Background
Right heart function is the key determinant of symptoms and prognosis in pulmonary hypertension (PH), but the right ventricle has a complex geometry that is challenging to quantify by two-dimensional (2D) echocardiography. A novel 2D echocardiographic technique for right ventricular (RV) quantitation involves knowledge-based reconstruction (KBR), a hybrid of 2D echocardiography–acquired coordinates localized in three-dimensional space and connected by reference to a disease-specific RV shape library. The aim of this study was to determine the accuracy of 2D KBR against cardiac magnetic resonance imaging in PH and the test-retest reproducibility of both conventional 2D echocardiographic RV fractional area change (FAC) and 2D KBR.
Methods
Twenty-eight patients with PH underwent same-day echocardiography and cardiac magnetic resonance imaging. Two operators performed serial RV FAC and 2D KBR acquisition and postprocessing to assess inter- and intraobserver test-retest reproducibility.
Results
Bland-Altman analysis (mean bias ± 95% limits of agreement) showed good agreement for end-diastolic volume (3.5 ± 25.0 mL), end-systolic volume (0.9 ± 19.9 mL), stroke volume (2.6 ± 23.1 mL), and ejection fraction (0.4 ± 10.2%) measured by 2D KBR and cardiac magnetic resonance imaging. There were no significant interobserver or intraobserver test-retest differences for 2D KBR RV metrics, with acceptable limits of agreement (interobserver end-diastolic volume, −0.9 ± 21.8 mL; end-systolic volume, −1.3 ± 25.8 mL; stroke volume, −0.2 ± 24.2 mL; ejection fraction, 0.7 ± 14.4%). Significant test-retest variability was observed for 2D echocardiographic RV areas and FAC.
Conclusions
Two-dimensional KBR is an accurate, novel technique for RV volumetric quantification in PH, with superior test-retest reproducibility compared with conventional 2D echocardiographic RV FAC.
Right ventricular (RV) function is the key symptomatic and prognostic determinant in pulmonary hypertension (PH). Cardiac magnetic resonance imaging (CMRI) is the gold standard for volumetric quantification of the right ventricle, but cardiac ultrasound is a comparatively cheaper and more widely available modality. However, the anatomy and complex geometry of the right ventricle confer significant limitations to two-dimensional (2D) echocardiography (2DE). Fractional area change (FAC), for example, is a simple measure of RV size and function that visualizes only one 2D plane of this complex chamber. Three-dimensional echocardiography (3DE) has shown promise for RV volumetric analysis in PH but requires operator experience for acquisition and postprocessing beyond that of 2DE, with lower spatial and temporal resolution, typically leading to underestimation of RV volumes.
A novel 2D echocardiographic technique for volumetric RV quantitation involves knowledge-based reconstruction (KBR). This hybrid approach uses the benefits of conventional 2DE in conjunction with a reference library of RV shapes to reconstruct a 3D RV polygon. The feasibility and accuracy of 2D KBR has been demonstrated in a small PH population, but the ability to accurately identify changes in RV function in response to treatment is also of clinical and prognostic significance. This will depend on the acquisition and postprocessing elements of 2D KBR that both contribute to its variability. We therefore sought to provide further validation data for 2D KBR RV quantification in PH and to investigate the test-retest reproducibility of this novel technique compared with FAC.
Methods
Study Population
We performed a prospective cross-sectional study that enrolled 28 patients in sinus rhythm with no contraindications to magnetic resonance imaging who presented for diagnosis and/or follow-up of PH (diagnosed by right heart catheterization as a mean pulmonary artery pressure > 25 mm Hg and a pulmonary capillary wedge pressure < 15 mm Hg ). All participants underwent comprehensive 2D transthoracic echocardiography and CMRI on the same day (median scan interval, 116 min; interquartile range, 104–150 min). The etiologies of PH were idiopathic ( n = 5), connective tissue disease associated ( n = 14), chronic thromboembolic disease ( n = 8) and portopulmonary ( n = 1). Exclusion criteria were arrhythmia and known independent left-sided cardiac disease unrelated to PH.
The study complied with the Declaration of Helsinki. The institutional research ethics committee approved the study, and informed written consent was obtained from all participants.
