, Germaine Cornelissen2 and Franz Halberg2
(1)
Department of Chronomics & Gerontology, Tokyo Women’s Medical University Medical Center East, Arakawa-ku, Tokyo, Japan
(2)
Halberg Chronobiology Center, University of Minnesota, Minneapolis, MN, USA
Abstract
The results for SCD in Minnesota resemble those in Tokyo. They are also in keeping with an about-1.3-year component detected in a 10-year long record of atrial fibrillations, potentially fatal arrhythmia that may underlie SCD. These results imply the possible influence of nonphotic as well as of photic solar effects, at least in some geographic locations.
This possibility is in keeping with the finding of an about-10.5-year cycle characterizing the incidence of MI in Minnesota (1968–1996), accounting for a 5 % difference in mortality between years of maximal vs. minimal solar activity. The fact that nonphotic (e.g., magnetic) effects are latitude dependent may account in part for geographic differences observed in the patterns of incidence of SCD. Nonphotic influences may also stem from several sources, such as cosmic rays, solar flares, the solar wind, the interplanetary magnetic field, and/or geomagnetic disturbances as measured on earth, to name but a few.
For SCD, high geomagnetic activity is associated with a decreased incidence of SCD. For SCD, underlying factors may be explored in pertinent markers such as the incidence of potentially lethal arrhythmia recorded in some pacemaker-cardioverter-defibrillators, monitored longitudinally for the individual patient, before an untoward event occurs.
Keywords
Sudden cardiac deathCircaseptan componentTransyearsNear-transyearsGeomagnetics15.1 Introduction
This study aimed at reexamining, in different geographic/geomagnetic locations, the time patterns of SCD, in the strict sense, as a refined diagnosis according to the International Classification of Diseases, revision 10 (ICD10, code I46.1). Thereby, we wish to assess the relative prominence, gauged by a nonzero amplitude (A) of different spectral components (classified by the 95 % CI of their period not overlapping a period between 0.8- and 2.0-year length). Thus, we aim at exploring the relative importance of photic vs. nonphotic influences on SCD or, more specifically, of the seasons vs. unseen cosmic factors for which magnetic cycles may be a proxy, if not a cause, or both.
By 1991, it was apparent that the least-squares spectrum of SCD (as classified at the time, including MIs) already differed from that of MIs, strokes, cardiac arrhythmia, hypertensive episodes, and asthma [1, 2]. “SCD,” in quotation marks (including presumably MIs), had a somewhat prominent circadiseptan component [2–4] (also shown in Fig. 15.1), in addition to a very prominent about-yearly (Fig. 15.1) and a lesser about-weekly (Fig. 15.2) component, which latter it shared with other conditions. A circadiseptan component of about 15.2 days may be interpreted as reflecting the influence at a given time and/or through evolution of a very wobbly magnetic cycle in the environment (see Fig. 15.1), e.g., in a global geomagnetic index, Kp, or a local such index, K [4]; cf. [5, 6], although the influence of factors of lifestyle, such as alcohol consumption on paydays, cannot be ruled out (Fig. 15.1) [3, 4].



