Introduction
The leadership at the American Society of Echocardiography (ASE) decided on a proactive role in defining selected areas of research necessary in this decade that will meet our future clinical needs. Consequently, ASE sponsored a Technology and Research Summit in the fall of 2010 in conjunction with the American Heart Association Scientific Sessions in Chicago. In addition to the ASE executive committee, in attendance were the editor, deputy editor, and one of the associate editors of the Journal of the American Society of Echocardiography . Also invited were physician-scientists active in the field of cardiovascular ultrasound, respected ultrasound physicists, and senior engineers from the various ultrasound companies.
The agenda for the full-day meeting covered a selected range of subjects including the assessment of global and regional left ventricular function, regional myocardial perfusion, molecular imaging, therapeutic ultrasound, and peripheral vascular imaging. Also addressed were research necessary to determine the broad clinical utility of hand held ultrasound devices and the impact of future technological developments on the field of cardiovascular imaging.
Because of time constraints, other important and worthy areas of research were not discussed. There was an hour devoted to the discussion of each subject that was initiated by the chairs and panelists assigned to each of the topics. The discussion was robust, and at the end, the chairs and panelists for each topic were requested to submit in writing a short synopsis of the discussion. These have been compiled into a document that we believe will serve as a roadmap for cardiovascular ultrasound research for this decade. At the end of each section a short list of references for selected reading is provided.
Although we have defined the areas that are ripe for future research, we also strongly believe that we have to train the future scientists who will implement this research agenda. ASE has historically awarded one or two fellowship training grants a year and also an award for research training of a sonographer. At some institutions fellows have also received training grants from the local American Heart Association, and very occasionally a training grant (F32) from the National Institutes of Health. However, this is not enough. We need more institutional training grants from the National Institutes of Health in order to train an adequate number of MD and PhD scientists in cardiovascular imaging. To our knowledge there are currently only a handful of such training grants in the country, which is woefully inadequate. We believe that we need at least 20–25 such training grants devoted to the general field of cardiovascular imaging so that within a decade there will be enough physicians trained in scientific methods and clinical research to address the subjects that have been discussed in this report.
The field of cardiovascular ultrasound is very broad, ranging from clinical validation of new technology to studies requiring knowledge of physics, mathematics, organic chemistry, physiology, pharmacology, molecular and vascular biology, genetics, clinical trials, and outcome research. Cross-training of individuals in one or more of these fields is essential for cardiovascular ultrasound to thrive and succeed. Our hope is that this report will encourage young people to realize the scope of cardiac ultrasound research and make a career in this dynamic field.
Selected Reading
- 1
Dorsey ER, Thompson JP, Carrasco M, de Roulet J, Vitticore P, Nicholson S, Johnston et al. Financing of US biomedical research and new drug approvals across therapeutic areas. PLoS ONE 2009;4:e7015.
Assessment of Global and Regional Left Ventricular Function
Global and regional function assessments are key to the clinical management of patients with cardiovascular diseases. Currently, these are usually performed qualitatively because most current quantitative methods for assessing them are not robust and are not automated. Recently, however, new methods have been introduced for quantifying cardiac function from estimates of myocardial deformation (myocardial strain and strain rate) and torsion (spatial variation of cardiac rotation) based on analyses of ultrasonic myocardial speckle patterns over the cardiac cycle. While these methods show great promise for assessing alterations in global and segmental myocardial performance, they currently exhibit a wide variation in the reported values with a significant dependence on the imaging system used as well as the image quality. Hence, there is a need to standardize the measurements among the echocardiographic systems employed. Additionally, for these measurements to be clinically useful, their precision, accuracy, and reproducibility must be significantly improved. Furthermore, the current standards are not optimized to permit the efficient storage and transfer of three-dimensional (3D) echocardiographic cineloops and related information.
