renal replacement therapy occurs in 1% to 4% of cases and is associated with mortality rates of 30% to 70%.1,2,4–7
In this chapter, the risk factors, diagnosis, and treatment of postoperative acute renal failure are discussed. Traditionally, renal replacement therapy for acute renal failure in cardiac surgery patients has involved peritoneal dialysis and intermittent hemodialysis. However, over the past decade, continuous and semicontinuous therapies that are better suited to the critically ill have become common. The indications, various types, and practicalities of these techniques are discussed. The physiology of normal and abnormal renal function is reviewed in Chapters 1 & 31.
ASSESSMENT OF RENAL FUNCTION
Tests of renal function evaluate either glomerular filtration rate (GFR) or tubular function. Severe renal hypoperfusion results in a reduction in the GFR and an increase in the plasma concentration of nitrogenous wastes (urea and creatinine). This is known as prerenal azotemia. When renal hypoperfusion is severe and sustained, acute tubular necrosis can occur, and it results in abnormal tubular function. The pathophysiologic mechanisms of prerenal azotemia and acute tubular necrosis are described in Chapter 1.
Glomerular Filtration
The two most widely used biochemical tests of GFR are plasma creatinine and blood urea nitrogen (BUN). The normal plasma creatinine concentration is 50 to 120 μmol/l (0.6 to 1.4 mg/dl) in males and 40 to 100 μmol/l (0.5 to 1.1 mg/dl) in females. The normal BUN concentration is 3.2 to 7.7 mmol/l (9 to 22 mg/dl). Of the two, creatinine is the more reflective of the GFR. Creatinine is released from muscle at a relatively constant rate, is freely filtered at the glomerulus and, unlike urea, is not reabsorbed in the tubules. Creatinine concentration is relatively constant despite changes in diet and metabolic rate. As people age, there is a gradual reduction in the GFR, which is matched by a decline in muscle mass. Thus, creatinine concentration remains relatively constant. The relationship between the creatinine concentration and the GFR is not linear (Fig. 33-1); with normal renal function, a small rise in creatinine concentration signals a large deterioration in the GFR; with severe renal dysfunction, large changes in creatinine reflect small changes in the GFR.
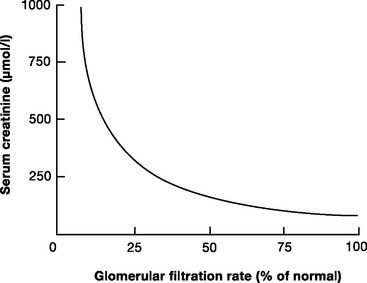
Figure 33.1 The relationship between plasma creatinine concentration and creatinine clearance. This graph assumes that plasma creatinine concentration is stable.
A more accurate estimate of the GFR, which is independent of age, is creatinine clearance. The normal range for creatinine clearance is 120 ± 25 ml/min for men and 95 ± 20 ml/min for women.8 Creatinine clearance (CCr) can be calculated on the basis of the plasma (PCr) and urinary (UCr) concentrations of creatinine and the urine volume (V):
This requires 24-hour urine collection. A simpler method is to estimate creatinine clearance on the basis of the following empiric formula:
This formula assumes that a steady state exists; it cannot be applied to estimate the GFR during the development of acute renal failure.
Measurement (or estimation) of creatinine clearance is particularly important in the elderly and in patients with abnormal body mass indexes.
As with creatinine, BUN levels are inversely related to the GFR. However, BUN levels are also influenced by the rate of urea production and the degree of tubular reabsorption. Increased urea production, and therefore increased BUN levels, occur with a high-protein diet, gastrointestinal bleeding, and increased protein catabolism (e.g., treatment with corticosteroids). Increased tubular reabsorption of urea, and therefore increased BUN levels, occur when tubular flow is low, as occurs in renal hypoperfusion. Thus, a greater increase in BUN levels relative to creatinine levels also occurs with hypovolemia and heart failure. One advantage of measuring BUN levels rather than creatinine levels is that they increase within a few hours of a reduction in the GFR, whereas creatinine takes at least 8 hours to become significantly elevated.
Tubular Function
Tubular dysfunction results in the inability to generate appropriately concentrated urine in response to a physiologic stimulus. With prerenal azotemia, tubular function is intact. In this situation, high levels of aldosterone and antidiuretic hormone lead to maximal tubular reabsorption of sodium and the excretion of low-volume, hypertonic urine. Urine osmolarity is typically greater than 500 mOsm/l and urinary sodium concentration less than 20 mmol/l. An exception to these findings is seen in patients treated with diuretics and in the very elderly, who have a higher sodium concentration and a lower osmolarity. With acute tubular necrosis, there is loss of the normal hypertonicity of the medulla (see Chapter 1) and the inability to conserve sodium and excrete concentrated urine. In this circumstance, urine osmolarity is typically less than 400 mOsm/l, and urinary sodium concentration is greater than 20 mmol/l.