Fig. 15.1
Wobbliness of the about-2-week variation in “sudden cardiac deaths” (top) and the local index, K, of geomagnetic activity (bottom), revealed by gliding spectra wherein data in a 6-month interval are progressively displaced by a 1.5-week increment. Note that detection of the about-2-week component is not consistent throughout the 3-year span. A possible resonance with occasional frequency trapping between the multiseptans of the local geomagnetic activity index, K, and “sudden cardiac death” is suggested by the more prominently expressed (darker) about-half-monthly variation in “sudden cardiac deaths” observed when this component is also detected in the spectrum of K [3, 4] (© Halberg Chronobiology Center)

Fig. 15.2
(a) Incidence of sudden cardiac death, in the strict sense according to the International Classification of Diseases, 10th revision (ICD10), code I46.1, in Minnesota. The naked eye has difficulty in discerning a pattern, yet some peaks occur apparently at intervals longer than 1 year (© Halberg Chronobiology Center). (b) The fit of a 1.39-year cosine curve, a period resolved by linear-nonlinear rhythmometry, to the data from Fig. 1a stacked for this transyear allows Fig. 15.2 (continued) the rejection of the zero-amplitude assumption below the 2 % level of statistical significance (© Halberg Chronobiology Center). (c) Stacking of the data in Fig. 15.2a for a calendar year does not reveal any statistical significance in a zero-amplitude (no-rhythm) test. This would be expected if only a transyear (Fig. 15.2b), but no calendar-yearly component characterized the data (© Halberg Chronobiology Center)
Our finding of a near week of 6.75 days [1, 4, 6] rather than a precise week [7] [8]; cf. [4, 6] in geomagnetics, first in the planetary index, Kp [1, 4, 6], was confirmed [9] and extended to the antipodal index aa [10] by physicists and then by ourselves [6], all as a counterpart of a built-in biological week [11]; cf. [12–15] This led us to the postulate that the rotation of the earth around its axis is not the only environmental cycle built into life on earth. Not even the extension of the time structure to include the time it takes for the earth to revolve once around the sun and the consequent seasonal changes with photic effects exhaust the list of putative built-in cycles. The finding of the near week in our environment prompted us to seek an environmental counterpart for each biological cycle, preferably one anchored in the genome, as suggested for the week by free-running [11]. Vice versa, we began to seek a biological counterpart for each more or less consistent, albeit wobbly environmental cycle, characterizing nonphotic as well as photic solar activity [1, 16–18]. Accordingly, in biology, half-yearly and about-decadal, about-didecadal, about-quindecadal, and even about-quincentennial cycles were found [18–20], the latter by Pales and Mikulecky [20].
With the discovery by the physicists John Richardson et al. [21] of a 1.3-year oscillation in satellite-measured changes of the speed of particles passing by, dubbed the solar wind, the follow-ups by Valdes-Galicia et al. [22] of a 1.68 year in cosmic rays [22] and by Rangarajan and Muniz Barreto [23], and the report of a 1.5- to 1.7-year oscillation by Mursula and Zieger [24], it seemed logical to look for biological counterparts for such years longer than a year – we called them “trans [= beyond] a year’s length,” briefly “transyears.” Indeed, we found them, to start with in each of several dozen longitudinal time series of human blood pressure and heart rate [25–27]. We defined transyears as spectral components with a period and its 95 % confidence interval (CI) between 1.0 and 2.0 years, and cisyears as spectral components with a period between 0.5 and 1.0 year and added the condition that the 95 % CIs of the periods must not overlap the specified limits. Eventually, we also found in the solar wind and geomagnetics a period of about 1.05 year [28] and the same period in biology: in human 17-ketosteroid excretion [29], in epidemiological data [28], and even in the oxygen production by a giant eukaryotic unicell Acetabularia [19]. We then separated near-transyears from far-transyears and near-cisyears from far-cisyears, in keeping with the very tentative Scheme 15.1, that, as in the circadian case [27], leads to limits specified in terms of period rather than in frequency.


Scheme 15.1
Nomenclature of cycles with periods between 0.4 and 2.0 years
Against this background, it became of interest to reexamine the time structure of the region of periods between 0.3 and 2.0 years, in order to compare the prominence of any spectral component corresponding, with its period, to the length of a calendar year vs. that of any cis- or transyears. Since the ICD10 eliminated death from MI and from unknown and unspecified causes from its code I46.1, it was of interest to focus mainly upon the years available with this code in the analyses presented herein, mostly for the span from 1999 to 2003. A longer series was available to check on the assumptions, supported by other data [25], that 95 % CIs overlapping 2.0 years were probably due to the brevity of the series analyzed and that the 95 % CIs of the periods will become tighter with increasing series length, as had been the case for the transannual and for the decadal components in blood pressure and heart rate series.