The formation of echocardiographic images and associated measurements based on analyses of speckle pattern data depend upon the processing and detailed characterization of received backscattered ultrasonic signals from the myocardium, which are dependent upon the intrinsic ultrasonic scattering and attenuation properties of the myocardium, the frequency-dependent attenuation properties of overlying tissues (e.g., chest wall), and the specific features of individual imaging systems. The specific characteristics of each type of imaging system have a profound impact on the detailed nature of the received backscattered signals and subsequent speckle patterns observed and include transmit and receive beam profiles, line density, frequency bandwidths, and pulse lengths. Because each manufacturer implements uniquely different choices of system-dependent characteristics, the received signals and features of resultant speckle patterns can give rise to system-dependent variations in measured values. In addition, manufacturers often choose to measure and report slightly different realizations of myocardial strain parameters. This makes the data acquired and analyzed using different echocardiographic imaging systems difficult to compare.
In addition to the technical issues associated with the nature of backscattered signals and characteristics of echocardiographic imaging systems that can have a significant impact on measurements, the effects of image acquisition and user analysis (e.g., the placement of the region-of-interest) also impact the variability of measured values. Although it is generally accepted that poor or non-standard echocardiographic windows can affect results of measurements, the degree of this effect is unknown. The effect of region-of-interest placement on measurement variability has not been systematically investigated. It is expected that precision will be adversely impacted as regions-of-interest grow smaller.
Utilization of myocardial deformation and torsion measurements will require knowledge of the range of normal values. The mean values and associated variations of these parameters for specific cohorts of normal subjects need to be firmly established before routine clinical adoption of myocardial deformation and torsion measurements can be implemented. Variability can take several forms. The easiest to achieve consistency should be with the same cardiac cycle data being analyzed by the same software. To the extent that this is fully automated, the same results should be achieved each time. If there is manual intervention, there may be variability based on precise placement of regions-of-interest, but such variability should be small. Analysis of the same acquisition by a second software package might also be considered; this may yield either random or systematic deviation from the first result depending on whether the two packages use the same mathematical algorithm to estimate strain. Even this simple test is often impossible to do, since most of the vendors store their data in proprietary scan line format, which can only be analyzed by their own software package. Additionally, analysis of successive beats within the same study should be assessed, using either the same software package or a different one. Finally, the ultimate test of variability is the test-retest assessment whereby the patient is scanned twice (with either the same or, more relevantly, another scanner), and the data are analyzed independently.
Standard methods for characterizing images and measurements obtained with ultrasonic imaging systems are available from a number of sources including the International Electrotechnical Commission, the American Institute of Ultrasound in Medicine, and the American Association of Physicists in Medicine. These approaches typically utilize images of commercially available tissue-mimicking phantoms and are largely designed to assess properties such as spatial and contrast resolution, sensitivity and dynamic range, and geometric measurement accuracy on static images. In addition, there are somewhat more limited approaches described for characterizing Doppler measurements using special “flow” phantoms. However, to date, there are no standard methods or commercially available tissue-mimicking phantoms designed to assess echocardiographic-specific measurements of myocardial deformation and rotation. The only such standards to date have been short lifetime models, ones utilizing ex-vivo porcine hearts with implanted sonomicrometry crystals and gel-based models that can be compressed, but unfortunately degrade within days. The development of standard characterization methods and specialized, well-characterized phantoms would provide an objective approach to estimate the limiting uncertainties of these echocardiographic image based measurements.
Image data archiving standards and formats are not currently optimized to handle all the types and large amounts of data associated with volumetric echocardiographic imaging and advanced image analysis features. For example, the current Digital Imaging and Communications in Medicine (DICOM) standard for two-dimensional (2D) echocardiographic images only accepts pixels in a Cartesian, raster-based orientation. Unfortunately, speckle tracking for most of the major vendors is performed on proprietary formats based on individual scan-lines. Full interoperability in speckle tracking will require collaboration to harmonize formats. There are three possible approaches to this: (1) the DICOM committee (and appropriate companies) could agree upon a single scan-line format, which all vendors would support. In the past, there has been little enthusiasm for this from either the vendor community or the DICOM parent committee, which is loath to produce complex standards that are applicable only to ultrasound. (2) The vendors in question could release details of their format standards, allowing others to develop programs to analyze the data. (3) Finally, it is desired that emerging analysis packages based on the raster DICOM format will yield results that are equivalent to those obtained from the proprietary formats and analysis packages. Given the challenges of the DICOM process, it is likely that interoperability will emerge most quickly from a combination of (2) and (3).
