Fig. 19.1
An ulcerated skin ulcer directly overlying an arteriovenous fistula. Hemorrhage from the ulcer is being controlled with manual pressure immediately before surgical repair in the operating room
The presence of a skin ulcer or eschar overlying a vascular access requires prompt surgical intervention. Attempts to suture the friable surrounding tissue will inevitably fail and may precipitate more bleeding by accelerating the rate of skin necrosis. Jaffers and Fasola recently reported uniform success in salvaging ulcerated, bleeding autologous fistulas [11]. In most cases, they excised the skin ulcer and primarily repaired the underlying fistula opening. Aneurysmal segments required plication after resection of the anterior fistula wall. All of the fistulas remained functional allowing uninterrupted dialysis. Salvaging an ulcerated AV graft involves excising the overlying skin and tunneling an interposition prosthetic bypass around the area of skin breakdown. Continued dialysis depends on whether the revised AV graft still has a usable segment for cannulation. A temporary dialysis catheter may be required until the interposition bypass has healed allowing more space for needle punctures.
Venous Stenosis
Venous stenosis remains the Achilles heel of vascular access. Triggered by several risk factors, venous stenosis is a common complication that responds poorly to endovascular intervention with a high rate of recurrence. Despite advances in hemodialysis surveillance and endovascular techniques, venous stenosis causes more AV access failures than any other complication. AV grafts typically fail due to stenosis at the venous anastomosis, while fistulas can develop stenosis anywhere including the arterial anastomosis, venous outflow, central veins, and superior vena cava. Depending on the location and severity of the lesion, venous stenosis can have a wide range of consequences including arm edema, superior vena cava syndrome, prolonged bleeding, aneurysm and pseudoaneurysm formation, ineffective dialysis, and vascular access thrombosis. The high prevalence of venous stenosis (16–50 %) coupled with being recalcitrant to definitive intervention creates one of the most vexing problems for vascular access. Management guidelines for venous stenosis therefore consist of prevention, palliative endovascular interventions, and surgical bypass in selected cases.
Central venous catheters remain the most prominent risk factor for the development of central venous stenosis. Catheters injure the venous endothelium inciting an inflammatory and thrombotic response. A vein’s response to injury can evolve over time into an organized thrombus associated with smooth muscle proliferation, vessel wall thickening, and formation of a bridge to the catheter. The injury induced by the catheter coupled with the vein’s hyperplastic response may create a precursor stenotic lesion which would explain the strong association between central venous catheters and venous stenosis [12]. Other high-risk factors for venous stenosis include multiple catheter placement, long dwell times, and subclavian vein location; however, all catheters, regardless of location or duration, have been linked to venous stenosis. Several studies have documented venous stenosis following internal jugular vein catheters, and even short-term catheters can incite thrombosis, fibrin sheath formation, and stenosis [13]. Therefore, the most effective way to prevent venous stenosis is to avoid the use of central venous catheters altogether [14, 16].
The diagnosis of venous stenosis relies on clinical assessment, hemodialysis monitoring, and imaging studies. Signs and symptoms of venous stenosis include arm edema, loss of a palpable thrill, access pulsatility, and prolonged bleeding after needle decannulation. Dialysis centers track objective measures of access and recirculation over time. Findings which suggest venous stenosis including persistently low flow (less than 600 ml/min), high venous pressure, or ineffective dialysis should prompt imaging studies for confirmation [17, 18]. Duplex ultrasound of the access can often detect venous stenosis; however, it offers limited, indirect evaluation of the central veins. A fistulogram has the highest sensitivity for diagnosing venous stenosis since it can image the entire vascular access from the arterial anastomosis to the right atrium [19].
In addition to being diagnostic, fistulograms also provide an opportunity for endovascular intervention. Balloon angioplasty to dilate stenotic lesions in peripheral and central veins has an immediate technical success rate of 88–94 % [20]. Most peripheral venous lesions require overdilation with angioplasty balloons 10–20 % larger in diameter than the vein being treated. Unlike atherosclerotic arterial disease, stenotic venous lesions consist of endothelial hyperplasia and fibrous tissue [21]. These histologic features may explain why venous stenoses tend to resist balloon angioplasty and recoil after dilation in as many as 64 % of patients as determined by intravascular ultrasound [22]. If the post-angioplasty images show persistent stenosis or immediate recoil, endovascular treatment options include repeat angioplasty using a high-pressure or cutting balloon or stent placement depending on the lesion type and location. Centrally located lesions require a more cautious approach to avoid the risk of potentially fatal central vein perforation [23]. Determining the appropriate balloon size for central venous lesions can be challenging because two-dimensional contrast images often underestimate the true vein diameter. Intravascular ultrasound measures the vessel lumen more accurately and may help in choosing a sufficiently large diameter balloon that will not overdilate the vein. Stent placement can play a role in treating elastic lesions of the central veins that fail to respond to balloon angioplasty or recur within 3 months of treatment [24]. Using self-expanding stents that are slightly larger than the diameter of the vein will assure full-wall apposition and minimize the risk of stent migration. An intravascular ultrasound or pre-procedure CT scan of the thorax provides accurate measurements of the true central vein diameter and ensure appropriate stent sizing.