15.2 Epidemiology, Materials, and Methods
Data were first obtained from the Minnesota Department of Health through the courtesy of Ms. Judy Palermo. When a period corresponding to a far-transyear was readily apparent in these data, since the 95 % CI overlapped neither the 1.0- nor the 2.0-year period, as seen in Table 15.1, and no component with the length of a calendar year was noted, this was interpreted as a putative signature of a magnetic cycle corresponding in length to cycles described in the solar wind by Richardson et al. [21] and Mursula and Zieger [24]. This assumption was in keeping with the rule of “reciprocity of cycles in and around us” made for investigation of The Biosphere and the Cosmos, BIOCOS [15]. Added data were then solicited from Arkansas; North Carolina; the Czech Republic; Tbilisi, Republic of Georgia; and Hong Kong. The data covered all or part of the span from 1999 to 2003, with a longer SCD series also added from the Czech Republic, as were Czech and Minnesotan data on MI. Linear-nonlinear rhythmometry was used on each series [30–34]. We also looked for product-moment correlations among the epidemiological series themselves and between each of these series and the planetary geomagnetic disturbance index Kp. Superposed epochs in relation to events defined by a Kp >6 were also applied. As yet, the correlation coefficients were small and the superposed epochs showed a minimum at Kp >6, but minima were also found at timepoints distant from the Kp >6 and hence are not shown herein.
Table 15.1
Geomagnetic/geographic differences among cycles with periods in the range of 0.8–2.0 years, characterizing the incidence of sudden cardiac death (SCD)a,b and myocardial infarction (MI)
Site | Span | T, Δt, N | SCD (N) | Period (y) | (95%CI) | Amplitude | (95%CI) | A(% MESOR) | P-valuec |
————— Transyear (TY) or Candidate Transyear (cTY) Detected ————— | |||||||||
Minnesota | 1999–2003 | 5y, 1d, 1826 | 343 | 1.392 (TY) | (1.173, 1.611) | 0.042 | (0.00, 0.09) | 22.0 | 0.014 |
Arkansas | 1999–2003 | 5y, 1d, 1826 | 273 | 1.095 | (0.939, 1.251) | 0.032 | (0.00, 0.07) | 21.1 | 0.040 |
1.686 (cTY) | (1.293, 2.071) | 0.031 | (0.00, 0.07) | 20.7 | 0.044 | ||||
Czech Rep. | 1999–2003 | 5y, 1d, 1826 | 1006 | 0.974 | (0.856, 1.091) | 0.078 | (0.00, 0.16) | 14.2 | 0.007 |
1.759 (cTY) | (1.408, 2.110) | 0.077 | (0.00, 0.15) | 13.9 | 0.010 | ||||
1994–2003 | 10y, 1d, 3652 | 1792 | 1.726 (TY) | (1.605, 1.848) | 0.074 | (0.02, 0.13) | 15.1 | <0.001 | |
1.000 | (0.944, 1.056) | 0.052 | (0.00, 0.10) | 10.6 | 0.010 | ||||
———————– Candidate Transyear Not Detected ———————– | |||||||||
North Carolina | 1999–2003 | 5y, 1d, 1826 | 752 | 0.929 | (0.834, 1.023) | 0.069 | (0.00, 0.14) | 16.9 | 0.007 |
Tbillsi, Georgia | Nov99–2003 | 4.1y, 1d, 1505 | 130 | 0.988 | (0.862, 1.114) | 0.035 | (0.00, 0.07) | 40.7 | 0.007 |
Hong Kong | 2001–2003 | 3y, 1m, 36 | 52 | 0.843 | (0.651, 1.036) | 0.022 | (NS) | 44.9 | 0.077 |
Myocardial Infarction (MI) | |||||||||
Site | Span | T, Δt, N | MI (N) | Period (y) | (95%CI) | Amplitude | (95%CI) | A(% MESOR) | P-value2 |
————— Coexisting Year (Circannual) and Transyear (TY) ————— | |||||||||
Czech Rep. | 1999–2003 | 5y, 1d, 1826 | 52598 | 1.014 | (0.989, 1.038) | 2.85 | (2.22, 3.48) | 9.88 | <0.001 |
1.354 (TY) | (1.252, 1.456) | 1.35 | (0.69, 2.02) | 4.68 | <0.001 | ||||
1994–2003 | 10y, 1d, 3652 | 115520 | 0.998 | (0.988, 1.009) | 3.03 | (2.47, 3.60) | 9.58 | <0.001 | |
1.453 (TY) | (1.417, 1.489) | 1.91 | (1.34, 2.49) | 6.04 | <0.001 | ||||
1.15 (TY) | (1.116, 1.184) | 1.23 | (0.64, 1.82) | 3.88 | <0.001 |
15.3 Transyear Rhythms in SCD and Mortality from MI