The situation is even more challenging in 3D, where storage requirements can quickly overwhelm a network. A typical scan-line based format stores (for example) a 90 x 90 pyramid of scan lines, each with 512 8-bit data samples, requiring more than 4MB per volume or about 120MB for one second’s storage of 30 volumes/sec of 3D data. Storage requirements are even more onerous with the recently-approved DICOM 3D standard, which mandates Cartesian rather than scan-line storage. Assuming isotropic sampling at the original data rate (512 samples/scan line), a single 512 x 512 x 512 x 8 bit volume requires 134MB in storage or > 4GB for a single second of 3D imaging! While lossless run-length-encoding can reduce this by a factor of 3 or more, it is noteworthy that to date, none of the major ultrasound vendors are exporting data in the approved 3D DICOM format. One significant deterrent is the lack of an approved lossy compression technique for 3D echo, similar to the ∼15:1 JPEG compression allowed in the 2D standard. Prior work on first-generation real-time ultrasound data showed that 3D wavelet compression as great as 100:1 could be applied without significant image degradation. Whether such compression would impact 3D speckle tracking is unknown, but it certainly seems to be a fruitful area of investigation and possible adoption by the DICOM committee.
Recommendations
To increase confidence in the precision and accuracy of speckle-based measurements of myocardial deformation and torsion (across all vendors), quantifying the uncertainties associated with the measurements, and standardizing 2D and 3D data archiving formats and reported values among different echocardiographic imaging systems, specific recommendations are offered in the following key areas:
- 1
Encourage vendor interoperability to allow better workflow in advanced echo mechanics:
- •
Provide a setting, perhaps under the auspices of echocardiographic societies, where vendors can work together to improve interoperability without antitrust concerns. ASE and the European Association of Echocardiography have already established a task force for this purpose.
- •
Encourage all vendors to release details of the algorithms used to measure strain within their software packages. This is essential since “strain” can be defined in various acceptable ways, which yield discrepant values from the same deformation map.
- •
Encourage all vendors to place in the public domain (or, less ideally, via cross licensing agreements) the details of their individual scan-line formats.
- •
Explore with DICOM the possibility of a multidimensional scan-line format specific to ultrasound.
- •
Encourage the development and validation of analysis packages based on raster-based DICOM images.
- •
- 2
Identify sources of error and uncertainty in speckle-tracking based estimates of myocardial deformation and torsion (error mechanisms) and identify methods for enhancing the precision and accuracy of measurements:
- •
Develop a better understanding of the underlying physical mechanisms that influence the measured segmental and global values. How do regional intrinsic ultrasonic properties of myocardium (scattering, attenuation) affect measurements? How can the effects of overlying tissue affect measurements?
- •
Identify the impact of imaging system dependent characteristics on measurements and develop methods for the compensation and reduction of these effects on reported results.
- •
Identify the impact of variability in image acquisition and (user) analysis methods on measured values. Establish image acquisition and data analysis protocols designed to reduce variability of measurements.
- •
- 3
Develop methods for quantifying the uncertainties associated with speckle-tracking based estimates of myocardial deformation and torsion and verifying results:
- •
Establish a set of standard echocardiographic images/data sets to be used to verify analyses among different manufacturers. These might be derived from animal experiments where sonomicrometry provides a reference standard.
- •
Develop specialized, stable tissue-mimicking phantoms to be used to verify measurements. The use of phantoms with known, well-controlled properties will provide estimates of the limiting uncertainties of measurements.
- •
Encourage additional studies designed to establish the mean and standard variation of myocardial strain and rotation measurements for specific normal subject populations (e.g., age, gender, etc.). Perform these studies with multiple machines and analysis approaches to determine intervendor reproducibility.