Urine Output
Oliguria (urine output <0.5 ml/kg/hr) is an important marker of renal hypoperfusion and evolving acute tubular necrosis. However, urine output must be interpreted with caution. With a normal diet, about 600 mOsm/day of solute waste products generated by metabolism must be excreted in the urine. With normal renal tubular function and maximal antidiuretic hormone stimulation, this requires a minimum of 500 ml/day of urine. In postoperative patients who have low dietary intake and high plasma levels of catecholamines, aldosterone, and antidiuretic hormone (see Chapter 32), oliguria may be a normal finding and not indicative of a reduced GFR or tubular dysfunction. Oliguria in association with normal plasma creatinine concentration and normal intravascular volume does not require treatment. However, oliguria in association with prerenal azotemia or acute tubular necrosis mandates a search for the cause of renal hypoperfusion, in particular, a careful assessment of intravascular volume status and cardiac function. Conversely, high urine output can occur despite intravascular volume depletion. Polyuria is common in the first few hours following cardiac surgery as a consequence of hypothermic cardiopulmonary bypass (CPB) and the administration of diuretics—particularly mannitol, which may be used in the CPB prime solution.
Oliguria is usually present with prerenal azotemia in the early period of acute tubular necrosis. If tubular necrosis is severe, anuria develops because of a low or absent GFR and tubular obstruction (see Chapter 1). If the GFR is then partially or fully restored, polyuria often develops due to the inability to concentrate urine.
Renal Imaging
Ultrasound scanning of the kidneys and renal tract is the most useful imaging technique in patients with acute renal dysfunction, and it can be performed at the bedside. Ultrasonography can identify obstruction in the upper or lower urinary tract and can confirm the presence and size of each kidney. Small (or large polycystic) kidneys indicate chronic renal disease. Doppler flow imaging may suggest the presence of atherosclerotic renal artery stenosis or, occasionally, renal vein thrombosis.
Intravenous pyelography and contrast-enhanced computed tomography should be avoided if possible because of the risk for contrast nephropathy, unless it is likely to change the management of the patient (e.g., renal artery stenting following aortic dissection).
ACUTE RENAL FAILURE
Definition
Various definitions of renal dysfunction are used; they are based on the plasma creatinine concentration, the percentage of rise in creatinine concentration, or the need for renal replacement therapy. Recently, the RIFLE (risk, injury, failure, loss, and end-stage kidney disease) classification has been proposed by the Acute Dialysis Quality Initiative Workgroup as a consensus definition of acute renal failure in critically ill patients.9 Renal dysfunction is divided into three categories (risk, injury, failure) on the basis of plasma creatinine concentration and urine output. In addition, there are two levels of outcome (loss, end-stage kidney disease), determined by the persistence of renal failure at 4 weeks or 3 months (Table 33-1). The RIFLE classification has recently been evaluated in cardiac surgery patients and has been shown to be an independent predictor of mortality: patients categorized as having risk, injury, or failure postoperatively had 90-day mortality rates of 8%, 22%, and 33%, respectively.10
Table 33-1 RIFLE Classification of Renal Dysfunction and Failure
Classification | GFR Criteria | Urine Output Criteria |
---|---|---|
Risk | Increase in creatinine by 50% | Urine volume <0.5 ml/kg/hr for 6 hr |
Injury | Increase in creatinine by 100% | Urine volume <0.5 ml/kg/hr for 12 hr |
Failure | Increase in creatinine by 200% to more than 4 mg/dl (354 μmol/l), with a rise ≥0.5 mg/dl (44μmol/l | Urine volume <0.3 ml/kg/hr for 24 hr or anuria for 12 hr |
Loss | Persistent acute renal failure or complete loss of renal function for >4 weeks | |
End-stage kidney disease | End-stage kidney disease (>3 months) |
GFR, glomerular filtration rate
From Bellomo R, Ronco C, Kellum JA, et al: Acute renal failure—definition, outcome measures, animal models, fluid therapy and i nformation technology needs: the Second International Consensus Conference of the Acute Dialysis Quality Initiative (ADQI) Group. Crit Care 8:R204-R212, 2004.