Table 15.1 shows that under sudden cardiac death in 3 of the 6 geographic/geomagnetic regions examined, there was only a spectral component of a calendar year in length. In these locations (North Carolina, Tbilisi, and Hong Kong), the point estimate of the period may differ slightly numerically from the exact year’s length, but the 95 % CI in each case covers the precise calendar-year length. By contrast, in the data from Minnesota, there is only a transyearly component. Figure 15.2a shows the actual data: it is difficult to interpret these very low monthly incidences without analyses. Nonetheless, some peaks are easy to see and are more than a year distant from each other and are roughly in keeping with the period given in Table 15.1. Stacking of the data over this period in Fig. 15.2b results in a zero-amplitude test significant below the 2 % probability level. By contrast, as would be expected if a calendar-yearly component were absent, the fit of a 1-year cosine curve to data stacked along the calendar year in Fig. 15.2c does not allow the rejection of the zero circannual amplitude assumption (P = 0.171).
In the data from Arkansas and the Czech Republic, there are components with the length of a calendar year, along with a probable candidate transyear. We speak of a candidate transyear when the period is between 1.0- and 2.0-year length and does not overlap 1 year, but overlaps the length of 2 years. In other words, in the 1999–2003 series, the condition of the 95 % CI of the period not overlapping 2 years is not (yet?) met by transyears in Arkansas and the Czech Republic in the data limited to 5 years, but it is met for the longer series from 1994 to 2003 from the Czech Republic. As noted, it seems likely from Table 15.1 and from individual series of blood pressure and heart rate measurements over decades (that are continuing) that the longer the series, the more it may be characterized by a transyearly component. Indeed the longer SCD series from the Czech Republic reveals a clear far-transyear, with no overlap of 2.0 years (an overlap is found with the shorter series from the same area). Mortality from MI, also included in Table 15.1, shows in turn during 1999–2003 a very prominent circannual component and a much less prominent transannual. In the longer series on MI, also summarized in Table 15.1, a near-transyear and a far-transyear (see Scheme 15.1) are both resolved.
15.4 Biomedical Background
The variability of a large data set on diagnoses made in the context of calls for an ambulance in Moscow, including 71,525 “SCDs” (that included some MIs) during a span of intensive solar activity from 1979 to 1981, had prompted us to look into any effects of a turn in the north–south vector, Bz, of the interplanetary magnetic field, as a measure of a magnetic storm [1]. There has been a long controversy about the merits or demerits of a “heliobiology.” Its importance was very strongly supported in the Soviet Union and subsequently again fully accepted and highly regarded as a scientific area sui generis in present-day Russia [35–45]. The effect of geomagnetic activity as such or as a proxy for solar effects upon human mortality from MI and other cardiovascular conditions is in keeping further with a summary of mortality from different causes in Europe in the 1930s [46–48] and in keeping with the continuing contributions of Stoupel [49, 50], Ertel [51, 52], Mikulecky [14, 53–55], Strestik and Prigancova [56], the Russian team leader Breus [57] with Villoresi et al. [58, 59], and ourselves [1, 15, 57, 60]. Superposed epochs revealed an effect of the magnetic storm [1]. A mechanism possibly underlying these associations was found by self-studies [60] in Fig. 15.3a, b and found in the laboratory as well [42, 61]. The strongest support came from an approach by subtraction and addition, in an individual or group [62, 63].


