Fig. 7.1
The use of the Stevenson Hemodynamic Profile as a guide for acute heart failure management. Congestion is determined by the presence of orthopnea, jugular venous distension, rales, hepatojugular reflex, ascites, peripheral edema, a leftward radiation of the pulmonic heart sound, and/or a square-wave blood pressure response to the Valsalva maneuver. Inadequate perfusion is determined by the presence of a narrow pulse pressure, pulsus alternans, symptomatic hypotension, cool extremities, and/or impaired mentation (modified from Nohria et al. J Am Coll Cardiol. 2003;41(10):1797–804)
Biomarkers and Heart Failure
Acute decompensated heart failure contributes to a significant proportion of hospital admissions and is associated with high morbidity and mortality rates [2, 7–10]. A variety of biomarkers related to heart failure have emerged to provide not only more precise diagnosis but have also been correlated to response to therapy and, more importantly, to prognosis. Circulating natriuretic peptides and cardiac troponins have emerged as the leading biomarkers in the field of heart failure. Measuring their levels is now part of the standard of care in the assessment and management of a patient who presents to a medical facility with signs and symptoms of ADHF [12–15].
Biomarkers and Heart Failure: Natriuretic Peptides
Cardiac myocytes release natriuretic peptides in response to wall stress, typically in the setting of chamber dilation resulting from volume overload or myocardial injury [16, 17]. These hormones activate cyclic guanosine monophosphate-dependent signaling cascades. Through this pathway, these “counterregulatory hormones” exert a number of actions that counteract the hemodynamic aberrations commonly observed in heart failure.
Three predominant natriuretic peptides circulate in the bloodstream and have been well characterized (◘ Table 7.1). Natriuretic peptides, specifically the B-type natriuretic peptide (BNP) and amino-terminal counterpart (NT-proBNP), are useful for establishing the diagnosis of acute heart failure and distinguishing heart failure from other noncardiac causes of dyspnea [18]. BNP and NT-proBNP levels are well correlated and either assay can be utilized in this setting. Generally, as the BNP threshold level is increased, the sensitivity for heart failure declines, but specificity rises (◘ Fig. 7.2) [18]. As demonstrated in the ProBNP Investigation of Dyspnea in the Emergency Department (PRIDE) trial, natriuretic peptides are most helpful when used in conjunction with and to support medical decision-making [19], particularly when the cause of dyspnea is not evident. However, it is important to note that elevated plasma levels may result from a variety of conditions, including acute coronary syndromes, valvular heart disease, myocarditis, pericarditis, atrial fibrillation, and/or cardiac surgery [1].

Table 7.1
Natriuretic peptides in heart failure
Natriuretic peptide | Stimulus | Actions |
---|---|---|
A-type (ANP) | Atrial distension | Vasodilation, natriuresis, attenuation of RAAS, promote renal blood flow |
B-type (BNP) | Ventricle distension | Vasodilation, natriuresis, attenuation of RAAS, promote renal blood flow |
C-type (CNP) | Vascular endothelium | Vasodilation, natriuresis, attenuation of RAAS, antiproliferation of vascular smooth muscle |

Fig. 7.2
The role of B-type natriuretic peptide (BNP) in assessing the etiology of dyspnea. (a) Receiver operating characteristic curve for various cutoff levels of BNP in differentiating between dyspnea due to congestive heart failure and dyspnea due to other causes. (b) Table outlining the sensitivity, specificity, predictive values, and accuracy of BNP levels. Note that the figure was adapted from reference #18
Natriuretic peptides also have important prognostic implications, as higher BNP levels indicate an increased risk of mortality [20–25]. Furthermore, changes in BNP over time are associated with corresponding changes in the risk of death [21]—and while natriuretic peptide levels generally improve with the treatment of heart failure [21, 26–28], it is unclear whether “guided therapy” leads to improved outcomes. Trials examining such a strategy have generally been limited by sample size and power; however, in two meta-analyses, therapy guided by natriuretic peptide levels led to reductions in all-cause mortality [29, 30]. Prospective, randomized controlled studies are ongoing to determine whether outcomes are improved by titrating heart failure medications to achieve a target NT-proBNP level [31]. The American College of Cardiology Foundation (ACCF) and the American Heart Association (AHA) 2013 guidelines on heart failure management therefore provide a class IA recommendation for the inclusion of natriuretic peptide levels to establish the diagnosis of heart failure as the cause for dyspnea in the acute care setting, but a class IIB recommendation for the use of these hormones to guide therapy after the diagnosis of heart failure has been made [32]. It is important to note that natriuretic peptides are dependent on renal clearance; thus, BNP and/or NT-proBNP levels may be elevated in the setting of acute kidney injury. In addition, an inverse correlation exists between natriuretic peptide levels and body mass index. Therefore, obese individuals may have falsely low levels of BNP and/or NT-proBNP .
