Abstract
Background/Purpose
In a number of patients with acute myocardial infarction (AMI), myocardial hypoperfusion, known as the no-reflow phenomenon, persists after primary percutaneous intervention (PPCI). The aim of this study was to evaluate the feasibility and safety of a new quantitative method of measuring absolute blood flow and resistance within the perfusion bed of an infarct-related artery. Furthermore, we sought to study no-reflow by correlating these measurements to the index of microvascular resistance (IMR) and the area at risk (AR) as determined by cardiac magnetic resonance imaging (CMR).
Methods
Measurements of absolute flow and myocardial resistance were performed in 20 patients with ST-segment elevation myocardial infarction (STEMI), first immediately following PPCI and then again after 3–5 days. These measurements used the technique of thermodilution during a continuous infusion of saline. Flow was expressed in ml/min per gram of tissue within the area at risk.
Results
The average time needed for measurement of absolute flow, resistance and IMR was 20 min, and all measurements could be performed without complication. A higher flow supplying the AR correlated with a lower IMR in the acute phase. Absolute flow increased from 3.14 to 3.68 ml/min/g (p = 0.25) and absolute resistance decreased from 1317 to 1099 dyne.sec.cm-5/g (p = 0.40) between the first day and fifth day after STEMI.
Conclusions
Measurement of absolute flow and microvascular resistance is safe and feasible in STEMI patients and may allow for a better understanding of microvascular (dys)function in the early phase of AMI.
Highlights
- •
We measured absolute coronary blood flow and microvascular resistance in STEMI patients in the acute phase and in the subacute phase, using the technique of thermodilution with low grade intracoronary continuous infusion of saline.
- •
These measurements are safe and feasible during PPCI in STEMI patients.
- •
In STEMI patients, absolute flow increases and resistance decreases in a considerable part of the patients during the first couple of days indicating recovery of microvascular dysfunction over time.
1
Introduction
In acute myocardial infarction, early restoration of blood flow to the jeopardized myocardium is of paramount importance to limit infarct size and obtain a favorable long-term outcome. Primary percutaneous coronary intervention (PPCI) is the treatment of choice for reestablishing epicardial blood flow in patients with ST-segment elevation myocardial infarction (STEMI) . Despite achievement of epicardial coronary artery reperfusion in approximately 90% of all patients, a number of these patients experiences persistent myocardial hypoperfusion due to moderate or severe microvascular dysfunction, also referred to as the “no-reflow” phenomenon . In 1974, Kloner et al. described this phenomenon in dogs and showed that, after the temporary occlusion of a coronary artery, subendocardial perfusion defects were detectable and persistent after 90 min of occlusion . Failure of reflow was associated with extensive capillary damage and myocardial cell swelling, leading to injury of the myocardial microvasculature.
The pathogenesis of no-reflow has not been elucidated completely and seems to be complex and multifactorial. Microvascular thromboembolism, spasm, intramyocardial edema and inflammatory responses of the myocardium with neutrophil plugging of the capillaries are all suggested to be responsible for this condition. It is well known that in patients in whom no or poor reflow occurs, prognosis is poor and more severe left ventricular dysfunction can be expected in comparison to those patients in whom microvascular reperfusion after PPCI is restored . Therefore, knowledge about the actual state of the microvasculature and myocardial perfusion shortly after PPCI is important from a prognostic point of view.
Moreover, if microvascular reperfusion is still limited immediately after myocardial infarction, but recovers quickly in the days thereafter, it might be beneficial for long-term prognosis . Lastly, knowledge about microvascular reperfusion in the acute phase might be important with respect to choice of adjunct mechanical or medical therapy, such as intra aortic balloon pumping (IABP), glycoprotein IIb/IIIa inhibitors or continuation of nitroglycerine.
Assessment of microvascular perfusion and function has so far been difficult and hampered by a number of methodological and technical shortcomings. Furthermore, measurement of absolute blood flow in the infarcted area and true quantitative calculation of absolute resistance in acute MI has never been performed.