Limited durability remains the most significant disadvantage of endovascular therapy for venous stenosis. Primary patency following balloon angioplasty for central venous stenosis falls to 25 % and 17 % after 6 months and 12 months, respectively [25]. Balloon angioplasty for peripheral veins results in a similar, slightly less steep, decline in short- and midterm patency. The benefit of stenting appears to be confined to salvaging immediate technical success after an unsatisfactory response to balloon angioplasty [26]. Stents placed in peripheral and central veins fail to prolong primary and secondary patency and may accelerate restenosis in some locations.
The short-lived success of endovascular therapy for venous stenosis mandates regular post-intervention follow-up. Clinical evidence of restenosis including the reappearance of arm edema, prolonged bleeding, high venous pressures, and ineffective dialysis should prompt further investigation with a fistulogram. The treatment of recurrent venous stenosis depends on the time interval to restenosis and the lesion location. Repeated balloon angioplasty may be the most reasonable treatment strategy for patients with peripheral or central venous stenosis who can achieve effective dialysis for at least 3–6 months between endovascular interventions. Although it is not definitive, endovascular therapy can prolong effective patency and allow these patients to avoid or delay the need for surgery and catheter placement.
Surgical revision should be considered if endovascular treatment fails or the stenosis recurs within a short period of time (less than 3 months). Surgical options depend on the extent of the lesion, its location, and the superficial and central venous anatomy. For peripheral venous stenosis, surgery to reestablish venous outflow usually involves patch angioplasty of the venous outflow (Fig. 19.2) or an interposition bypass of the lesion using prosthetic graft (Fig. 19.3). Other surgical options include mobilizing and reimplanting the distal AV fistula on a patent vein or translocating the basilic or cephalic vein. Likewise, a variety of techniques to manage recalcitrant subclavian vein lesions have been described including axillary to internal jugular vein bypass, internal jugular to axillary vein transposition, axillary to saphenous vein bypass, and patch angioplasty of the axillosubclavian vein stenosis [27]. A direct bypass to the right atrium offers a solution to central venous occlusion; however, the morbidity and requirement for a thoracotomy makes this procedure appropriate only for highly selected patients with few remaining vascular access options [28, 29].
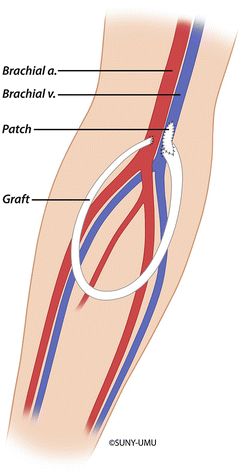
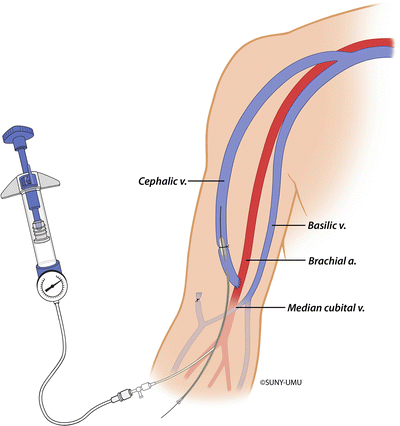
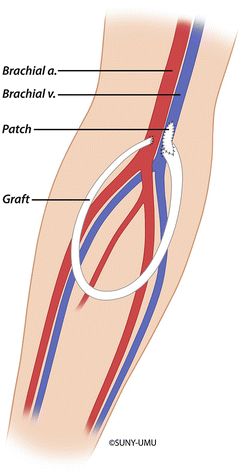
Fig. 19.2
Surgical management of venous outflow stenosis with patch angioplasty of the venous anastomosis
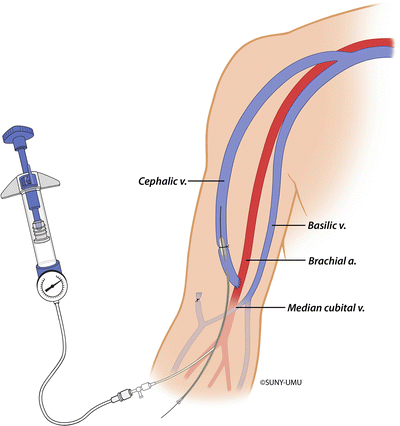
Fig. 19.3
Surgical management of venous outflow stenosis with interposition bypass to a more proximal vein using prosthetic graft
Venous stenosis at the costoclavicular junction deserves special consideration because of its poor response to balloon angioplasty and stent placement. The musculoskeletal structures surrounding the costoclavicular junction can extrinsically compress the adjacent subclavian vein. Exposure of this anatomically narrowed segment of the vein to turbulent flow from a proximal AV fistula can create a chronic functional injury equivalent to venous thoracic outlet syndrome. According to this theory, endovascular therapy at the costoclavicular junction will fail in the absence of thoracic outlet decompression which usually involves a transaxillary first rib resection. Illig achieved promising early results using a surgical approach for patients with so-called dialysis associated venous thoracic outlet syndrome. He reported an 8-month functional patency of 75 % in a series of 12 selected patients treated with first rib resection [30].