Risk Factors for Acute Renal Failure Following Cardiac Surgery
The causes of renal failure following cardiac surgery are multifactorial. Renal hypoperfusion may occur because of periods of hypovolemia, hypotension, or low cardiac output. Nonpulsatile CPB leads to reduced renal blood flow.11,12 Renal function may be adversely affected by the systemic inflammatory response to surgery and CPB and by free plasma hemoglobin generated by bypass-induced trauma to red blood cells. Atheroembolism may occur due to aortic manipulations such as cross-clamping, cannulation, and the use of an intraaortic balloon pump. Nephrotoxic drugs—in particular radiocontrast agents used for coronary angiography—may be administered during the perioperative period.
The risk factors for renal failure requiring renal replacement therapy following cardiac surgery are listed in Table 33-2. Of these, preexisting renal dysfunction is by far the most important; it is a major predictor of postoperative mortality.13
Table 33-2 Factors That are Independently Associated with the Need for Postoperative Renal Replacement Therapy Following Cardiac Surgery
Requirement for blood transfusion |
Emergency operation |
Preoperative renal dysfunction (creatinine >124 μmol/l or > 1.4 mg/dl) |
Requirement for re-sternotomy |
Mitral valve surgery |
Low ejection fraction (< 40%) |
Use of an intraaortic balloon pump |
Prolonged cardiopulmonary bypass time |
From Bove T, Calabro MG, Landoni G, et al: The incidence and risk of acute renal failure after cardiac surgery. J Cardiothorac Vasc Anesth 18:442-445, 2004.
Causes of chronic renal dysfunction include hypertension, diabetes, connective tissue disease, chronic use of nonsteroidal antiinflammatory drugs, glomerulonephritis, and polycystic kidney disease. Chronic renal dysfunction is very common in patients presenting for cardiac surgery. In one large series, 51% of patients had mild renal dysfunction (GFR 60 to 90 ml/min); 24% had moderate renal dysfunction (GFR 30 to 59 ml/min); 2% had severe renal dysfunction (GFR <30 ml/min); and 1.5% were dialysis dependent.13
Nephrotoxic Drugs
Drugs may cause renal injury by impairing renal blood flow or by causing tubular damage. Drugs such as nonsteroidal antiinflammatory drugs (NSAIDS) adversely affect vascular tone in the glomerular arterioles and can therefore reduce renal blood flow and the GFR. These drugs should be avoided when renal blood flow is reduced (e.g., in hypovolemia and low cardiac output) or fixed (e.g., in bilateral renal artery stenosis). Similarly, the use of vasopressors in the presence of hypovolemia can lead to reduced renal blood flow (see subsequent material). By virtue of their ability to cause intravascular volume depletion, diuretics are also potentially nephrotoxic.
Aminoglycosides, amphotericin and, to a lesser extent, vancomycin can cause tubular injury. In the case of amphotericin, the lipophilic formulation is associated with reduced tubular injury and should be used when there is renal dysfunction.14
Cyclosporin-induced renal dysfunction is characterized by an obliterative vasculopathy of the afferent arteriole and tubulointerstitial fibrosis.15 These changes (which take weeks or months to develop) are believed to be caused by an alteration in the release of vasoactive substances, such as angiotensin II, endothelin, prostaglandins, and nitric oxide, as well as by the stimulation of certain proliferative cell genes.15 Contrast nephropathy is discussed subsequently.
Physiologic Consequences of Renal Failure
Acidosis and Hyperkalemia
In severe renal dysfunction, an elevated anion-gap metabolic acidosis develops due to the inability of the kidneys to excrete the acid load generated by metabolism (see Chapter 31). In chronic renal failure, metabolic acidosis becomes apparent when the GFR falls to about 25% of normal, but in acute tubular necrosis, acidosis is an early finding.
Potassium undergoes glomerular filtration and tubular secretion (see Chapter 32). Hyperkalemia can develop when renal blood flow is low—independent of acute tubular necrosis—as the result of reduced blood flow in the peritubular capillaries. Thus, hyperkalemia is closely linked to oliguria and is uncommon in polyuric renal failure.
Water and Sodium Excess
Oliguric renal failure is associated with water and sodium excess, which can cause pulmonary congestion and systemic edema and can contribute to hypertension. Water is usually ingested in excess of sodium, so hyponatremia is common (see Chapter 32).
Other Electrolytes
The excretion of phosphate and magnesium is reduced in acute renal failure, and hyperphosphatemia and hypermagnesemia develop relatively quickly. Phosphate forms insoluble salts with calcium and leads to ionized hypocalcemia.
Uremia
Uremia is an imprecise term that comprises a range of conditions associated with the accumulation of the products of protein metabolism (uremic toxins). Uremic symptoms correlate with BUN levels but are not directly caused by high levels of urea. Conditions associated with uremia include encephalopathy, neuropathy, and serositis.