- •
- 4
Develop methods for standardizing the reported myocardial deformation and torsion values among different echocardiographic imaging systems and enhance formats for archiving and sharing 3D and enhanced 2D image data sets:
- •
Develop a two-tiered approach for reporting myocardial deformation and torsion measurements: a set of standardized measurements and other measurements unique to each manufacturer. Establishing a set of standard measurements will permit comparison of results of data acquired with different echocardiographic imaging systems. Measurements unique to each manufacturer will continue to encourage innovation among developers. Over time, some vendor specific measurements may enter the standardized list.
- •
Establish optimal data formats and enhance image and data archiving standards (e.g., an enhanced DICOM standard) to permit the efficient storage and transfer of 3D and four-dimensional echocardiographic images and associated data.
- •
Investigate the impact of lossy 3D data compression on image quality, volumetric measurements, and speckle tracking. If acceptable, strongly urge the DICOM committee to develop a standardized method of compressing 3D data.
- •
Identify and define standard universal formats of 2D and 3D data for archiving relatively unprocessed image data permitting subsequent analyses of the data sets beyond standard image processing methods.
- •
Selected Readings
- 1
Notomi Y, Lysyansky P, Setser RM, Shiota T, Popovic ZB, Martin-Miklovic MG, et al. Measurement of ventricular torsion by two-dimensional ultrasound speckle tracking imaging. J Am Coll Cardiol 2005;45:2034-41.
- 2
Notomi Y, Martin-Miklovic MG, Oryszak SJ, Shiota T, Deserranno D, Popovic ZB, et al. Enhanced ventricular untwisting during exercise: a mechanistic manifestation of elastic recoil described by Doppler tissue imaging. Circulation. 2006;113:2524-33.
- 3
Opdahl A, Helle-Valle T, Remme EW, Vartdal T, Pettersen E, Lunde K, et al. Apical rotation by speckle tracking echocardiography: a simplified bedside index of left ventricular twist. J Am Soc Echocardiogr 2008;21:1121-8.
- 4
Seo Y, Ishizu T, Enomoto Y, Sugimori H, Yamamoto M, Machino T, et al. Validation of 3-dimensional speckle tracking imaging to quantify regional myocardial deformation. Circulation Cardiovascular Imaging 2009;2:451-9.
- 5
Ashraf M, Myronenko A, Nguyen T, Inage A, Smith W, Lowe RI, et al. Defining left ventricular apex-to-base twist mechanics computed from high-resolution 3D echocardiography: validation against sonomicrometry. JACC: Cardiovascular Imaging 2010;3:227-34.
- 6
Amundsen BH, Helle-Valle T, Edvardsen T, Torp H, Crosby J, Lyseggen E, et al. Noninvasive myocardial strain measurement by speckle tracking echocardiography: validation against sonomicrometry and tagged magnetic resonance imaging. J Am Coll Cardiol 2006;47:789-93.
- 7
Hang X, Greenberg NL, Zheng YF, Thomas JD. Compression of 3-D echocardiographic images using a modified 3-D set-partitioning-in-hierarchical-trees algorithm based on a 3-D wavelet packet transform. J Electron Imaging Apr–Jun 2006;15:023016-1.
Assessment of Global and Regional Left Ventricular Function
Global and regional function assessments are key to the clinical management of patients with cardiovascular diseases. Currently, these are usually performed qualitatively because most current quantitative methods for assessing them are not robust and are not automated. Recently, however, new methods have been introduced for quantifying cardiac function from estimates of myocardial deformation (myocardial strain and strain rate) and torsion (spatial variation of cardiac rotation) based on analyses of ultrasonic myocardial speckle patterns over the cardiac cycle. While these methods show great promise for assessing alterations in global and segmental myocardial performance, they currently exhibit a wide variation in the reported values with a significant dependence on the imaging system used as well as the image quality. Hence, there is a need to standardize the measurements among the echocardiographic systems employed. Additionally, for these measurements to be clinically useful, their precision, accuracy, and reproducibility must be significantly improved. Furthermore, the current standards are not optimized to permit the efficient storage and transfer of three-dimensional (3D) echocardiographic cineloops and related information.