The anatomic area known as the cephalic arch (the final segment of the cephalic vein before its confluence with the axillary vein) is also prone to stenosis and relatively resistant to endovascular intervention (Fig. 19.4). In patients with brachiocephalic AV fistulas, the incidence of cephalic arch stenosis may exceed 77 %, making it a leading cause of vascular access failure [31]. Balloon angioplasty, the widely accepted first-line therapy, has limited durability with a primary 6-month patency of only 42 % [31, 32]. The unique anatomy of the cephalic arch may explain its tendency to form recalcitrant stenotic lesions [33]. After the creation of an AV fistula, all veins react to the hemodynamic changes with intimal hyperplasia and wall thickening. Peripheral veins can usually preserve their lumen diameter because they have the anatomic freedom to dilate and remodel. In contrast, the cephalic arch’s pathway over the deltoid muscle creates a constriction limiting fistula dilation and accommodation for intimal hyperplasia. Stenotic lesions develop early in the cephalic arch and usually recoil or quickly recur in response to dilation with balloon angioplasty. Endovascular alternatives for treating cephalic arch stenosis including cutting balloon angioplasty and stent graft placement have shown promising early results in small, selected case series [34, 35, 32]. Surgical revision usually involves rerouting the venous outflow around the cephalic arch by transposing the proximal cephalic vein to the basilic or axillary vein.
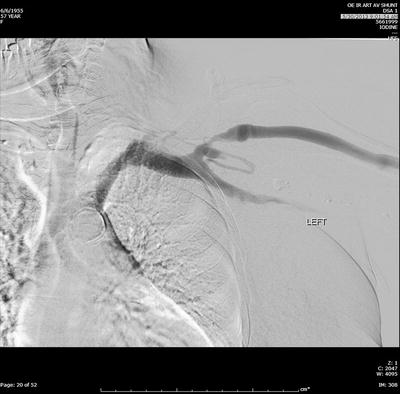
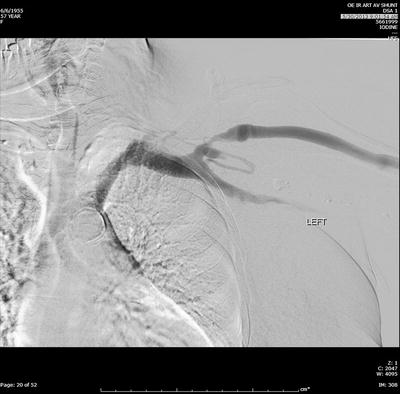
Fig. 19.4
Fistulogram of a left upper arm brachiocephalic AV fistula with stenosis of the cephalic vein in the cephalic arch
Ischemia
Ischemia associated with vascular access goes by several names including hemodialysis access-induced distal ischemia (HAIDI), dialysis-associated steal syndrome, distal hypoperfusion ischemic syndrome, and access-related hand ischemia. All of these terms describe a complication clinically defined as hypoperfusion distal (more peripheral) to an AV fistula or graft [36]. The incidence and severity of HAIDI varies with the type and location of the vascular access. For AV fistulas originating from the brachial artery, the incidence of HAIDI can approach 20 % with over half of the cases classified as severe [37]. Radial artery-based fistulas have a much lower incidence (2 %) and rarely require treatment [38]. The severity of clinical symptoms determines the classification of HAIDI as follows [39]:
Stage 1: retrograde flow without complaints
Stage 2: forearm or hand pain with exertion
Stage 3: forearm or hand pain at rest
Stage 4: ulceration, necrosis, or gangrene
The pathophysiology of HAIDI provides insight into its prevention, diagnosis, and management. Connecting an artery to a vein for vascular access creates a high-flow, low-resistance circuit. Distal perfusion pressure decreases as an increasing volume of arterial blood flows preferentially through the access into the low-resistance venous outflow. Retrograde flow from the distal artery into the access site occurs during all or part of the cardiac cycle further decreasing pressure in the forearm and hand. Although this “physiologic steal” occurs in virtually all AV fistulas and grafts, only a few patients develop significant distal ischemia. The lack of correlation between retrograde flow (steal) and clinical ischemia has fueled speculation that “steal” itself plays only a minor role in the so-called steal syndrome. Scheltinga and Bruijninckx assert that locoregional hypotension, not retrograde flow or steal, is the most critical factor in the onset of HAIDI [40]. The majority of patients overcome “physiologic steal” and other hemodynamic changes by vasodilating inflow arteries, recruiting collaterals, and increasing cardiac output to augment distal perfusion. Ischemia occurs only when these adaptive mechanisms fail to compensate for distal hypoperfusion. The most common clinical scenarios resulting in HAIDI include excessive access flow, arterial occlusive disease, and lack of arterial adaptation or collateral flow.