Recently, a new invasive technique for measuring absolute coronary blood flow and absolute myocardial resistance has been developed for patients with stable coronary disease . This technique is based upon thermodilution using a continuous infusion of a low amount of saline through a microcatheter positioned selectively in a coronary artery. This technique is precise and not operator-dependent . Measuring absolute blood flow and resistance has no meaning by itself as long as the distribution territory is unknown. Also, it is difficult to compare flow and resistance among territories, not to mention among patients. However, if the area of interest is the dependent myocardial territory of the occluded coronary artery, this technique makes it possible to compare absolute flow (ml/min) in STEMI patients between the moment immediately after epicardial reperfusion by PPCI and the recovery phase a few days later, with potential implications for treatment. Because the sensor measuring absolute blood flow by continuous infusion methodology simultaneously measures distal coronary pressure, absolute minimal resistance can be calculated in dyne.sec.cm-5, as shown before in experimental studies and in humans with stable coronary artery disease . This quantitative measurement of flow and resistance is accurate and offers advantages over qualitative and indirect measures of resistance in STEMI, such as flow velocity-based indices or IMR .
Consequently, the purpose of the present study was to evaluate the feasibility and safety of absolute blood flow measurement and resistance in STEMI patients, both in the acute and subacute phases. Furthermore, we aimed to correlate this method of flow measurement to IMR, the existing, non-quantitative, invasive standard method for detecting microvascular dysfunction.
2
Material and methods
2.1
Patient selection and inclusion criteria
STEMI patients < 75 years of age admitted for PPCI with a total ST-segment deviation of more than 10 mm were eligible if they presented within 12 h of symptom onset and had a culprit lesion in the proximal or mid segment of a coronary artery whose reference diameter was ≥ 3.0 mm by visual estimation. Patients with previous myocardial infarction in the culprit area or with previous bypass surgery were excluded. Other exclusion criteria were cardiogenic shock, inability to obtain femoral access to the arterial circulation, tortuous coronary arteries, complex or long-lasting PPCI, inability to understand and give informed consent, severe concomitant disease or conditions with a life expectancy of less than one year, other known myocardial diseases such as cardiomyopathy or left ventricular hypertrophy, and pregnancy.
Oral informed consent was obtained by the interventional cardiologist immediately after successful PPCI. A more extensive written informed consent was obtained after the procedure, either in the catheterization laboratory or in the coronary care unit. Twenty patients were permitted to be included by the institutional review board of the Catharina Hospital Eindhoven.
2.2
PPCI Procedure
Patients received aspirin (300 mg) and a loading dose of clopidogrel, ticagrelor or prasugrel in the ambulance according to routine. Before angiography, all patients received heparin (5000 IU). Primary PCI was performed according to standard clinical practice. Use of thrombosuction or adjunctive pharmacology such as glycoprotein IIb/IIIa receptor inhibitors was left to the discretion of the operator. When, immediately following successful PPCI, the patient met the inclusion criteria and had given informed consent, measurements of distal coronary pressure and absolute coronary blood flow and resistance were performed as described below.
2.3
Invasive hemodynamic measurements
After successful PPCI of the culprit vessel, the guide wire was exchanged for one with distal pressure and temperature sensors (St Jude Medical, Minneapolis), whereafter distal coronary pressure, the index of microvascular resistance (IMR) and absolute flow were measured in the infarct-related artery as previously described in stable patients . Maximal hyperemia, and thereby minimal microvascular resistance, was induced by using 140 μg/kg/min of intravenous adenosine via a central venous catheter. Absolute minimal resistance was calculated by dividing distal pressure by the absolute flow and expressed in dyne.sec.cm-5 per gram of tissue in the area at risk.
For determination of IMR, small bolus injections of 3cm 3 of saline were given into the infarct-related artery as described by Fearon et al. . This was done three times at hyperemia. Mean transit time (Tmn) and Pd were measured simultaneously. IMR was calculated by multiplying the mean P d by the hyperaemic T mn . IMR was taken as the average of the three consecutive measurements at hyperemia as described before .