Two-Dimensional Echocardiography and KBR
Image Acquisition
All patients underwent comprehensive 2D and Doppler transthoracic echocardiography in the left lateral decubitus position using the Philips iE33 echocardiographic system (Philips Medical Systems, Andover, MA) with an S5-1 transducer (frequency bandwidth, 1–5 MHz). A standard clinical protocol for all examinations was followed in conjunction with American Society of Echocardiography guidelines for chamber quantification.
A magnetic localizer was attached to the S5-1 transducer by a molded plastic sheath. The magnetic localizer was connected to a dedicated console, from which a mechanical arm with an attached magnetic field generator hung over the patient ( Figure 1 ; VentriPoint Diagnostics Ltd, Seattle, WA). The localizer mounted on the ultrasound transducer detects orthogonal magnetic fields from the generator hanging over the patient, and in this manner the ultrasound probe position is localized in 3D space at the point of any 2D acquisition. A cushioned wedge was placed on the echocardiography couch to ensure that the metallic couch apparatus did not interfere with the magnetic field, and patients were instructed to remain entirely stationary in the left lateral decubitus position for the duration of a study acquisition. The ultrasound depth required to visualize all relevant structures was determined before commencing the study and remained fixed throughout.

Seven 2D transthoracic echocardiographic views were obtained in all subjects: parasternal long axis, parasternal short axis at the papillary muscle and apical levels, parasternal RV inflow, parasternal RV outflow including pulmonary valve hinge points and infundibulum, apical four chamber, and an off-axis RV apical view. The 2D KBR acquisition from each view consists of a 2-sec period (usually containing two or three heartbeats) acquired during end-expiratory breath-holds. The electrocardiograph was connected to the echocardiographic system via the dedicated 2D KBR console, and the console images were reproduced from the echocardiographic system’s video output and digitized at 30 frames/sec. Image quality was subjectively graded on a 5-point scale from 0 (very poor) to 4 (perfect).
Postprocessing: RV FAC
End-diastolic and end-systolic frames were assigned by visual identification of the largest and smallest RV four-chamber cavity areas, respectively, on the 2D KBR console. These frames were exported to the open-source OsiriX Digital Imaging and Communications in Medicine software for the measurement of RV FAC by tracing the RV endocardium in both frames and using the formula [(end-diastolic area − end-systolic area)/end-diastolic area] × 100.
Postprocessing: 2D KBR
The largest and smallest RV four-chamber cavity areas were visually identified as end-diastole and end-systole, respectively, on the 2D KBR console, with the software subsequently assigning the same time interval between these frames to all other views. On the 2D KBR console, a series of anatomic RV landmarks were identified on the 2D echocardiography images ( Figures 2A–2G ) in the end-diastolic frames and subsequently in the end-systolic frames. A minimum of 26 points was plotted for each of the end-diastolic and end-systolic data sets. RV endocardial points were placed at the junction between trabeculations and myocardium. The plotted anatomic landmarks with their respective 3D spatial coordinates were then submitted via the Internet to a secure remote server for remote processing by a proprietary 2D KBR algorithm. The algorithm interpolates between the plotted points by referencing against a catalogue of RV shapes generated by CMRI from patients with known diagnoses of PH.

End-diastolic and end-systolic 3D models of the right ventricle were reviewed in a systematic fashion. Intersections between the borders of the 3D model and the original 2D scan plane were inspected to ensure concordance between 2D images and 3D reconstructions ( Figures 2A–2G ), and marked points were checked for alignment with the surface of the 3D model. Where significant deviations between the reconstructed model and either the plotted points and/or 2D echocardiographic endocardial borders existed, points were replotted and the algorithm was rerun. Where significant border versus 2D image misalignment suggested a shift in patient position or an inadequate breath-hold, all points from that 2D view were removed, and the erroneous 2D echocardiographic view was excluded from the 2D KBR reconstruction. A maximum of one view of the seven required in the data acquisition protocol could be excluded for any given study because of a change in patient position or an inadequate breath-hold. If this problem was encountered in more than one of the seven required views, the entire study was excluded from the final analysis.