Biomarkers and Heart Failure: Cardiac Troponins
Cardiac troponin levels have previously been shown to correlate with impaired hemodynamics and BNP levels in patients with HF [33]. This finding implies that in the setting of HF, there is ongoing injury and destruction of cardiomyocytes that contributes to the decline in clinical status [34]. Similar to natriuretic peptide levels, elevated troponin levels are associated with worse outcomes [33–40]. The ACCF/AHA 2013 guidelines on heart failure management therefore provide a class IA recommendation for including cardiac troponins for risk stratification of patients hospitalized with acute heart failure [32].
Myocardial Infarction and Acute Decompensated Heart Failure
Myocardial infarction is common and is associated with tremendous morbidity and mortality. Each year, more than 700,000 Americans have a myocardial infarction, of which 500,000 of these patients experience their first heart attack [2]. About 25–40 % of these myocardial infarctions are ST-segment elevation myocardial infarctions (STEMIs ) [2]. These STEMI injuries result in complete occlusion of the coronary vessel and cause a transmural infarct in the absence of an intervention. While healthcare education and primary percutaneous interventional revascularization strategies have decreased the number of transmural infarctions, acute pump failure due to proximal left anterior descending (LAD) artery occlusion requires urgent attention. These patients typically present with tachycardia, hypotension, pulmonary edema, and end-organ dysfunction (elevated hepatic transaminases, renal dysfunction, etc.). This low-output state or cardiogenic shock (cardiac index less than 2 l/min/m2) still requires percutaneous revascularization and, in the absence of aortic insufficiency or peripheral vascular disease, support with a short-term mechanical circulatory support device [e.g., Impella (2.5 or 5.0), intra-aortic balloon pump (IABP), or TandemHeart]. In addition to the mechanical support provided by a temporary ventricular assist device, supplemental oxygen and low-molecular-weight heparin can be used with or without intravenous nitroglycerin. The goal is to promote cardiac perfusion and decrease afterload to ultimately promote cardiac output. Patients with myocardial infarction and Killip class IV (cardiogenic shock ) (◘ Table 7.2) have a mortality rate of about 25 % and require intervention, ICU monitoring, and nursing support [25–41].
Table 7.2
Killip classification in patients following ischemic cardiac insult
Killip Classification | |
---|---|
Killip class I | Signs of heart failure |
Killip class II | Rales and diffuse wheezing over the lung fields, and/or an S3 |
Killip class III | Acute pulmonary edema |
Killip class IV | Cardiogenic shock |
Structural Complications and Acute Heart Failure
Following the patient’s immediate stabilization, it is important to monitor for structural complications . The profile of patients at higher risk for structural complications following myocardial infarction (MI) includes female gender, first ischemic event, low body mass index, and advanced age [2]. Typically, ventricular (septal or free wall) rupture occurs about 2–8 days following the transmural infarct and is associated temporally with extracellular remodeling of the infarct [46–49]. The mortality for patients with these structural perforations is extremely high and requires immediate surgical intervention. Typically, an ischemic myocardial rupture following an acute myocardial infarction involves the left ventricular or right ventricular free walls, ventricular septum, or left ventricular papillary muscle (in decreasing order of frequency) [46–49]. Rupture may cause pericardial tamponade, formation of a pseudoaneurysm, acute mitral regurgitation, or shunt (ventricular septal defect resulting in a left-to-right shunt) (◘ Fig. 7.3). The diagnosis usually is established using transthoracic echocardiography. Physical exam findings include a holosystolic murmur with or without a thrill (typically associated with a ventricular septal rupture) appreciated at the left sternal border, and hypotension.


