We tested the accuracy and reproducibility of knowledge-based reconstruction (KBR) for measuring right ventricular (RV) volume and function. KBR enables rapid assessment of the right ventricle from sparse user input by referencing a database. KBR generates a 3-dimensional surface to fit points that the user enters at anatomic landmarks. We measured the RV volume using KBR from magnetic resonance images in 20 patients with repaired tetralogy of Fallot at end-diastole and end-systole. We entered points in the long- and short-axis and/or oblique views. The true volume was computed by manually tracing the RV borders for 3-dimensional reconstruction using the piecewise smooth subdivision surface method. The reference database included 54 patients with tetralogy of Fallot patients. The KBR values agreed closely with the true values for the end-diastolic volume (r = 0.993), end-systolic volume (r = 0.992), and ejection fraction (EF; r = 0.930). KBR slightly overestimated the end-diastolic volume (4 ± 10 ml, p = NS), end-systolic volume (1 ± 9 ml, p = NS), and EF (4 ± 3%, p = NS). No bias in the error was found by Bland-Altman analysis (p = NS for end-diastolic and end-systolic volume and EF). The KBR volumes had approached the true volumes (235 ± 93 vs 243 ± 93, p = 0.012, r = 0.978 for end-diastolic and end-systolic volumes combined) already after the first run and the entry of 19 ± 3 points. In conclusion, KBR provided accurate measurement of the RV volume and EF with minimal user input. KBR is a clinically feasible alternative to full manual tracing of the heart borders from imaging data.
Monitoring the right ventricular (RV) volume and function is recommended in the care of patients with repaired tetralogy of Fallot (TOF). However, measurement of the RV volume and function using 2-dimensional echocardiography is inaccurate. Knowledge-based reconstruction (KBR) enables rapid 3-dimensional reconstruction of the right ventricle without requiring border tracing or image processing. The user enters points at anatomic landmarks on the valves, apex, septum, and free wall. The points are used to create a 3-dimensional surface with the aid of a database that embodies knowledge of the shape of the human right ventricle in both health and disease states. The KBR method was previously validated for the left ventricle. We tested the accuracy and reproducibility of KBR for analysis of the right ventricle from magnetic resonance images in patients with repaired TOF.
Methods
The Database for Congenital Heart Disease is a prospective study to collect a resource for 3-dimensional KBR of the right and left ventricles in patients with congenital anomalies. The present study reports the results obtained from testing TOF.
The KBR database included data from 110 patients. Of these, 54 patients had the principal diagnosis of repaired TOF. The others were normal subjects or patients with diverse diagnoses affecting the right ventricle who had undergone magnetic resonance imaging or freehand 3-dimensional echocardiography as a part of other studies. The patients with TOF were recruited at our institutions (except for Ventripoint, Seattle, Washington). These patients had undergone magnetic resonance imaging for clinical indications. All were selected solely by diagnosis without regard to age, clinical history, or severity of pulmonary regurgitation. The intent in selecting the patients with TOF was to include patients who had undergone different surgical procedures, who had a range of severities of tricuspid and pulmonary regurgitation, and whose outflow tracks had dilated to varying degrees to populate the database with a wide variety of RV shapes. The Human Subjects Committee at the University of Washington and at each institution approved the study. The prospectively recruited subjects gave informed consent. Other patients’ data were obtained through retrospective record review by the site investigators. All data were de-identified in accordance with institutional review board regulations before submission to the Core Laboratory at University of Washington (Seattle, Washington).
Magnetic resonance imaging was performed using steady-state, free procession, cine gradient echo sequences, appropriate for the hardware available at each institution. A total of 8 to 12 consecutive, short-axis slices covering both the left and right ventricles were acquired, with a 6- to 10-mm slice thickness, depending on patient size. In addition, long-axis slices were obtained in 4-chamber orientation, in radial long-axis views rotating around the left ventricular long axis, and/or in RV long-axis view to aid in delineating the valves and apex.
A piecewise smooth subdivision surface (PSSS) was used to reconstruct the right ventricle for the database and to define the true RV volume at end-diastole and end-systole. (PSSSs were used in the Pixar movies “Toy Story II” and “Monsters Inc.”) The University of Washington’s version was developed to avoid the inherent limitations of other methods that restricted their anatomic accuracy or application to different 3-dimensional image acquisition techniques. The PSSS reconstruction method has been shown to accurately measure the left ventricular and RV volumes, enables labeling of anatomic landmarks, and is the only method shown to reproduce the ventricular shape with anatomic accuracy.
The PSSS method accepts input from any combination of views. Thus, the basal structures and apex can be defined from long-axis views where they are best visualized, the body of the ventricle can be defined from short axis views that lie orthogonal to the wall, and any difficult anatomic feature such as the repaired RV outflow tract can be defined from the views that best display it, regardless of orientation.