The final check entailed inspection of the nested view of end-diastolic and end-systolic models to verify alignment of the tricuspid and pulmonary annular planes ( Figure 2 H). The final 2D KBR polygon was assessed for precision by subjectively scoring on a 5-point scale depending on the proximity of intersections of the plotted landmarks with the reconstructed polygon: 4 (all points intersect), 3 (three or fewer points significantly deviate from polygon), 2 (five or fewer points significantly deviate from polygon), 1 (seven or fewer points significantly deviate from polygon), and 0 (poor agreement).
FAC and 2D KBR Test-Retest Reproducibility
All subjects underwent serial 2D echocardiographic acquisition and postprocessing by two independent sonographers (D.S.K. and J.P.S.), as described previously. The two sonographers had similar experience in 2D transthoracic echocardiography (>4 years each) and received the same vendor training for the 2D KBR system. Sonographer 1 (D.S.K.) obtained a 2D KBR data set, after which sonographer 2 (J.P.S.) independently obtained a 2D KBR data set. Sonographer 1 then acquired a second 2D KBR data set. The sonographers, who were blinded to each other’s results and the results from CMRI, performed postprocessing of their own data sets for FAC and 2D KBR. Data sets analyzed for intraobserver test-retest reproducibility were postprocessed separately at time intervals of >2 weeks.
CMRI
Image Acquisition
All CMRI images were acquired using a 1.5-T magnetic resonance scanner (Avanto; Siemens Healthcare, Erlangen, Germany) using a 12-element phased-array coil for signal reception and the body coil for signal transmission. A vector electrocardiographic system was used for cardiac gating. In all patients, ventricular volumes and great vessel flow were measured as previously described. Volumetric RV data were obtained using real-time radial k – t sensitivity-encoded imaging of contiguous transaxial slices. Real-time radial k – t sensitivity-encoded imaging allows the collection of high–spatiotemporal resolution, real-time images during free breathing and is part of the standard clinical CMRI work flow at our institution in the pediatric PH population.
Postprocessing
All image postprocessing was performed using “in-house” plugins for the open-source OsiriX Digital Imaging and Communications in Medicine software. Endocardial RV borders were traced manually at end-diastole and end-systole, the time points of which were identified by the largest and smallest RV cavity areas, respectively. The inclusion of RV trabeculations was the same as that performed in echocardiographic postprocessing. Ventricular stroke volume was the difference between the end-diastolic volume and end-systolic volume, and ejection fraction was calculated as (stroke volume/end-diastolic volume) × 100.
Statistical Analysis
Statistical analysis was performed using SPSS version 22.0 (IBM Corporation, Armonk, NY) and Prism version 6.0b for Mac (GraphPad Software, Inc, La Jolla, CA). All continuous data were normally distributed and expressed as mean ± SD. Systematic differences between measurements were evaluated with Student’s paired t test (two tailed). P values < .05 were considered to indicate statistical significance. Intermodality agreement was studied using the Bland-Altman method, whereby the mean difference was presented as the bias and 95% limits of agreement around the bias expressed as the mean difference ± 1.96 SDs.
Differences between test-retest measurements were analyzed using one-way repeated-measures analysis of variance, with the Bonferroni post hoc test identifying which specific means differed. The Greenhouse-Geisser correction was used if the assumption of sphericity had been violated. Test-retest variability was expressed using intraclass correlation coefficients, relative differences and coefficients of variation. The intraclass correlation coefficient was quantified by the two-way random-effects model with absolute agreement. An intraclass correlation coefficient > 0.85 was considered excellent. Relative differences were calculated by taking the absolute difference between two observations divided by the mean of the repeated observations and expressed as a percentage. Coefficients of variation were calculated as the SD of the difference between two acquisitions divided by their mean value and expressed as a percentage. A coefficient of variation ≤ 10% was considered excellent.