Fig. 7.3
Ventricular septal rupture following a myocardial infarction results in acute heart failure. Schematic highlighting ventricular septal rupture following a myocardial infarction and subsequent left-to-right shunt
Inferior wall myocardial infarctions involving the posterior descending coronary artery are also associated with structural complications. These complications are due to the single-vessel (typically the right coronary artery) blood supply to the posterormedial papillary muscle for the mitral valve. Rupture of the posterormedial papillary muscle results in acute and severe mitral regurgitation. The acute rupture of the posterormedial papillary muscle results in hypotension (decreased cardiac output), pulmonary congestion (resulting in hypoxia), a holosystolic murmur appreciated at the apex and radiating to the axilla, and tachycardia. This complication requires immediate intervention with parenteral agents such as sodium nitroprusside (SNP) to decrease afterload, decrease the regurgitant fraction, and increase forward flow (increase cardiac output) with diuretic therapy, and surgical consultation for mitral valve replacement. Acute rupture of the posterormedial papillary muscle with severe mitral regurgitation, pulmonary edema, and hypotension is a surgical emergency. While SNP and IABP support may improve cardiac output, surgical intervention is required for improved morbidity and survival following ischemic rupture of the posterormedial papillary muscle and acute heart failure.
Myocarditis
Myocarditis is another cardiovascular disease state resulting in ADHF and characterized by inflammation and damage to the heart muscle. Myocarditis is believed to be a relatively common cause of sudden cardiac death in the young; about 10 % of sudden cardiac deaths are due to myocarditis [50–52]. The causes of myocarditis include autoimmune diseases, viral infections (Coxsackie B, hepatitis B, hepatitis C, human immunodeficiency virus (HIV), or human parvovirus B19), nonviral infections (Borrelia burgdorferi, Aspergillus, Haemophilus influenzae, gonococcus, Corynebacterium diphtheriae, etc.), toxins (alcohol, cocaine, etc.), and adverse reactions to medications (anthracyclines, antipsychotics, digoxin, dobutamine, cephalosporins, etc.) [50, 52, 53]. Typically, patients complain of flu-like symptoms (fever, chills, myalgias, night sweats, malaise, etc.), chest pain, and symptoms consistent with congestive heart failure (i.e., shortness of breath, dyspnea on exertion, orthopnea, paroxysmal nocturnal dyspnea, etc.). Patients will often have an elevated erythrocyte sedimentation rate, C-reactive protein, complete blood count, serum cardiac troponin levels, and electrocardiogram (ECG) findings. These ECG findings may consist of ST-segment elevation, T-wave inversion, ST-segment depressions, or Q waves that extend beyond a single coronary artery distribution. An endocardial biopsy (revealing an eosinophilic infiltrate, myocardial edema, inflammation, cardiomyocyte necrosis, etc.) remains the gold standard for the diagnosis (◘ Fig. 7.4) , but the increasing use of cardiac magnetic resonance imaging has also been useful (◘ Fig. 7.5) [52]. Other specific causes of myocarditis include sarcoidosis, eosinophilic myocarditis, or giant cell myocarditis [50, 52, 54–56]. Giant cell myocarditis is relatively rare but typically is rapidly progressive and lethal. Diagnosis of giant cell myocarditis is based on the presence of multinucleated giant cells and a T-lymphocytic cellular infiltration on biopsy (◘ Fig. 7.6). As outlined in the Multicenter Giant Cell Myocarditis Study Group , acute heart failure is the typical presenting symptom in more than 75 % of the patients [54].




Fig. 7.4
Endomyocardial biopsy is the gold standard for the diagnosis of myocarditis . (a) An endocardial biopsy in a patient with lymphocytic myocarditis shows myocardial edema, lymphocytic infiltration (arrowheads), and cardiomyocyte necrosis. (b) An endocardial biopsy from a patient with cardiac sarcoid. Note the noncaseating epithelioid-cell granulomas (arrowheads) that may convert into hyaline connective tissue

Fig. 7.5
Cardiac magnetic resonance (CMR) imaging and gadolinium enhancement is useful for the diagnosis of myocarditis. CMR of a patient with acute viral myocarditis demonstrating epicardial hyperenhancement (white arrows) in the anterior and lateral walls. Panel a is the four-chamber view, Panel b is the two-chamber view, Panel c is the three-chamber view, and Panel d is the short-axis view

Fig. 7.6
Endomyocardial biopsy is essential for the diagnosis of giant cell myocarditis. Endomyocardial biopsy specimen from a patient with giant cell myocarditis. Note the extensive infiltrate of lymphocytes, macrophages, and giant cells (solid arrowheads) with cardiomyocyte necrosis (open arrowheads)
Medical Management of Acute Heart Failure
The ACCF/AHA 2013 guidelines on heart failure management recommend that goal-directed medical therapy be continued for all patients requiring hospitalization for acute heart failure in the absence of hemodynamic instability [1]. However, all maintenance heart failure medications should be reviewed carefully on hospital admission to determine whether it is appropriate to continue, adjust, or temporarily withhold heart failure medications based on an individual’s hemodynamic profile.