The formation of echocardiographic images and associated measurements based on analyses of speckle pattern data depend upon the processing and detailed characterization of received backscattered ultrasonic signals from the myocardium, which are dependent upon the intrinsic ultrasonic scattering and attenuation properties of the myocardium, the frequency-dependent attenuation properties of overlying tissues (e.g., chest wall), and the specific features of individual imaging systems. The specific characteristics of each type of imaging system have a profound impact on the detailed nature of the received backscattered signals and subsequent speckle patterns observed and include transmit and receive beam profiles, line density, frequency bandwidths, and pulse lengths. Because each manufacturer implements uniquely different choices of system-dependent characteristics, the received signals and features of resultant speckle patterns can give rise to system-dependent variations in measured values. In addition, manufacturers often choose to measure and report slightly different realizations of myocardial strain parameters. This makes the data acquired and analyzed using different echocardiographic imaging systems difficult to compare.
In addition to the technical issues associated with the nature of backscattered signals and characteristics of echocardiographic imaging systems that can have a significant impact on measurements, the effects of image acquisition and user analysis (e.g., the placement of the region-of-interest) also impact the variability of measured values. Although it is generally accepted that poor or non-standard echocardiographic windows can affect results of measurements, the degree of this effect is unknown. The effect of region-of-interest placement on measurement variability has not been systematically investigated. It is expected that precision will be adversely impacted as regions-of-interest grow smaller.
Utilization of myocardial deformation and torsion measurements will require knowledge of the range of normal values. The mean values and associated variations of these parameters for specific cohorts of normal subjects need to be firmly established before routine clinical adoption of myocardial deformation and torsion measurements can be implemented. Variability can take several forms. The easiest to achieve consistency should be with the same cardiac cycle data being analyzed by the same software. To the extent that this is fully automated, the same results should be achieved each time. If there is manual intervention, there may be variability based on precise placement of regions-of-interest, but such variability should be small. Analysis of the same acquisition by a second software package might also be considered; this may yield either random or systematic deviation from the first result depending on whether the two packages use the same mathematical algorithm to estimate strain. Even this simple test is often impossible to do, since most of the vendors store their data in proprietary scan line format, which can only be analyzed by their own software package. Additionally, analysis of successive beats within the same study should be assessed, using either the same software package or a different one. Finally, the ultimate test of variability is the test-retest assessment whereby the patient is scanned twice (with either the same or, more relevantly, another scanner), and the data are analyzed independently.
Standard methods for characterizing images and measurements obtained with ultrasonic imaging systems are available from a number of sources including the International Electrotechnical Commission, the American Institute of Ultrasound in Medicine, and the American Association of Physicists in Medicine. These approaches typically utilize images of commercially available tissue-mimicking phantoms and are largely designed to assess properties such as spatial and contrast resolution, sensitivity and dynamic range, and geometric measurement accuracy on static images. In addition, there are somewhat more limited approaches described for characterizing Doppler measurements using special “flow” phantoms. However, to date, there are no standard methods or commercially available tissue-mimicking phantoms designed to assess echocardiographic-specific measurements of myocardial deformation and rotation. The only such standards to date have been short lifetime models, ones utilizing ex-vivo porcine hearts with implanted sonomicrometry crystals and gel-based models that can be compressed, but unfortunately degrade within days. The development of standard characterization methods and specialized, well-characterized phantoms would provide an objective approach to estimate the limiting uncertainties of these echocardiographic image based measurements.
Image data archiving standards and formats are not currently optimized to handle all the types and large amounts of data associated with volumetric echocardiographic imaging and advanced image analysis features. For example, the current Digital Imaging and Communications in Medicine (DICOM) standard for two-dimensional (2D) echocardiographic images only accepts pixels in a Cartesian, raster-based orientation. Unfortunately, speckle tracking for most of the major vendors is performed on proprietary formats based on individual scan-lines. Full interoperability in speckle tracking will require collaboration to harmonize formats. There are three possible approaches to this: (1) the DICOM committee (and appropriate companies) could agree upon a single scan-line format, which all vendors would support. In the past, there has been little enthusiasm for this from either the vendor community or the DICOM parent committee, which is loath to produce complex standards that are applicable only to ultrasound. (2) The vendors in question could release details of their format standards, allowing others to develop programs to analyze the data. (3) Finally, it is desired that emerging analysis packages based on the raster DICOM format will yield results that are equivalent to those obtained from the proprietary formats and analysis packages. Given the challenges of the DICOM process, it is likely that interoperability will emerge most quickly from a combination of (2) and (3).