The magnetic resonance imaging studies were reviewed, and the time of the minimum RV chamber area was manually selected in each view. Global end-systole was defined as the most frequently occurring systolic interval and was applied to all views. The borders of the right ventricle and valve orifices and other anatomic landmarks were manually traced using custom software ( Figure 1 ). The papillary muscles and trabeculae were included in the chamber volume. The border points were fitted with a spline curve and then converted to the x, y, and z coordinates using the image position and orientation data. The right ventricle was then reconstructed using the PSSS method ( Figure 1 ).
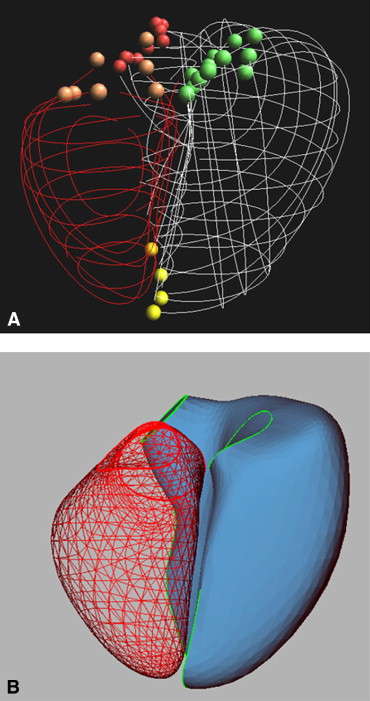
In the PSSS method, a triangulated control mesh is designed as a model for the RV endocardium. The method allows parts of the control mesh to be marked as sharp, such as around the valve orifices and along the RV free wall to the septal edge in the final PSSS. The control mesh is fit to the manually traced borders of the ventricle, valves, apex, and other anatomic landmarks in a process that minimizes a penalized least squares criterion that trades off fidelity to the borders against surface smoothness. The mitral and tricuspid annuli are fitted using a 4-term Fourier series approximation. The points traced from the aortic and pulmonary valves are fit to a circle.
Reconstruction by the PSSS method takes 10 ± 8 minutes using an Intel 3.4-GHz processor (i386) with 1 GB of RAM. The RV volume was computed as the sum of the signed volumes of the tetrahedra formed by connecting a point in space with each face.
The PSSS method is a research tool and is not intended for clinical application. We devoted approximately 5 hours for each 3-dimensional magnetic resonance imaging study of the left and right ventricles to obtain an accurate representation of the 3-dimensional RV shape, because the KBR method depends on shape analysis. We reviewed each border in 3 dimensions immediately after tracing for compatibility with previously traced borders, evaluated the border intersections to ensure concordance with intersecting views, and verified the image plane registration. The long analysis period was also related to the large number of views traced (range 14 to 39, mean 21 ± 6).
The RV volume and ejection fraction (EF) were also measured using the method of disk summation from the short-axis “stack” for comparison with the KBR. Tracing was performed without assistance from the long-axis or oblique images in delineating the basal and apical “slices.”
Interobserver variability of the PSSS method was analyzed in 10 studies randomly selected from the database, including 2 normal volunteers, 4 patients with repaired TOF, 1 with repaired pulmonary atresia, and 3 with repaired transposition of the great arteries.
The database was created by translating, rotating, and scaling the PSSS reconstructions of the 110 patients to accelerate matching to input points in the KBR process. The test population of 20 patients with TOF was selected from the 54 patients in the database using a random number generator. Studies with image plane misregistration were excluded. Each KBR test was performed with a database that was “blinded” to the test patient’s results, by deleting the test patient’s data from the database during their analysis. The KBR software was not trained on the database in the manner of automatic segmentation algorithms; therefore, removal of the test subject from the database removed all knowledge of that test subject’s anatomy from the KBR analysis. This testing by cross-validation was used to maximize the size of the database, because preliminary testing showed that a database of 25 studies was inadequate to represent the variability of RV shape in patients with TOF.
The KBR analysis was performed using VentriPoint Medical System (VentriPoint, Seattle, Washington). The user selected the views containing the key anatomy and then entered points at the landmarks. In the initial run, 16 points were selected: 2 for each valve, 1 at the crista supraventricularis from an inflow-outflow track view, 1 at the apex, 2 on the septum, and 3 distributed around the free wall from the 4-chamber view. One point each was also placed on the lateral and inferior aspects of the free wall from a basal short-axis view, with additional points placed on the lateral free wall and septum from a more apical short-axis view. In 8 patients, additional points were selected in the short-axis views in the initial run to test whether accuracy or efficiency improved.
After point selection, a PSSS was computed as a weighted sum of surfaces in the database ( Figure 2 ). The weights were determined by shape similarity using an optimization routine that minimizes the distance from the entered points to the surface. The RV volume was computed as the sum of signed volumes of tetrahedra formed by connecting a point in space with each face. The run time for KBR was 5 seconds.