Signs of uremic encephalopathy are uncommon when the BUN level is less than 35 mmol/l (100 mg/dl). Initial symptoms include nausea, lethargy, anorexia, and reduced cognitive function. Subsequently, delirium, stupor, flapping tremor (asterixis), seizures, and coma can develop. Uremic serositis is a syndrome involving one or more of the following symptoms: pericardial effusion, pleural effusion, and ascites.
Chronic Renal Failure
Patients with chronic renal failure may have a number of abnormalities in addition to those described for acute renal failure. They include hypertension, anemia (due to reduced renal synthesis of erythropoietin), abnormal platelet function, and reduced immune competence. Chronic hyperphosphatemia can lead to the deposition of calcium phosphate salts in soft tissues such as skeletal muscle and blood vessels. Hypocalcemia stimulates the release of parathyroid hormone which, over time, can cause bone demineralization (renal osteodystrophy).
NONDIALYTIC MANAGEMENT OF ACUTE RENAL FAILURE
Monitoring Renal Function
In all patients with acute renal dysfunction, a urinary catheter should be inserted. Fluid input and output should be recorded hourly. Acid-base, sodium, potassium, creatinine, BUN, and hemoglobin levels should be measured at least daily. Potassium should be measured much more frequently (e.g., every 1 to 4 hours) in acutely oliguric patients. Measurements of urinary sodium concentration and osmolarity are also useful. Careful monitoring of cardiorespiratory function is indicated. Intraabdominal pressure monitoring may also be indicated.
Preventing Further Renal Injury
Renal Tract Obstruction
A rare but important cause of acute renal failure is an obstructed urinary tract. Abrupt cessation of, or large variations in, urine output may indicate a blocked or partially obstructed urinary catheter. If this is suspected, the catheter should be flushed with 50 ml of sterile water. If the problem persists, the catheter should be replaced and, if necessary, an ultrasound scan of the bladder performed to assess residual urine volume. If renal dysfunction is out of proportion to the apparent degree of hypoperfusion, an ultrasound scan of the kidneys and renal tract should be obtained to exclude obstructive nephropathy.
Occasionally, raised intraabdominal pressure due to abdominal pathology contributes to renal (and respiratory and splanchnic) dysfunction. If this is suspected, intravesicular (bladder) pressure, which is a surrogate for intraabdominal pressure, should be measured. An intravesicular pressure greater than 24 cm H2O is associated with the development of postoperative renal failure.16 If intraabdominal hypertension is severe (intravesicular pressure >30 to 40 cm H2O) and there are signs of renal, respiratory, or splanchnic compromise, a laparotomy to decompress the abdomen should be considered.17,18
Optimizing Cardiovascular Performance
Maintaining an adequate circulating volume and hemodynamic state is of paramount importance in preventing further renal injury. Patients with evolving renal failure should undergo careful examinations of the cardiovascular and respiratory systems. If there is hypotension or evidence of low cardiac output, central venous and intraarterial pressure monitoring is indicated. Measurement of superior vena cava oxygen saturation (SSVCo2; see Chapter 20) is also valuable. Hypovolemia should be treated by a balanced salt solution. Normal (0.9%) saline can exacerbate acidosis (see Chapter 31) and Plasma-Lyte (which contains potassium; see Table 32-3) can exacerbate hyperkalemia. If, despite adequate volume resuscitation, the hemodynamic state remains inadequate, pulmonary artery catheterization and echocardiography should be considered to guide further treatment.
Table 33-3 Criteria for the Initiation of Renal Replacement Therapy
Oliguria (urine volume <500 ml/day) |
Anuria (urine volume <50 ml/12 hr) |
BUN >30 mmol/l (>85 mg/dl) |
Creatinine >400 mol/l (>4.5 mg/dl) |
Potassium >6 mmol/l |
Pulmonary edema not responsive to diuretics |
Severe metabolic acidemia (pH <7.1, base deficit >10 mmol/l) |
Uremic encephalopathy |
Uremic pericarditis |
Uremic neuropathy |
From Bellomo R, Ronco C: Indications and criteria for initiating renal replacement therapy in the intensive care unit. Kidney Int 66(suppl):S106-S109, 1998.