The situation is even more challenging in 3D, where storage requirements can quickly overwhelm a network. A typical scan-line based format stores (for example) a 90 x 90 pyramid of scan lines, each with 512 8-bit data samples, requiring more than 4MB per volume or about 120MB for one second’s storage of 30 volumes/sec of 3D data. Storage requirements are even more onerous with the recently-approved DICOM 3D standard, which mandates Cartesian rather than scan-line storage. Assuming isotropic sampling at the original data rate (512 samples/scan line), a single 512 x 512 x 512 x 8 bit volume requires 134MB in storage or > 4GB for a single second of 3D imaging! While lossless run-length-encoding can reduce this by a factor of 3 or more, it is noteworthy that to date, none of the major ultrasound vendors are exporting data in the approved 3D DICOM format. One significant deterrent is the lack of an approved lossy compression technique for 3D echo, similar to the ∼15:1 JPEG compression allowed in the 2D standard. Prior work on first-generation real-time ultrasound data showed that 3D wavelet compression as great as 100:1 could be applied without significant image degradation. Whether such compression would impact 3D speckle tracking is unknown, but it certainly seems to be a fruitful area of investigation and possible adoption by the DICOM committee.
Recommendations
To increase confidence in the precision and accuracy of speckle-based measurements of myocardial deformation and torsion (across all vendors), quantifying the uncertainties associated with the measurements, and standardizing 2D and 3D data archiving formats and reported values among different echocardiographic imaging systems, specific recommendations are offered in the following key areas:
- 1
Encourage vendor interoperability to allow better workflow in advanced echo mechanics:
- •
Provide a setting, perhaps under the auspices of echocardiographic societies, where vendors can work together to improve interoperability without antitrust concerns. ASE and the European Association of Echocardiography have already established a task force for this purpose.
- •
Encourage all vendors to release details of the algorithms used to measure strain within their software packages. This is essential since “strain” can be defined in various acceptable ways, which yield discrepant values from the same deformation map.
- •
Encourage all vendors to place in the public domain (or, less ideally, via cross licensing agreements) the details of their individual scan-line formats.
- •
Explore with DICOM the possibility of a multidimensional scan-line format specific to ultrasound.
- •
Encourage the development and validation of analysis packages based on raster-based DICOM images.
- •
- 2
Identify sources of error and uncertainty in speckle-tracking based estimates of myocardial deformation and torsion (error mechanisms) and identify methods for enhancing the precision and accuracy of measurements:
- •
Develop a better understanding of the underlying physical mechanisms that influence the measured segmental and global values. How do regional intrinsic ultrasonic properties of myocardium (scattering, attenuation) affect measurements? How can the effects of overlying tissue affect measurements?
- •
Identify the impact of imaging system dependent characteristics on measurements and develop methods for the compensation and reduction of these effects on reported results.
- •
Identify the impact of variability in image acquisition and (user) analysis methods on measured values. Establish image acquisition and data analysis protocols designed to reduce variability of measurements.
- •
- 3
Develop methods for quantifying the uncertainties associated with speckle-tracking based estimates of myocardial deformation and torsion and verifying results:
- •
Establish a set of standard echocardiographic images/data sets to be used to verify analyses among different manufacturers. These might be derived from animal experiments where sonomicrometry provides a reference standard.
- •
Develop specialized, stable tissue-mimicking phantoms to be used to verify measurements. The use of phantoms with known, well-controlled properties will provide estimates of the limiting uncertainties of measurements.