The first step in preventing HAIDI involves recognizing which patients are at risk. Diabetes emerged as the most predictive factor for developing ischemia in a multivariate analysis of 324 AV fistulas in 309 patients [41]. Other clinical predictors of HAIDI include brachial artery-based access, female gender, age greater than 60, low digital brachial index, and previous operations on the same limb. Unfortunately, none of the preoperative predictors of ischemia has enough predictability to preclude creating an AV access in an “at-risk” patient.
Basing an AV access on the radial artery will decrease the ischemic risk; however, the non-maturation rate increases, and not all patients have an adequate caliber radial artery at the wrist. Whittaker and Bakran reported a low rate of ischemia (2 %) using the proximal radial or ulnar artery as arterial inflow [42]. Through a longitudinal incision in the proximal forearm, they create an end-to-side anastomosis between the median cubital vein and the proximal radial or ulnar artery. This technique offers a reasonable alternative to using inflow from the brachial artery for patients who cannot have a wrist fistula. If the brachial artery must be used, limiting the length of the AV anastomosis to less than 10 mm decreases the risk of developing excessive flow through the access.
Symptoms of HAIDI have a bimodal distribution with half of patients presenting acutely (within 7 days of surgery) and the other half following a more chronic course (presenting after 30 days) [43]. In the acute presentation, the signs and symptoms of HAIDI mirror the classic 6 Ps of acute lower extremity ischemia, namely, pulselessness, pain, pallor, poikilothermia, paresthesias, and paralysis. Chronic symptoms of HAIDI emerge months to years after surgery and include arm fatigue with exertion, hand pain at rest, and tissue loss. Often the tissue loss occurs due to what would otherwise be insignificant skin trauma. The clinical diagnosis of HAIDI relies on both the history and physical examination. Physical exam of a patient with HAIDI usually demonstrates an absent wrist pulse that returns with manual compression of the access; however, this finding cannot be used as the sole diagnostic criteria for HAIDI. In practice, many patients lack a palpable wrist pulse after AV access creation while maintaining adequate perfusion to the arm and hand. At the other extreme, some patients with symptoms of ischemia have a palpable wrist pulse at rest. This apparently contradictory presentation suggests that the upper extremity can develop ischemic symptoms at relatively higher absolute pressures [44].
The timing and type of treatment for HAIDI depend on several factors including symptom severity, type of access, and the patient’s clinical condition [45]. Patients with mild symptoms warrant close observation as many will improve over time [46]. Moderate and severe symptoms of HAIDI require prompt intervention to improve perfusion and prevent permanent ischemic injury or tissue loss. The treatment options listed in Table 19.1 should be considered complementary as none of the techniques has universal success nor can any be applied to all clinical scenarios. All interventions should primarily focus on reversing ischemia with a secondary goal of salvaging the vascular access.
Table 19.1
Techniques for treating hemodialysis access-induced distal ischemia (HAIDI)
Technique | Advantages | Drawbacks |
---|---|---|
Access ligation | • Maximizes hand perfusion | • Loss of dialysis access |
• Technically simple | • High risk of HAIDI in new access | |
Banding | • Preserves access | • Increases risk of access thrombosis |
• Technically simple | ||
Distal revascularization-interval ligation (DRIL) | • Preserves access | • More demanding technically |
• Brachial artery ligated | ||
Proximalization of arterial inflow (PAI) | • Preserves access | • Less effective in patients with high access flow or tissue loss |
• Brachial artery remains in continuity | • Requires prosthetic conduit | |
Revision using distal inflow (RUDI) | • Preserves access with shorter bypass conduit | • Higher failure rate |
• Brachial artery remains in continuity |
Ligation immediately and reliably reverses ischemia at the cost of sacrificing the vascular access. Although symptoms usually improve quickly, paresthesias can persist due to neuropathy related to the initial ischemic injury. Access ligation may serve as a first-line treatment for acute ischemia after a prosthetic AV graft given a graft’s predictably short patency. At the other extreme, access ligation can also function as a last resort to reverse ischemia in patients who have failed to improve after a previous remedial procedure. The straightforward nature of access ligation also makes this intervention appropriate for patients with severe comorbidities that preclude more extensive revascularization procedures.