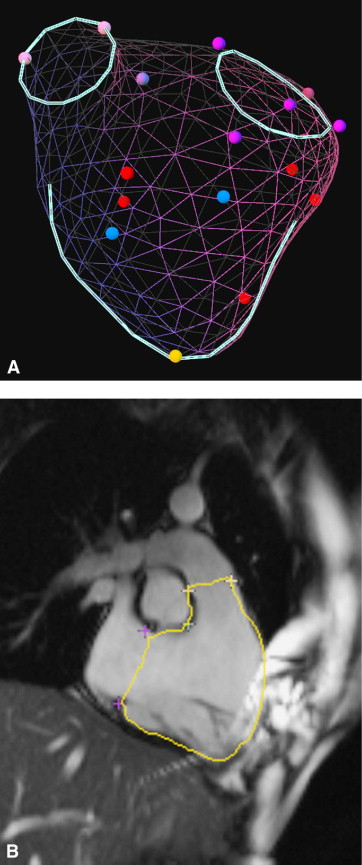
The borders at which the KBR surface intersected the image planes were then displayed on the images ( Figure 2 ). The user could accept the surface or edit the points and rerun the fitting process until a satisfactory surface had been obtained.
To save time, the KBR point selection was limited to 14 views that visualized all regions of the right ventricle. We had recently found that the RV volume could be measured accurately by the PSSS method using 6 short-axis views, 2 inflow-outflow track views, and 6 long-axis views that visualize the free wall from its inferior to superior aspects.
The KBR analysis was performed on one workstation positioned adjacent to a second workstation displaying the borders traced for PSSS analysis of the same study. This protocol was used to enable measurement of the accuracy of the KBR method without interference from interobserver variability in image interpretation.
The selection of points and surfaces was performed by a single observer. Interobserver variability testing was performed by a second observer. Both observers were unaware of the true volume during the KBR analysis.
The volume and EF values measured by KBR were compared with the true values measured by PSSS using linear regression analysis and a paired t test. Bias was investigated using Bland-Altman analysis. The coefficient of variability between observers for KBR analysis was expressed as the SD of the difference between observers divided by the true value. For PSSS analysis, the coefficient of variability between observers was expressed as the SD of the difference divided by the mean. Because of the large range of volumes, the magnitude of variability was also computed as the absolute difference between observers, normalized by the true or mean values, respectively, for KBR or PSSS analysis and expressed as a percentage. Agreement between observers was assessed using intraclass correlation coefficients estimated using analysis of variance models. The absolute value of the difference in volume and EF between the PSSS and KBR methods was compared with the absolute difference between values measured by PSSS and by the method of disk summation using a paired t test. The values are presented as the mean ± SD. Statistical significance was assumed for p <0.05.
Results
The test population included 20 patients (12 males and 8 females) with a repaired TOF. Their age ranged from 17 to 54 years (mean 33 ± 11). Of the 16 patients for whom surgery data were available, the average age at the repair was 4 ± 3 years; 6 patients had had a Blalock-Taussig or Waterston shunt before repair, and 4 had undergone pulmonary valve procedures after repair.
The true RV end-diastolic volume ranged from 164 to 461 ml, the end-systolic volume from 70 to 337 ml, and the EF from 24% to 57%.
KBR analysis was successfully completed in all studies. The RV end-diastolic and end-systolic volumes and EF as measured by KBR on the final run performed by the user agreed closely with the true values ( Figure 3 and Tables 1 and 2 ). The agreement was unbiased according to the Bland-Altman analysis ( Figure 4 ; p = NS for all).