The ACCF/AHA 2013 guidelines on heart failure recommend that beta blockers should generally be continued during the hospitalization for acute heart failure if it is reasonable and safe for the patient [1]. This recommendation is based on data derived from large studies evaluating patients hospitalized with acute heart failure, which demonstrated improved survival among individuals whose beta blockers were continued during hospitalizations for acute heart failure [57, 58]. For example, the ESCAPE trial showed that continuation of beta blockers throughout hospitalization was associated with a reduction in the rate of rehospitalization or death within a 6-month period following discharge [57]. Similarly, the Carvedilol Or Metoprolol European Trial (COMET ) showed that 1- and 2-year mortality rates were higher in patients whose beta blockers were discontinued [58]. It is important to emphasize that the higher mortality rate among individuals for whom beta blockers were stopped is related, in part, to the severity of their underlying disease, and there are a number of scenarios in which beta blockers should be discontinued [58]. For example, beta blockers should be withheld on patients who present with a “wet-cold” profile (◘ Fig. 7.1; Stevenson Hemodynamic Profile C), with evidence of congestion, and with a low-output state (inadequate perfusion) until they are stabilized [1].
Similarly, angiotensin-converting enzyme (ACE) inhibitors and aldosterone receptor antagonists (ARBs) should be continued during hospitalizations for acute heart failure , as long as it is reasonable to do so [1]. ACE inhibitors may be advantageous in this setting, by reducing afterload and systemic vascular resistance and by promoting natriuresis [58]. Patients with significant rises in creatinine (representing a decrease in glomerular filtration rate, GFR) should have temporary reductions in the dosage of ACE inhibitors/ARBs or have the drugs held altogether until the renal function normalizes.
Diuretics are a class I indication for patients admitted with heart failure who have evidence of volume overload (e.g., Stevenson Hemodynamic Profile B or C) [1]. Generally, the dose administered should be equal to or greater than the patient’s chronic daily oral dose, and the diuretic should be administered as an intravenous formulation. In the Diuretic Optimization Strategies Evaluation (DOSE) trial , there was no difference in symptoms or change in renal function when diuretics were administered as either a bolus or continuous infusion [60]. Thus, the method of intravenous administration is generally based on clinician experience/preference. For patients who have congestion refractory to loop diuretics, clinicians may elect to either increase the dose of intravenous loop diuretics or add a thiazide diuretic to promote natriuresis [1]. When attempts for diuresis are unsuccessful, ultrafiltration may be considered as an adjunctive therapy. However, outcomes on ultrafiltration in this context are mixed. In one study of 200 patients admitted with acute heart failure and congestion, ultrafiltration led to greater weight and fluid loss than intravenous diuretics, as well as a reduction in 90-day rehospitalizations [61]. However, in a similarly sized cohort of patients with acute decompensated heart failure and congestion, pharmacologic therapy (intravenous diuretics) more effectively reduced creatinine levels than ultrafiltration [62]. Furthermore, ultrafiltration use was associated with a higher rate of adverse events, including worsening renal failure, bleeding complications, and catheter-related complications [62].
Attempts at diuresis are frequently impaired by hyponatremia. In states of hypervolemic hyponatremia, arginine vasopressin antagonists may be considered to allow for continued diuresis. Vasopressin antagonists have been shown to improve serum sodium levels in this patient population [63]; however, there is no survival benefit associated with their usage [64].