Rarely, treatment for HAIDI involves simply correcting arterial inflow stenosis. Prior to creating the access, a thorough preoperative evaluation should detect and treat inflow lesions. Scali and Huber recommend measuring upper extremity arterial pressure and Doppler waveforms on all potential access patients. Their criteria for a suitable inflow artery include adequate diameter (brachial artery >3 mm; radial artery >2 mm) and absence of hemodynamically significant arterial stenosis based on pressures and waveforms [44]. All patients with symptomatic HAIDI should have complete angiographic imaging of all vessels bringing blood to and from the AV access. The procedure begins by puncturing the AV access in the direction of the arterial anastomosis and advancing a directional catheter over a wire through the arterial anastomosis and into the arterial system as far proximally as the aortic arch. Images and pressure measurements at the aortic arch, subclavian artery origin, axillary artery, and brachial artery (proximal, mid, and pre-anastomotic) are then recorded. Any stenotic lesions encountered can be treated with balloon angioplasty. Redirecting the catheter into the distal arterial runoff can then evaluate for occlusive disease in the forearm and hand.
Most patients do not harbor an arterial inflow lesion of such severity that correcting it alone would alleviate all symptoms of ischemia. Usually HAIDI develops from a combination of excessive access flow and hypotension in the distal arterial system. Reversing ischemia and salvaging the vascular access therefore requires interventions that limit access flow and/or increase distal arterial perfusion. Banding describes a variety of techniques that reduce access flow by increasing resistance in the venous outflow. In its simplest form, banding narrows the venous outflow by suture plication or placement of a restrictive prosthetic cuff. The underlying hemodynamic flaw of banding leads to poor long-term access patency rates. A band that restricts flow enough to reverse ischemia often converts the access into a flow-dependent, high-resistance circuit whose natural tendency is to clot [47]. Miller et al. reported improved results with a modification to traditional banding procedures. The MILLER procedure (minimally invasive limited ligation endoluminal-assisted revision) involves inflating a percutaneously placed 3 or 4 mm diameter angioplasty balloon in the proximal venous outflow. Through a small incision, a suture is passed around the access and tied over the inflated balloon narrowing the access to the diameter of the chosen balloon (Fig. 19.5). The precise band sizing achieved by the MILLER procedure may account for the promising midterm results including a 6-month primary band patency of 75–85 % in a selected series of 183 patients [36].
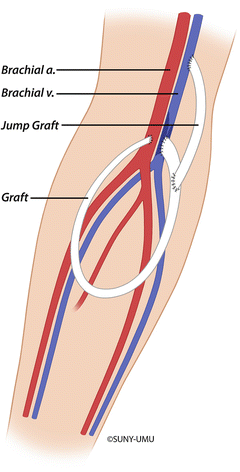
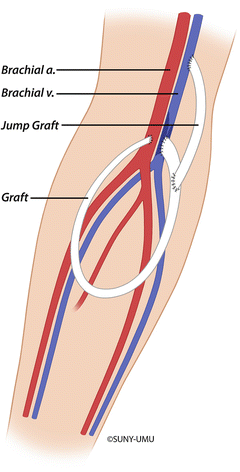
Fig. 19.5
The MILLER procedure for banding an arteriovenous fistula. The percutaneously inserted angioplasty balloon is inflated in the proximal fistula, and a suture is introduced through a small skin incision and tied around the inflated balloon to narrow the vein to the diameter of the chosen balloon
Although banding procedures can decrease access flow, they do not directly increase distal arterial perfusion. The distal revascularization-interval ligation (DRIL) procedure is currently the most accepted method of retaining the AV fistula and improving distal perfusion. DRIL consists of two components in an attempt to address both of these treatment goals. Distal revascularization creates a bypass around the fistula to increase forearm and hand perfusion, while interval ligation of the distal brachial artery cuts off retrograde flow into the access (Fig. 19.6). The great saphenous vein in the thigh and a segment of cephalic or basilic vein from the ipsilateral upper extremity rank first and second in preference as conduits for the brachial bypass. Placing the vein in reverse configuration avoids the need for a valvulotome which can damage thin-walled arm veins. In the absence of an autogenous vein larger than 3 mm in diameter, some access surgeons have reported satisfactory results with a prosthetic graft. In contrast, Scali and Huber avoid using a prosthetic graft opting instead for an alternative revascularization procedure or fistula ligation [44].
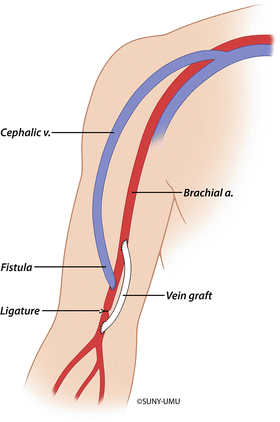
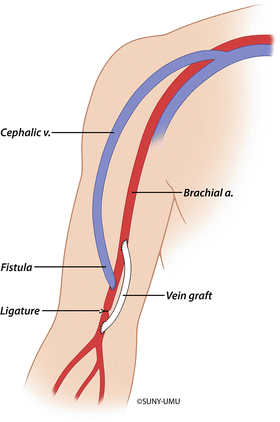
Fig. 19.6
Distal revascularization-interval ligation (DRIL) procedure. A bypass from the proximal brachial artery extends to the brachial artery just distal to the AV fistula anastomosis. The brachial artery is then ligated distal to the AV anastomosis and proximal to the bypass graft
Preparation for a DRIL procedure begins with angiography and brachial artery pressure measurements. In his original description of the DRIL procedure, Schanzer empirically started the brachial bypass 5 cm proximal to the fistula anastomosis [48]. Over the last 25 years, a more clear understanding of the hemodynamics of HAIDI has emerged including the concept of locoregional hypotension. In a series of patients with access-related ischemia, Illig et al. demonstrated a significant pressure drop-off in the brachial artery [49]. They found that this “pressure sink” often extended along the brachial artery more than 5 cm proximal to the fistula anastomosis. To effectively increase distal perfusion, the brachial artery bypass should originate proximal to the “pressure sink.” By measuring the distance from the fistula anastomosis to the pressure drop-off, preoperative pressure measurements can identify an appropriate location for the proximal bypass.