Results
Population Characteristics and 2D KBR Technical Data
The clinical characteristics of the 28 participants are presented in Table 1 , all of whom had adequate 2D echocardiographic windows for the specified protocol. Participants’ heart rates recorded on the 2D echocardiographic loop acquired first were similar to those recorded on the 2D echocardiographic loop acquired last ( P = .90). Image acquisition for one data set took on the order of approximately 5 min per patient, with 2D KBR postprocessing and analysis taking no longer than about 15 min. Good mean subjective scores were observed for 2D echocardiographic image acquisition (2.9 ± 0.9) and 2D KBR reconstruction (3.2 ± 0.7), with moderate correlation between the two scores ( r = 0.54, P = .003).
Variable | Value |
---|---|
Age (y) | 54 ± 13 |
Women | 20 (71%) |
Height (cm) | 165 ± 11 |
Weight (kg) | 71 ± 18 |
Body surface area (m 2 ) | 1.8 ± 0.3 |
Heart rate (beats/min) | 79 ± 13 |
Mean PASP on RHC (mm Hg) | 47 ± 12 |
Pulmonary vasodilators | |
Endothelin antagonists | 11 (39%) |
PDE 5 antagonists | 18 (64%) |
Oral prostanoid | 1 (4%) |
Inhaled prostanoid | 1 (4%) |
RV EDV (mL/m 2 ) | 98 ± 26 |
RV ESV (mL/m 2 ) | 59 ± 23 |
RV EF (%) | 41 ± 11 |
RV Quantification by 2D KBR versus CMRI
RV volumes and ejection fractions for all participants measured by 2D KBR showed no significant differences with CMRI ( Table 2 ), with no significant bias and clinically acceptable limits of agreement ( Figure 3 ).
Measurement | 2D KBR | CMRI | P ∗ |
---|---|---|---|
RV EDV (mL) | 179 ± 66 | 176 ± 61 | .16 |
RV ESV (mL) | 107 ± 47 | 106 ± 47 | .63 |