Fig. 15.3
(a) Reduced heart rate variability of a clinically healthy 48-year-old man (KO) on days of high magnetic activity vs. days of low magnetic activity, assessed longitudinally in beat-to-beat data recorded around the clock over a 7-day span and analyzed over consecutive 14.4-min intervals. Heart rate variability is gauged by two related endpoints: the coefficient of variation of RR intervals (time domain; left) and the total spectral power (frequency domain; right). The abnormal record prompted an inquiry by one of us (GC) into possible causes. The response by KO was first that nothing was unusual until the record of geomagnetics became available and a magnetic storm was found during the recording span (© Halberg Chronobiology Center). (b) During the span examined in Fig. 15.3a, during days of high magnetic activity, heart rate variability (HRV), in different spectral regions of a clinically healthy 48-year-old man (KO) on days of high magnetic activity vs. days of low magnetic activity, assessed longitudinally, is reduced (left) to different extents or not at all (right): the decrease in HRV is more pronounced for periods in the spectral region between 6.5 and 333 sec than in other spectral regions. This result has been confirmed on additional cases; it may point to some underlying physiological mechanism responsible for the physiological response to changes in magnetic activity other than the parasympathetic, usually identified with spectral power in the 3.5-sec region. *References to “high” and “low” frequency are misnomers once it is realized that HRV changes, with the ~10.5-year solar cycle, a frequency much lower than a very low frequency: an “infra VLF” [56, 57] (© Halberg Chronobiology Center)
Geographic differences may underlie the failure of very thorough investigations in the USA by Feinleib et al. [64] and Lipa et al. [65] to confirm earlier findings, notably in Russian and German studies, and studies in Israel and Lithuania, led with proper documentation by Stoupel. Much more on the effect of magnetic storms or cycles upon human morphology, physiology and pathology have been the subject of nine conferences [19, 66, 67].
A special section of Table 15.1 reveals further that both yearly (or circannual) and transyearly components coexist in MI, but in that case, the transyear is substantially smaller in amplitude than the calendar-yearly component. It is the more remarkable that during the span investigated for the Minnesota SCDs, in the strict sense, there is only a transyearly and no calendar-yearly component detected, notably since for a much longer 29-yearly Minnesota series of MI, the seasonal component was much more prominent than an also-present signature of a nonphotic, probably magnetic circadecadal component.
15.5 Caveats
Two thousand years ago, Hippocrates reportedly associated thoracic pain with sudden cardiac death [68]. In 1707 and again in 1718, Giovanni Maria Lancisi [69, 70]; cf. [71] published a scholarly, all-encompassing monograph on sudden death; and in 1917, Heinrich Ewald Hering published a book on “death within seconds, with special reference to fibrillation” [72]. The topic remains of interest as an entity in its own right, although the suddenness of the death is differently described by different authors, with the International Classification of Diseases, 10th revision, now referring to death within up to 1 hour from the start of symptoms to the terminal event [73–75]. The forensic pathologist, however, in the case of an unwitnessed death, may use the definition of sudden death for a person known to be alive and functioning 24 hours earlier, and this is acceptable to scholars in the field with proper qualification. “To the best of my knowledge” is accordingly included in the wording of death certificates. It seems reasonable in the future to focus on a subsample of cases (e.g., with cardioverter-defibrillators and/or other monitors) whose premortal records may be studied for antecedents, even when death was not witnessed.