Regarding the management of acute myocarditis, therapy usually consists of providing supportive care, which includes conventional heart failure medications such as vasodilators, ACE inhibitors, diuretics, parenteral inotropic medications (i.e., milrinone or dobutamine), and/or insertion of a short-term mechanical circulatory support device for decompensated acute heart failure. The use of inotropic agents and/or mechanical support requires intensive care unit monitoring due to the hemodynamic instability of the patient, the need for one-on-one nursing care, potential complications related to the mechanical device, and the proarrhythmic effects of inotropic agents. The use of immunosuppressive agents (i.e., prednisone, cyclosporine, or azathioprine) has been shown to have no survival benefits in patients randomized to immunosuppression vs. conventional supportive therapies (Myocarditis Treatment Trial) [65–67]. Furthermore, the Intervention in Myocarditis and Acute Cardiomyopathy study (a double-blind randomized study of 62 patients with myocarditis) did not show a survival benefit or improvement in cardiac function with the use of intravenous immune globulin [67].
Advanced Therapies for Acute Decompensated Heart Failure
Several therapeutic strategies exist for management of patients with advanced heart failure. Inotropes (typically either milrinone or dobutamine) are employed early in the treatment course for patients with evidence of congestion and inadequate end-organ perfusion [7, 68, 69]. However, patients with decompensated heart failure will often require a more aggressive treatment strategy, and, in such cases, mechanical circulatory support is employed either in the form of short-term or durable (permanent) left ventricular assist devices (LVADs ). Orthotopic heart transplantation (OHT ) may also be considered for patients with refractory disease .
Short-Term Mechanical Circulatory Support for Decompensated Heart Failure
Several types of devices are available to provide additional circulatory support for patients with heart failure refractory to medical management (◘ Table 7.3). Decisions regarding which device(s) to use depend on clinician experience/preference and the level of support needed [70–74]. Generally, these devices can be incorporated as a “bridging strategy” to support decompensated patients, while the failing left ventricle recovers, or while patients are awaiting definitive treatment in the form of either LVAD or OHT [75, 76].
Table 7.3
Types of short-term mechanical circulatory support devices for optimization of cardiac output in patients with refractory heart failure
Device | Level of support | Duration of support | Adverse events |
---|---|---|---|
IABP | 7–14 days | Thrombocytopenia: 50 % [74] | |
Fever: 36 % [74] | |||
Sepsis: 15.7 % [81] | |||
Limb ischemia: 4.3 % [81] | |||
Thromboembolism: 1 % [74] | |||
Impella 2.5 | 1.0–2.5 L/min [71] | 6 h–7 days [75] | Aortic insufficiency |
Tamponade | |||
Thromboembolism | |||
Impella 5.0 | 5.0 L/min | 6 h–7 days [75] | |
TandemHeart | 3.5–4.0 L/min [75] | 6 h–14 days [75] | Tamponade |
Bleeding | |||
Limb ischemia | |||
Residual ASD |
While these devices have the advantage of optimizing cardiac output, they are not without risks. Major risks linked to these devices include vascular complications associated with device insertion, thrombocytopenia, limb ischemia, infection, and thromboembolic phenomena. Generally, IABPs are associated with a high rate of thrombocytopenia and fever, whereas the TandemHeart is associated with a higher risk of bleeding [77].
Despite the widespread use of these devices (more than 70,000 IABPs are inserted annually in the United States [78]), outcomes are mixed. IABP does not improve outcomes when used as a prophylactic strategy prior to revascularization in high-risk patients suffering from acute myocardial infarction (AMI) [79], or in AMI patients suffering from cardiogenic shock [80]. The Intra-aortic Balloon Pump in Cardiogenic Shock II (IABP-SHOCK II) investigators reported a 30-day mortality of nearly 40 % among AMI patients with cardiogenic shock [80]. Further, when compared with IABP, the use of the Impella 2.5 led to similar rates of major adverse cardiac events at 30 days (35.1 % for Impella 2.5 vs. 40.1 % for IABP, P = 0.227) [81].