- •
Encourage additional studies designed to establish the mean and standard variation of myocardial strain and rotation measurements for specific normal subject populations (e.g., age, gender, etc.). Perform these studies with multiple machines and analysis approaches to determine intervendor reproducibility.
- •
- 4
Develop methods for standardizing the reported myocardial deformation and torsion values among different echocardiographic imaging systems and enhance formats for archiving and sharing 3D and enhanced 2D image data sets:
- •
Develop a two-tiered approach for reporting myocardial deformation and torsion measurements: a set of standardized measurements and other measurements unique to each manufacturer. Establishing a set of standard measurements will permit comparison of results of data acquired with different echocardiographic imaging systems. Measurements unique to each manufacturer will continue to encourage innovation among developers. Over time, some vendor specific measurements may enter the standardized list.
- •
Establish optimal data formats and enhance image and data archiving standards (e.g., an enhanced DICOM standard) to permit the efficient storage and transfer of 3D and four-dimensional echocardiographic images and associated data.
- •
Investigate the impact of lossy 3D data compression on image quality, volumetric measurements, and speckle tracking. If acceptable, strongly urge the DICOM committee to develop a standardized method of compressing 3D data.
- •
Identify and define standard universal formats of 2D and 3D data for archiving relatively unprocessed image data permitting subsequent analyses of the data sets beyond standard image processing methods.
- •
Selected Readings
- 1
Notomi Y, Lysyansky P, Setser RM, Shiota T, Popovic ZB, Martin-Miklovic MG, et al. Measurement of ventricular torsion by two-dimensional ultrasound speckle tracking imaging. J Am Coll Cardiol 2005;45:2034-41.
- 2
Notomi Y, Martin-Miklovic MG, Oryszak SJ, Shiota T, Deserranno D, Popovic ZB, et al. Enhanced ventricular untwisting during exercise: a mechanistic manifestation of elastic recoil described by Doppler tissue imaging. Circulation. 2006;113:2524-33.
- 3
Opdahl A, Helle-Valle T, Remme EW, Vartdal T, Pettersen E, Lunde K, et al. Apical rotation by speckle tracking echocardiography: a simplified bedside index of left ventricular twist. J Am Soc Echocardiogr 2008;21:1121-8.
- 4
Seo Y, Ishizu T, Enomoto Y, Sugimori H, Yamamoto M, Machino T, et al. Validation of 3-dimensional speckle tracking imaging to quantify regional myocardial deformation. Circulation Cardiovascular Imaging 2009;2:451-9.
- 5
Ashraf M, Myronenko A, Nguyen T, Inage A, Smith W, Lowe RI, et al. Defining left ventricular apex-to-base twist mechanics computed from high-resolution 3D echocardiography: validation against sonomicrometry. JACC: Cardiovascular Imaging 2010;3:227-34.
- 6
Amundsen BH, Helle-Valle T, Edvardsen T, Torp H, Crosby J, Lyseggen E, et al. Noninvasive myocardial strain measurement by speckle tracking echocardiography: validation against sonomicrometry and tagged magnetic resonance imaging. J Am Coll Cardiol 2006;47:789-93.
- 7
Hang X, Greenberg NL, Zheng YF, Thomas JD. Compression of 3-D echocardiographic images using a modified 3-D set-partitioning-in-hierarchical-trees algorithm based on a 3-D wavelet packet transform. J Electron Imaging Apr–Jun 2006;15:023016-1.