With such qualifications, it must also be noted that the relative brevity of the series in Table 15.1 is a serious limitation, as is the restriction of analyses to only six sites. Almost certainly, as seen in many other instances, with an increase in length of a given series, a spectrum can be very greatly changed, and we must not be speculating herein. For physics, Mursula and Zieger [24] proposed that an about-1.3-year component changes to an about-1.5- to about-1.7-year oscillation with each subsequent solar cycle. In pursuing this possibility, a further analysis of a 131-year series of the geomagnetic aa index then revealed the near-transyear as well, and as in the case of Mursula and Zieger [24], these components were intermittent; our analyses in sections of fixed rather than solar cycle lengths do not suffice to rule in a relation to changes in the solar bipolarity [6, 25].
The data in Table 15.1, however, suffice to point to the need for further scrutiny of any regional differences, even within the same country (USA), where three different situations are found with North Carolina having only an about-yearly component; Arkansas having both components, the year and a candidate transyear, with nearly equal amplitude; and Minnesota having just a transyear in the limited data available.
Geographic/geomagnetic differences have also been documented for the circadidecadal phase of human neonatal body weight, with a near antiphase between 2,150,122 newborns in Minnesota and 1,166,206 newborns in Denmark [76]. Whether local geo- or atmosphero-magnetics and/or other factors account for this difference and others, including those in Table 15.1, remains an open question. It seems reasonable to suggest that one may withhold inferences in any aspects of heliobiology until multiple locations are scrutinized for as long a span as possible, for a given pattern; one must also define the length of the time series examined, underlying a given finding so that it can be further qualified when added longer time series become available. In the context of broader evidence, it seems reasonable to postulate that unseen nonphotic cycles that have their origin in the solar wind, for which geomagnetics are a proxy if not cause, contribute substantially but differently to the pattern of human sudden death and to body weight at birth and that this contribution is also dependent on local factors, possibly terrestrial and atmospheric electrical currents. The role of nonphotic cycles in transdisciplinary human affairs is a challenge, for which an atlas in space and time constitutes an indispensable requirement [18], as agreed upon in a series of international consensus meetings [19].
The task of providing the data is the opportunity of national governments and international agencies in a historical context. We have shown cycles of about-50-year length, including one in stroke incidence with similar periods in the Czech Republic and in Minnesota, USA, and supporting data from Sweden, Slovakia, and Arkansas, USA [15, 67, 77]. Until governments and international agencies act, action by ministers of health like Bohumil Fiser [77] and/or responsible statisticians served us herein. Letters to US state health departments other than those of Minnesota, Arkansas, and South Carolina have remained unanswered for weeks and will remain so perhaps indefinitely. Government statisticians will have to realize that they are not only bookkeepers; their opportunities, if not mandates, should include the cost-free provision of dense (when indicated, preferably hourly) data as a minimum and the chronomic time series analysis by themselves of the accumulating records as an optimum. Health care can take stock of cycles which may differently affect MI and SCD in different geographic locations and to a different extent and in a different way in different variables [78]. Much added international research against this background could focus on many aspects of time structure, complementing focus on time of day [79–81] or rather circadian phase [82, 83]; cf. [84, 85], day of week or season [81], or rather circaseptan [2] and circannual phase [2, 82, 86, 87], cycles adding to the heart’s rhythm [88] a broader historical context.