The bypass should terminate on the brachial artery just distal to the fistula anastomosis. If the fistula originates from the very distal brachial artery, the fistula anastomosis should be transposed to the more proximal artery to allow space for the distal bypass anastomosis. After ensuring adequate flow through the bypass, the brachial artery is ligated between the anastomoses of the fistula and the distal bypass. Postoperative monitoring in the form of clinical exam and duplex ultrasound seems to be helpful; however, guidelines for the type and frequency of surveillance do not exist. Anaya-Ayala et al. recommend close follow-up in the 30-day postoperative period, especially in patients with suboptimal bypass conduits and microvascular disease [50].
Despite the technical demands, the DRIL procedure appears to be safe and effective. Wound infection is the most common complication, and Scali et al. reported a 30-day mortality rate of 2 % in the largest series of patients [51]. Outcome data after DRIL procedures vary with the definition of clinical success. Relief of presenting symptoms ranges from 78 to 100 % with higher rates reported by authors who overlook residual paresthesias presumably due to the initial ischemic injury [52, 53]. Concerns about the negative impact of ligating the native brachial artery appear to be unfounded. Primary bypass patency ranges from 73 to 100 % at 1 year, while secondary patency exceeds 80 % in most reports [43]. Long-term durability may not be necessary given the limited survival of patients undergoing the DRIL procedure. Anaya-Ayala et al. reported a 1-year mortality of 61 % due to comorbid illnesses unrelated to the DRIL procedure [50], while only 30 % of patients in Scali and Huber’s series were still alive 2 years after surgery [44].
Although the DRIL procedure is the most well-known technique, other procedures aimed at reversing ischemia and preserving the access have been described. Proximalization of arterial inflow (PAI) inserts an interposition bypass from the proximal brachial artery near the axilla to the original autogenous access in the antecubital fossa. The bypass consists of a 4–5 mm prosthetic graft which is tunneled in the deep tissue of the upper medial arm (Fig. 19.7). Needle cannulation sites remain on the anterior upper arm along the autogenous venous outflow tract. PAI most likely functions as a flow-limiting procedure as the long, small caliber bypass increases resistance in the access thereby improving distal perfusion [44]. In small case series, PAI resolved ischemic symptoms in 65–84 % of patients [54, 55]. These reports suggest that PAI is less effective in high access flow HAIDI and caution against the use of PAI in patients with severe tissue loss. Other concerns about PAI center around the infectious and thrombotic risk of converting a fistula to a composite prosthetic/autogenous access.
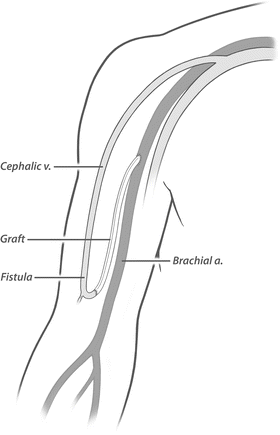
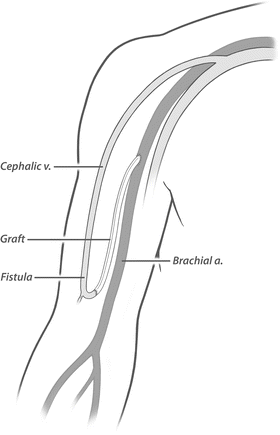
Fig. 19.7
Proximalization of arterial inflow. A long interposition bypass extends from the proximal brachial artery near the axilla to the autogenous access
In contrast to PAI, the revision using distal inflow (RUDI) procedure relocates the arterial inflow to a more distal artery. As a first step, the fistula is ligated and transected close to the brachial anastomosis. A short interposition bypass from the radial or ulnar artery to the fistula is then created using a nearby venous collateral or great saphenous vein graft (Fig. 19.8). By switching arterial inflow from the brachial to the radial or ulnar artery, RUDI decreases flow into the fistula and increases distal perfusion. Advantages of RUDI over the PAI and DRIL procedures include the use of a shorter bypass conduit and preservation of uninterrupted flow through the native brachial artery. Fistula failure is the primary drawback of RUDI as the smaller distal artery may fail to supply adequate inflow to the fistula. Although small case series reported that RUDI resolved ischemic symptoms in nearly all patients, the fistula failure rate ranged from 29 to 43 % [56, 57]. The presence of forearm arterial occlusive disease, especially in the elderly, diabetics, and women, may increase the risk of fistula failure after RUDI.