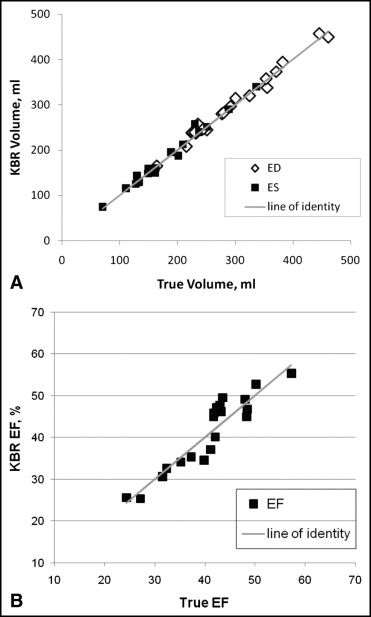
Parameter | n | True Value | KBR Value | Difference (% of True Value) |
---|---|---|---|---|
End-diastolic volume (ml) | 20 | 293 ± 80 | 297 ± 78 | −1.5 ± 3.5 |
End-systolic volume (ml) | 20 | 177 ± 66 | 178 ± 68 | −0.7 ± 4.8 |
Ejection fraction (%) | 20 | 41 ± 8 | 41 ± 9 | −0.8 ± 7.7 |
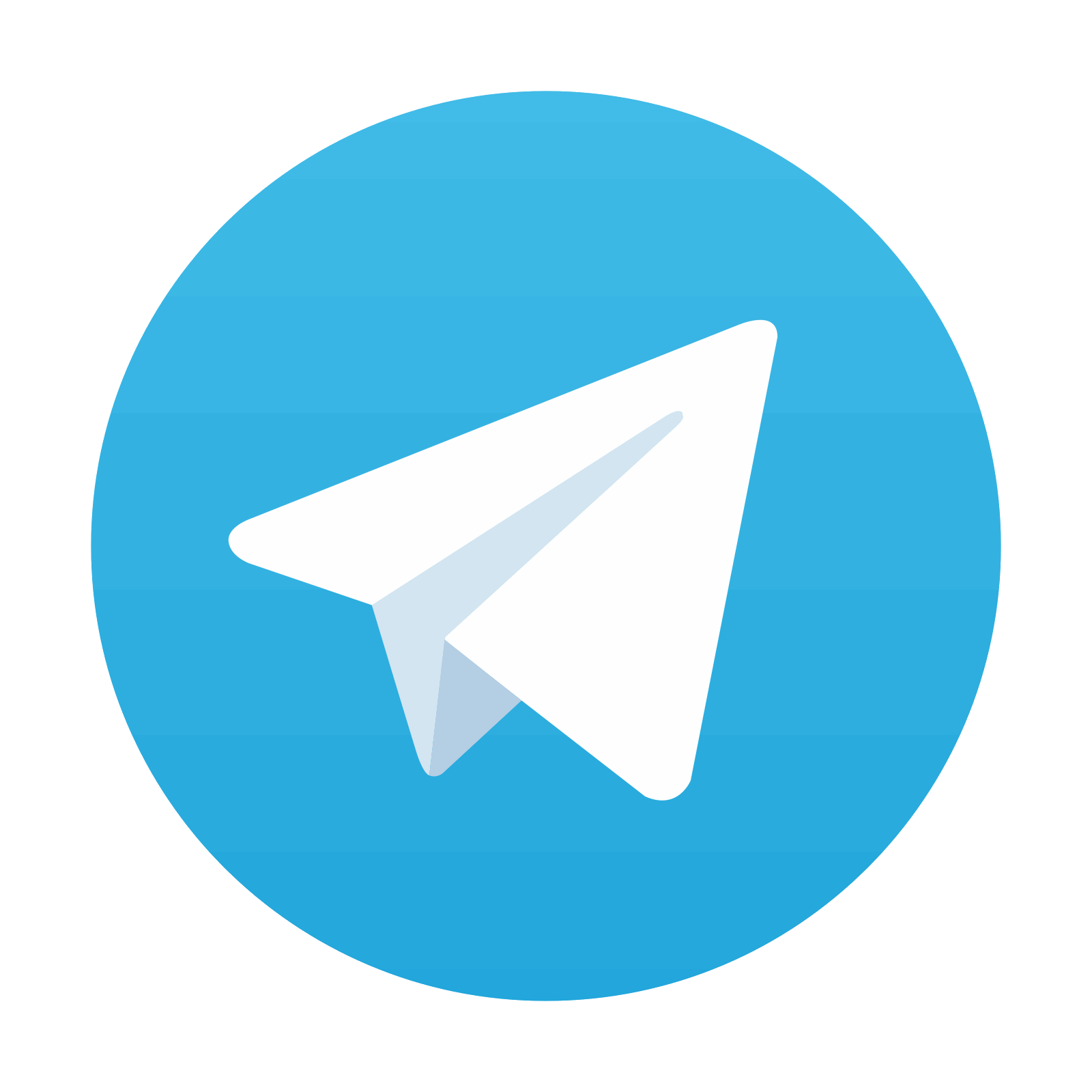
Stay updated, free articles. Join our Telegram channel

Full access? Get Clinical Tree
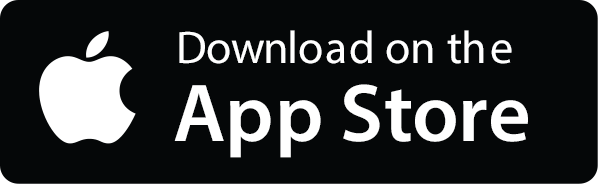
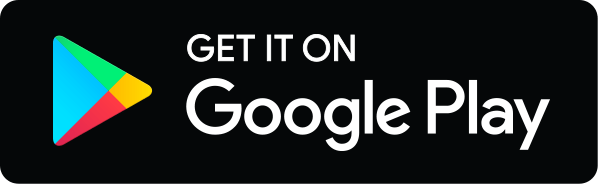