2.4
Measurement of absolute coronary blood flow
Thereafter, absolute flow was measured. A small specially designed infusion catheter with four side holes in its most distal part was advanced over the pressure guide wire through the Y-connector. The tip of the infusion catheter was placed in the stent, i.e. at the level of the previous occlusion, and the sensor was placed in the distal part of the infarct related artery. The infusion catheter was then connected to an infusion pump by a second Y connector, enabling continuous infusion of saline at a rate of 20–30 ml/min ( Fig. 1 ). The sensor-tipped guide wire was connected to the interface (Analyzer, St Jude Medical), and distal coronary pressure (P d ) and temperature were displayed simultaneously. The exact infusion rate was chosen depending on the size of the artery and at the discretion of the operator. Next, a continuous recording was made of P d and distal blood temperature after complete mixing during constant saline infusion ( Fig. 2 ). After a steady state had been achieved, the pressure/temperature sensor was pulled back into the infusion catheter so that the temperature of the saline (T i ) at the location of the most proximal side hole could be measured ( Fig. 2 ). Using the technology of continuous infusion of saline at a low rate, absolute hyperaemic blood flow (Q b ) can be calculated by the infusion rate of saline (Q i ), temperature of the infused saline (T i ) and distal blood temperature after complete mixing (T), using the following equation:
Q b = 1.08 T i T − 1 Q i + Q i ≈ 1.08 T i T Q i
The factor 1.08 corrects for the differences in specific heat between blood and saline. The protocol of absolute flow measurement was performed again at hyperemia after an interval of 5 min. The exact position of the sensor-tipped wire during measurements was documented on cine-angiography to make sure that the same position was chosen at the second exam 5 days later. All data were analyzed off line. Blood flow was expressed as ml/min and by adding the area at risk from CMR, blood flow per gram of tissue was calculated in ml/min/g for the area at risk.
After three to five days, control angiography of the infarct-related artery was performed followed by measurements of FFR, IMR and absolute flow using exactly the same position of the wire and infusion catheter as in the first procedure.
2.5
Cardiovascular magnetic resonance (CMR)
CMR was performed 3–5 days after the index procedure, preferably on the same day as the second invasive procedure. CMR was performed on either of two 1.5 T systems: Siemens or Magnetom Vision. Cine images were acquired on a 1.5 T scanner (Ingenia CV, Release 4.1.3.0 Philips Medical Systems, Best, The Netherlands) with electrocardiographic gating.
All subjects were placed in a supine position and short-axis, four-chamber, two-chamber and 3-chamber views were acquired during an end expiratory breath hold. Left ventricular function was assessed by a standard steady-state free precession technique (repetition time 3.3 ms, echo time 70 ms, flip angle 60 degrees, slice thickness 8 mm, slice gap 0 mm). An example of a mid LV short-axis image is shown in Fig. 3 .
For determination of the area at risk, we obtained short-axis slices covering the whole ventricle using a T2-weighted triple inversion recovery breath-hold pulse sequence (repetition time 2000 ms, echo time 70 ms, flip angle 90 degrees, slice thickness 8 mm, slice gap 0 mm).
All CMR images were analyzed using the freely available software Segment v 1.8 http://segment.heiberg.se (Heiberg et al. Bmc med imaging 10: 1, 2010).
The left ventricular (LV) mass was assessed on the cine images by manual tracing of the endocardial and epicardial borders with exclusion of the papillary muscles.
The AR was derived from the T2-weighted imaging and defined as the total amount of hyperintensive myocardium in all short-axis slices and expressed as a percentage of LV mass. All endocardial and epicardial tracings were performed by two observers blinded to the clinical data. They had to agree and provide the final percentage of the area at risk. Offline recalculation was done to obtain the AR and LV mass in grams.
2.6
Statistical analysis
Data are presented as mean ± standard deviation (SD), or median and interquartile range (IQR), as appropriate. Flow was expressed as ml/min/g. Resistance was expressed as dyne.sec.cm-5/g. IMR was expressed as dimensionless index (arbitrary units). Due to the maximal vasodilated state of the coronary circulation, a linear relationship was assumed to exist between perfusion pressure and blood flow . To compare the multiple measurements of flow over time within the same patient at different perfusion pressures, pressures were normalized to the initial aortic pressure (P a ) as proposed by Fearon et al. . IMR values at the initial measurement in the hyperacute phase of STEMI were divided in two groups, high and low, separated by the median value.
For evaluation of absolute flow and resistance over time within the same patient, each patient was his own control after normalization for aortic pressure. For comparison of flow and resistance between different patients, AR by CMR was used to calculate flow in ml/min/g of tissue, by dividing absolute flow and resistance by the mass of the AR. Differences between groups were assessed by the paired t-test for continuous data with a normal distribution. Otherwise, the nonparametric Wilcoxon rank-sum test was used.