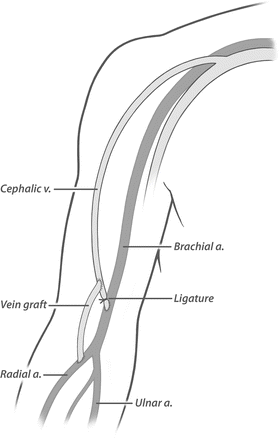
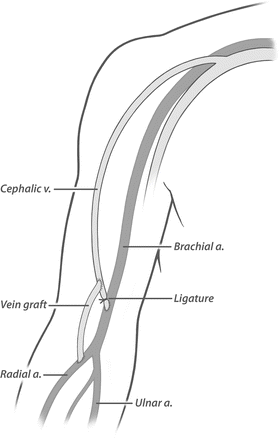
Fig. 19.8
Revision using distal inflow. The fistula is ligated and transected close to the brachial anastomosis. A short interposition bypass is then constructed from the more distal radial (or ulnar) artery
Ischemic Monomelic Neuropathy
In 1983 Wilbourn coined the term “ischemic monomelic neuropathy” (IMN) to describe an unusual form of ischemia affecting multiple nerves in the same extremity [58]. One of the three patients in his original paper developed IMN after an AV fistula and further experience has demonstrated that IMN is a rare but potentially devastating complication of vascular access with an incidence of 0.5 % [59]. As an unusual form of ischemia, IMN causes nerve damage often resulting in permanent limb dysfunction. The underlying pathology of IMN seems to involve mild, transient ischemia which triggers a disproportionately severe injury to susceptible peripheral nerves [58]. Most reports of IMN describe a diabetic patient who develops intense hand pain and weakness immediately following surgery to create a brachial artery-based AV access. Physical exam typically reveals a well-perfused hand with sensory and motor deficits of all three nerves (radial, ulnar, and median). When the diagnosis is unclear, noninvasive exams can provide valuable supplemental information. Most patients with IMN have digital pressures greater than 50 mmHg, while nerve conduction studies demonstrate reduced or unobtainable sensory and motor nerve action potentials [60]. Although these results support the diagnosis of IMN, noninvasive exams are not necessary in all cases and may serve only to delay treatment when the clinical scenario clearly points to IMN.
Immediate AV access ligation offers the only effective treatment for IMN. Failure to recognize and treat IMN leads to permanent nerve damage and a nonfunctional claw hand in severe cases [61]. Unfortunately, early diagnosis and immediate ligation often fails to completely reverse nerve dysfunction, and most patients require physical and occupational therapy to improve hand function [62]. IMN poses a risk to all patients undergoing AV access surgery making efforts at prevention difficult if not impossible. Although the presence of arterial insufficiency and diabetic neuropathy increases the risk, no preoperative finding or test can predict which patients will develop IMN [63]. Effective management of IMN requires a heightened awareness of its symptoms, early diagnosis in the immediate postoperative period, and prompt access ligation to minimize the long-term effects of the nerve injury.
Thrombosis
Vascular access thrombosis (VAT) is a disruptive and potentially dangerous event in the life of a dialysis patient. VAT interrupts dialysis which can be life-threatening in the setting of hyperkalemia or fluid overload. Salvaging the clotted access involves an invasive procedure with its associated risks, while failure to restore access patency puts the patient in the undesirable position of using a catheter for dialysis. Despite advances in surgery, dialysis surveillance, and endovascular tools, VAT causes more hospital admissions and more vascular access failures than any other complication [64]. Minimizing the negative impact of VAT requires an understanding of the pathophysiology and familiarity with surgical and endovascular thrombectomy techniques.
VAT results from a confluence of thrombotic factors described by Virchow’s triad: endothelial cell injury, reduction of blood flow, and hypercoagulability. The first part of the triad, endothelial dysfunction, reflects the underlying intimal hyperplasia which occurs in nearly all forms of vascular access. Intimal hyperplasia involves the proliferation of extracellular matrix, activated cells, and neovascularization which can narrow and ultimately occlude the vessel lumen. Several events commonly encountered in dialysis patients trigger intimal hyperplasia including surgical trauma, hemodynamic shear stress, AV graft bioincompatibility, needle puncture injury, and uremia. The second part of the triad, low blood flow or stasis, usually stems from a problem with access inflow, outflow, or the conduit itself. External compression and systemic hypotension can also compromise flow and predispose to VAT. Hypercoagulability, the third part of the triad, emerges when patients start receiving dialysis. In contrast to the bleeding tendency of uremic patients, patients on dialysis often exhibit hypercoagulability mediated primarily by activated platelets [65]. Exposure to prothrombotic surfaces (extracellular matrix, artificial membrane), coupled with turbulence in the AV access, activates platelets setting off a cascade of events which culminates in platelet deposition and conditions favoring intimal hyperplasia.