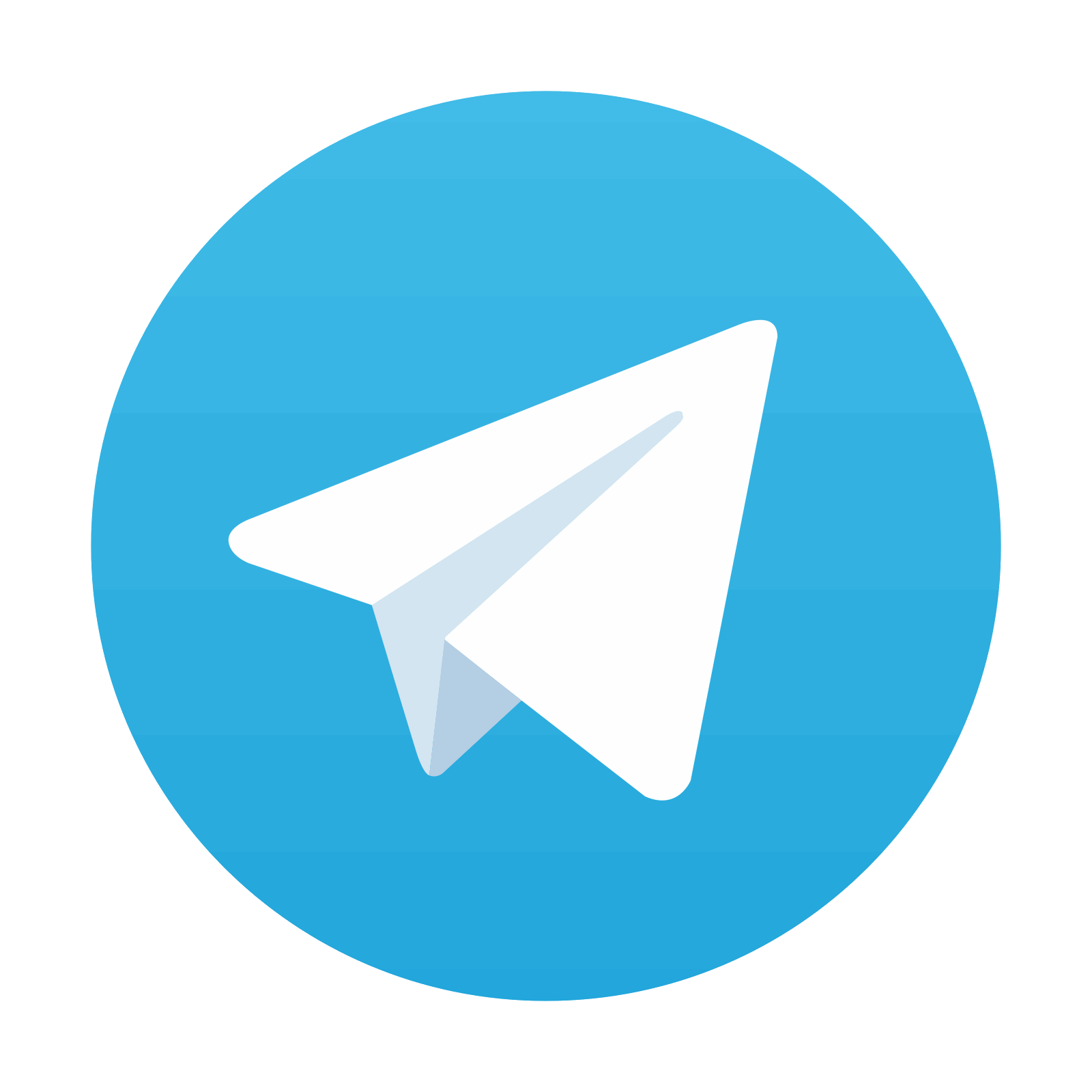
Stay updated, free articles. Join our Telegram channel

Full access? Get Clinical Tree
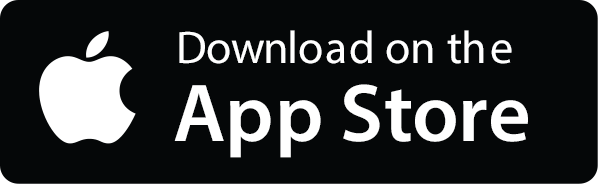
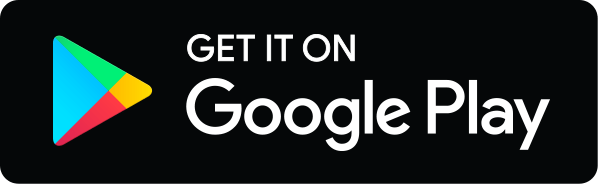