2
Material and methods
2.1
Patient selection and inclusion criteria
STEMI patients < 75 years of age admitted for PPCI with a total ST-segment deviation of more than 10 mm were eligible if they presented within 12 h of symptom onset and had a culprit lesion in the proximal or mid segment of a coronary artery whose reference diameter was ≥ 3.0 mm by visual estimation. Patients with previous myocardial infarction in the culprit area or with previous bypass surgery were excluded. Other exclusion criteria were cardiogenic shock, inability to obtain femoral access to the arterial circulation, tortuous coronary arteries, complex or long-lasting PPCI, inability to understand and give informed consent, severe concomitant disease or conditions with a life expectancy of less than one year, other known myocardial diseases such as cardiomyopathy or left ventricular hypertrophy, and pregnancy.
Oral informed consent was obtained by the interventional cardiologist immediately after successful PPCI. A more extensive written informed consent was obtained after the procedure, either in the catheterization laboratory or in the coronary care unit. Twenty patients were permitted to be included by the institutional review board of the Catharina Hospital Eindhoven.
2.2
PPCI Procedure
Patients received aspirin (300 mg) and a loading dose of clopidogrel, ticagrelor or prasugrel in the ambulance according to routine. Before angiography, all patients received heparin (5000 IU). Primary PCI was performed according to standard clinical practice. Use of thrombosuction or adjunctive pharmacology such as glycoprotein IIb/IIIa receptor inhibitors was left to the discretion of the operator. When, immediately following successful PPCI, the patient met the inclusion criteria and had given informed consent, measurements of distal coronary pressure and absolute coronary blood flow and resistance were performed as described below.
2.3
Invasive hemodynamic measurements
After successful PPCI of the culprit vessel, the guide wire was exchanged for one with distal pressure and temperature sensors (St Jude Medical, Minneapolis), whereafter distal coronary pressure, the index of microvascular resistance (IMR) and absolute flow were measured in the infarct-related artery as previously described in stable patients . Maximal hyperemia, and thereby minimal microvascular resistance, was induced by using 140 μg/kg/min of intravenous adenosine via a central venous catheter. Absolute minimal resistance was calculated by dividing distal pressure by the absolute flow and expressed in dyne.sec.cm-5 per gram of tissue in the area at risk.