RV SV (mL) | 73 ± 27 | 70 ± 26 | .26 |
RV EF (%) | 42 ± 10 | 41 ± 11 | .66 |

Test-Retest Intraobserver and Interobserver Reproducibility
One patient moved in the first data set acquisition, one patient moved in the third data set acquisition, and two patients moved in both the second and third data set acquisitions. The 2D KBR data sets for these four individuals were therefore excluded from the final test-retest reproducibility analysis because of significant movement artifact.
Good reproducibility metrics and acceptable limits of agreement were observed for the 24 intra- and interobserver 2D KBR test-retest studies ( Table 3 , Figure 4 ). There were no significant differences for RV volumes or ejection fraction between serial 2D KBR studies, but significant intra- and interobserver test-retest variability was demonstrated for serial RV areas and FAC ( Table 4 ).
Variable | Intraobserver | Interobserver | ||||
---|---|---|---|---|---|---|
ICC | COV (%) | RD (%) | ICC | COV (%) | RD (%) | |
2D KBR | ||||||
RV EDV | 0.985 | 3.0 | 4.2 | 0.986 | 3.9 | 5.5 |
RV ESV | 0.987 | 4.3 | 6.1 | 0.960 | 7.7 | 10.9 |
RV SV | 0.953 | 8.3 | 11.7 | 0.856 | 11.7 | 16.5 |
RV EF | 0.919 | 6.4 | 9.0 | 0.758 | 10.5 | 14.8 |
2DE | ||||||
RV EDA | 0.885 | 9.0 | 12.7 | 0.394 | 25.0 | 35.4 |
RV ESA | 0.931 | 9.3 | 13.2 | 0.440 | 31.0 | 43.9 |
RV FAC | 0.784 | 18.1 | 25.6 | 0.619 | 20.8 | 29.4 |
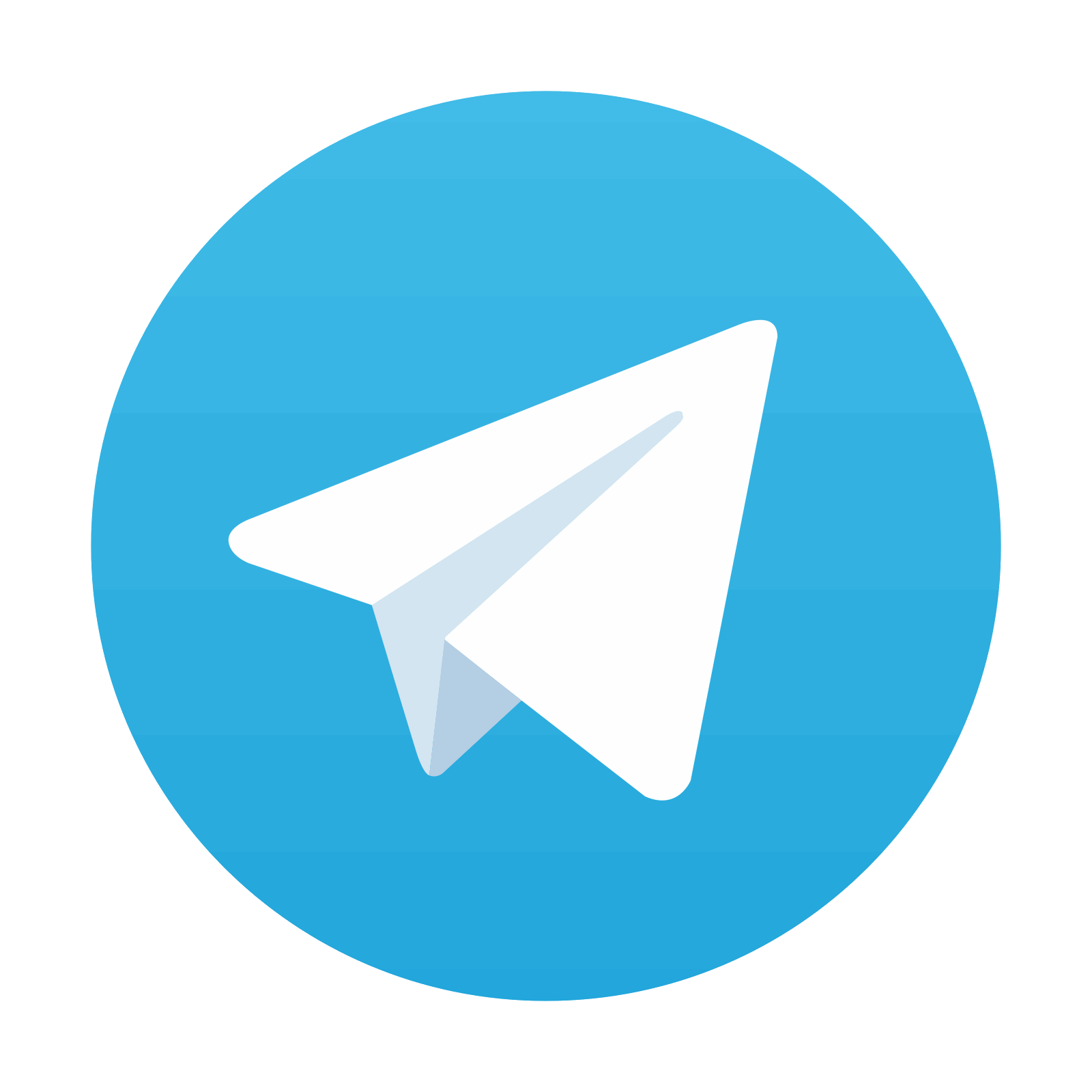
Stay updated, free articles. Join our Telegram channel

Full access? Get Clinical Tree
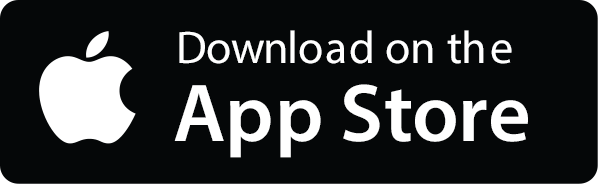
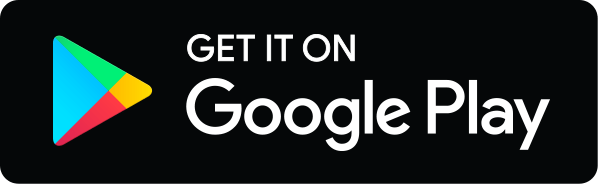