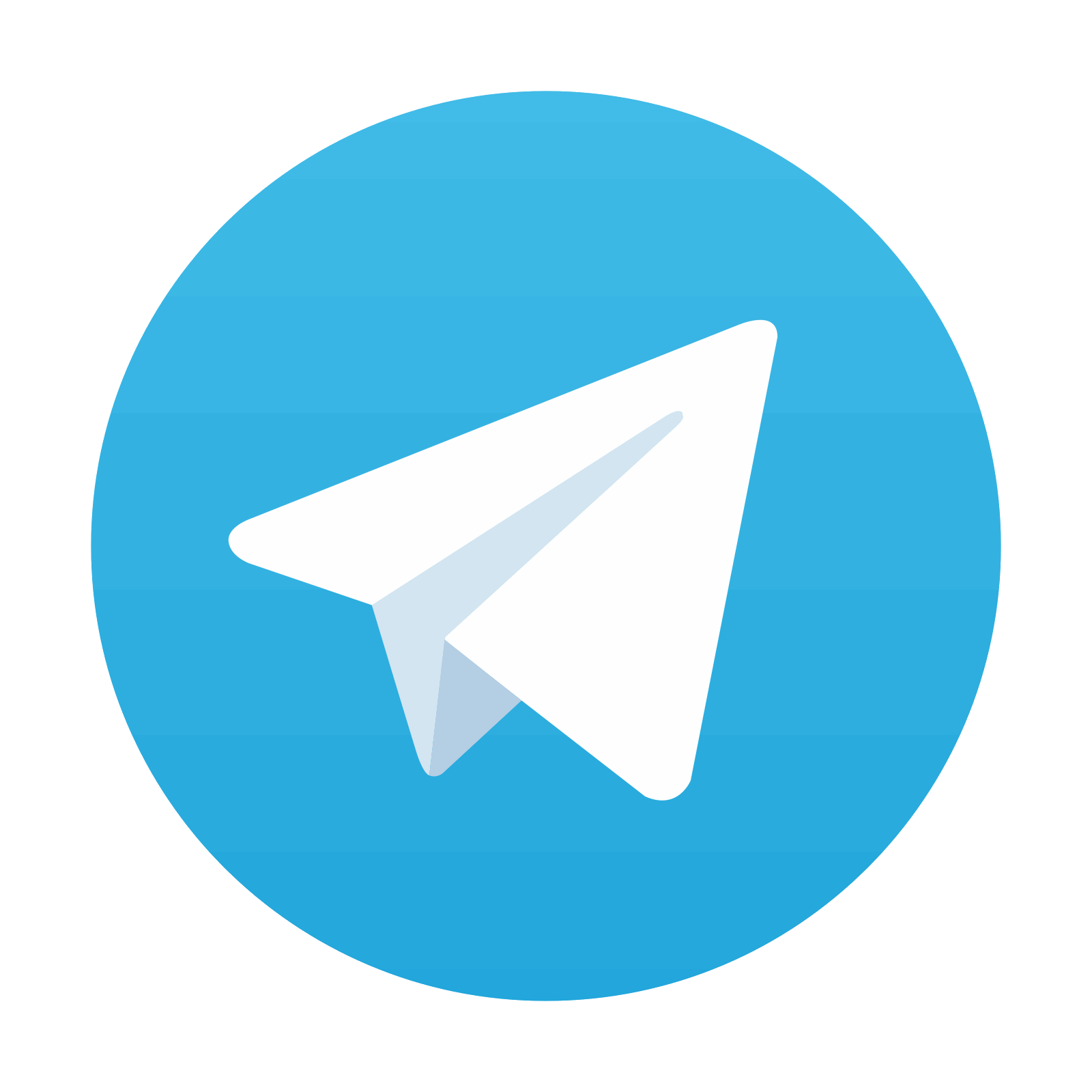
Stay updated, free articles. Join our Telegram channel

Full access? Get Clinical Tree
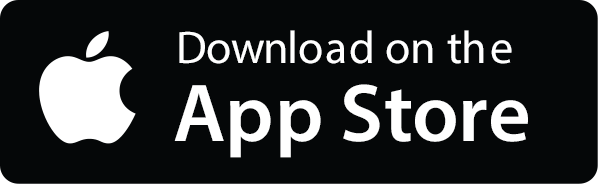
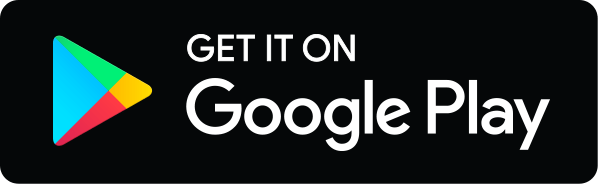