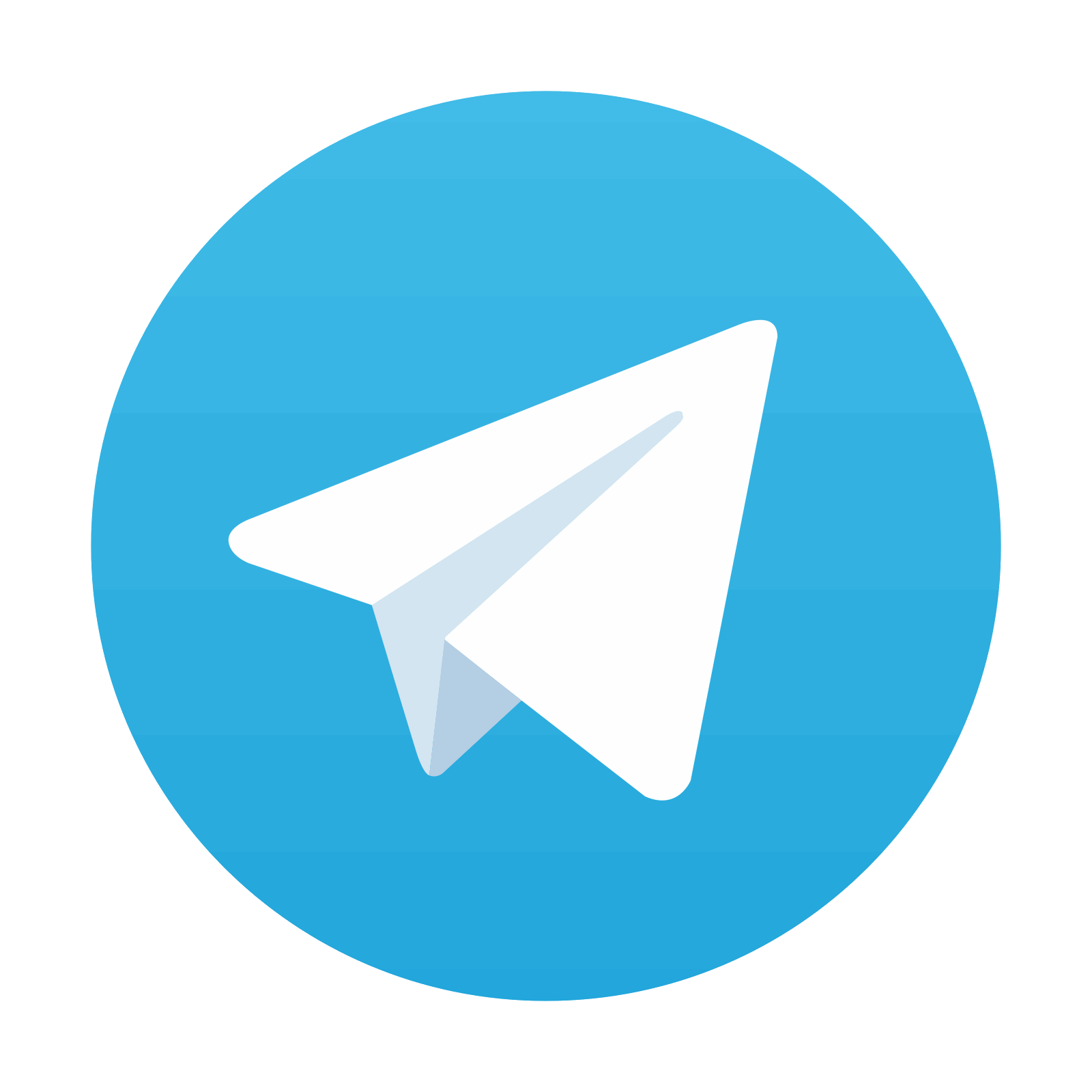
Stay updated, free articles. Join our Telegram channel

Full access? Get Clinical Tree
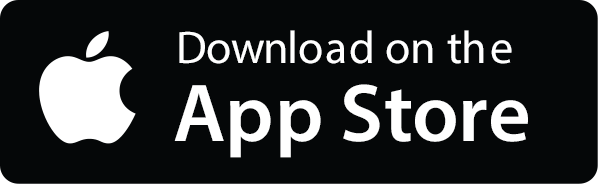
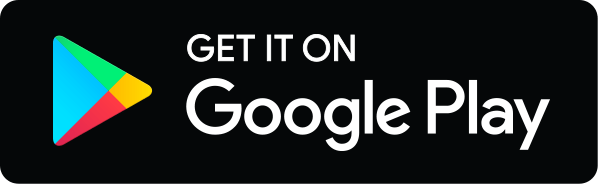