In normotensive patients, the lower limit of autoregulation of renal blood flow is 70 to 75 mmHg.19 This lower limit is likely to be higher in patients with chronic hypertension. Furthermore, in critically unwell patients, autoregulation may be lost entirely, in which case renal blood flow becomes pressure dependent.19
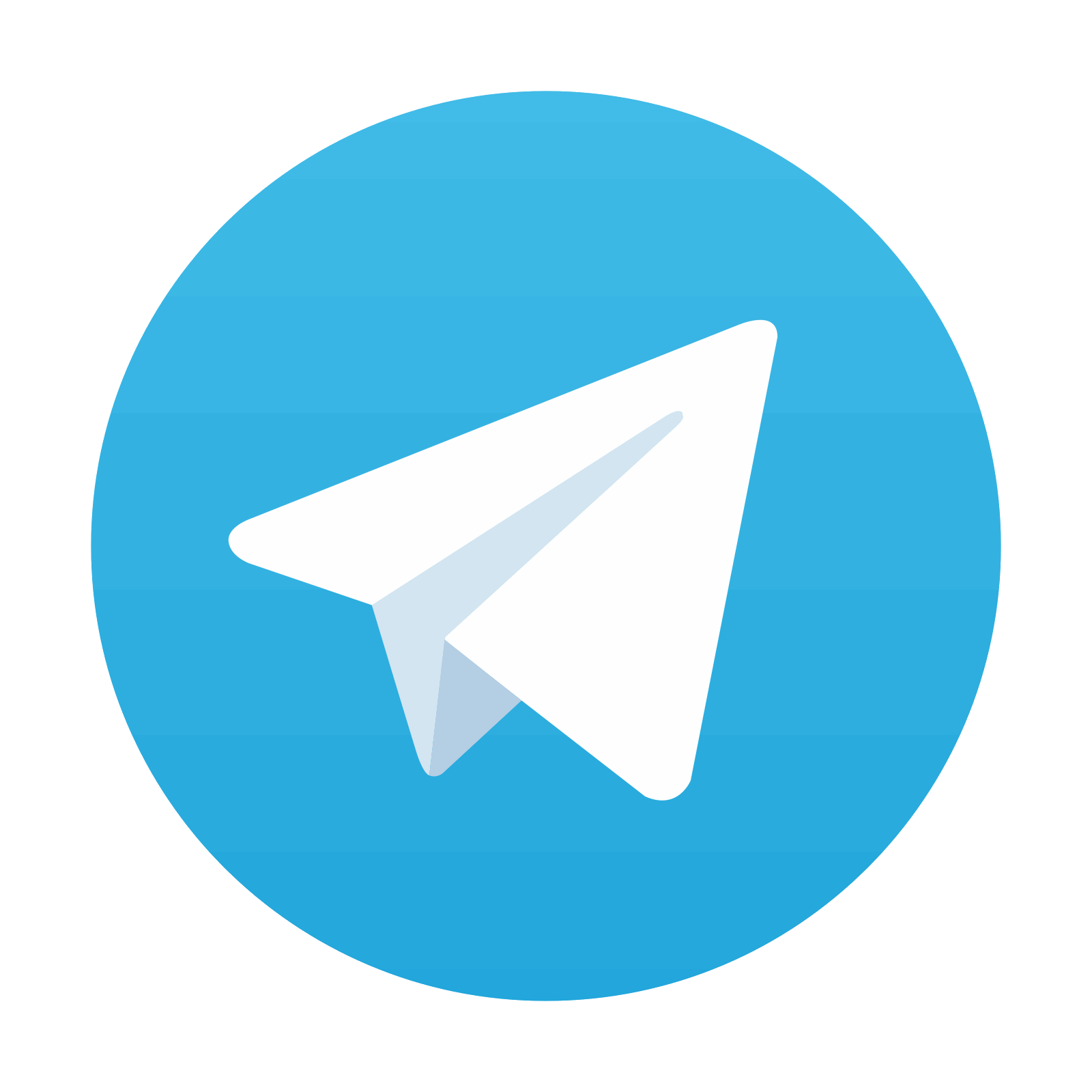
Stay updated, free articles. Join our Telegram channel

Full access? Get Clinical Tree
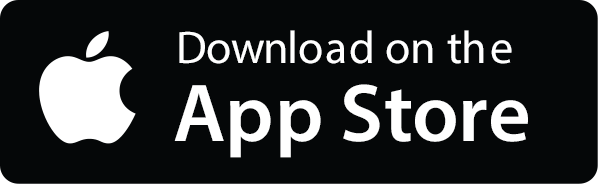
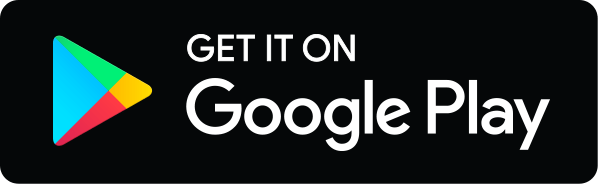