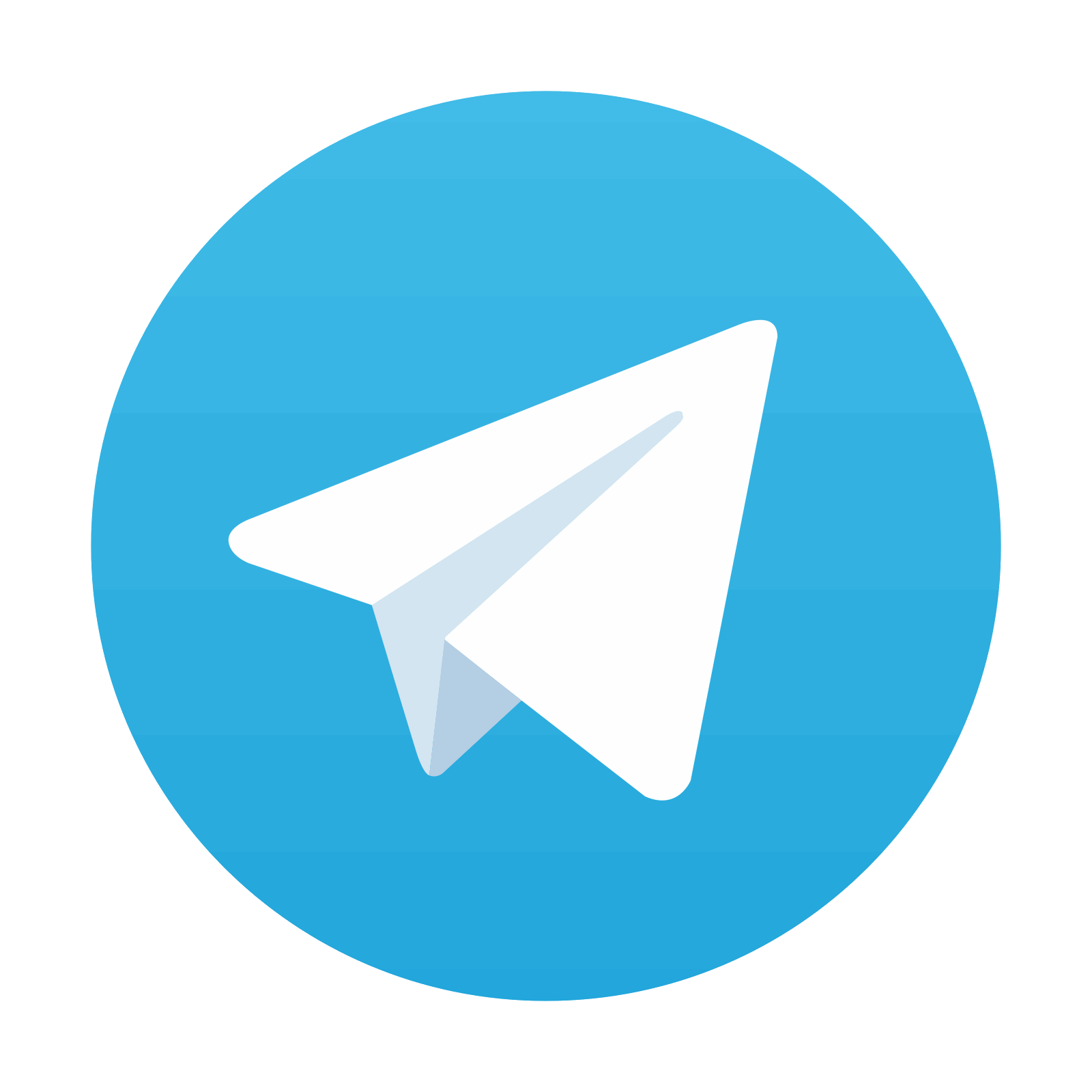
Stay updated, free articles. Join our Telegram channel

Full access? Get Clinical Tree
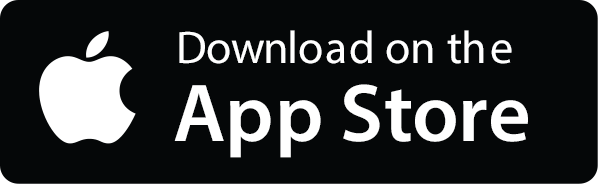
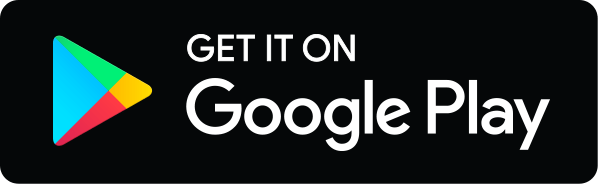