For determination of IMR, small bolus injections of 3cm 3 of saline were given into the infarct-related artery as described by Fearon et al. . This was done three times at hyperemia. Mean transit time (Tmn) and Pd were measured simultaneously. IMR was calculated by multiplying the mean P d by the hyperaemic T mn . IMR was taken as the average of the three consecutive measurements at hyperemia as described before .
2.4
Measurement of absolute coronary blood flow
Thereafter, absolute flow was measured. A small specially designed infusion catheter with four side holes in its most distal part was advanced over the pressure guide wire through the Y-connector. The tip of the infusion catheter was placed in the stent, i.e. at the level of the previous occlusion, and the sensor was placed in the distal part of the infarct related artery. The infusion catheter was then connected to an infusion pump by a second Y connector, enabling continuous infusion of saline at a rate of 20–30 ml/min ( Fig. 1 ). The sensor-tipped guide wire was connected to the interface (Analyzer, St Jude Medical), and distal coronary pressure (P d ) and temperature were displayed simultaneously. The exact infusion rate was chosen depending on the size of the artery and at the discretion of the operator. Next, a continuous recording was made of P d and distal blood temperature after complete mixing during constant saline infusion ( Fig. 2 ). After a steady state had been achieved, the pressure/temperature sensor was pulled back into the infusion catheter so that the temperature of the saline (T i ) at the location of the most proximal side hole could be measured ( Fig. 2 ). Using the technology of continuous infusion of saline at a low rate, absolute hyperaemic blood flow (Q b ) can be calculated by the infusion rate of saline (Q i ), temperature of the infused saline (T i ) and distal blood temperature after complete mixing (T), using the following equation:
Q b = 1.08 T i T − 1 Q i + Q i ≈ 1.08 T i T Q i
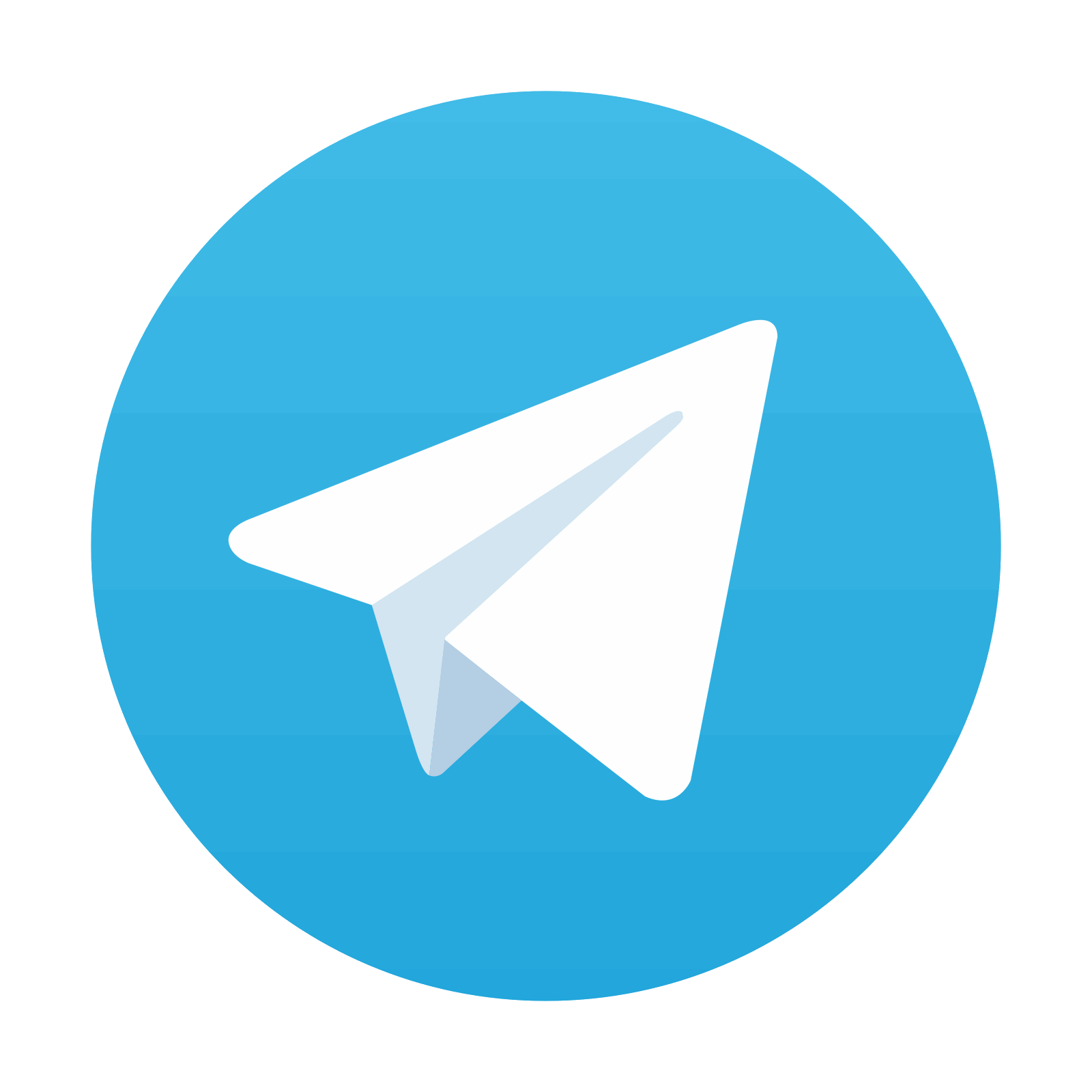
Stay updated, free articles. Join our Telegram channel

Full access? Get Clinical Tree
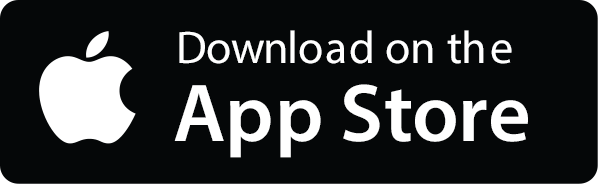
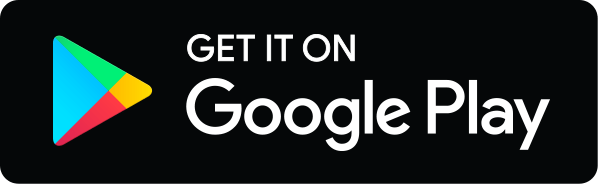