Highlights
- •
There is no consensus on host-specific TB biomarkers for diagnosis or treatment response.
- •
Testing multiple cytokines aids in the diagnosis of active and latent TB in children.
- •
There are constraints associated with technological advancements in TB diagnostics.
- •
Biomarkers of high sensitivity and specificity are urgently required.
Educational Aims
The reader will come to appreciate that:
- •
Currently, there is no consensus on definitive host-specific biomarkers for active or latent TB disease, reactivation, treatment response, and diagnosis.
- •
Testing multiple cytokines aids in active TB diagnosis and distinguishing it from latent infection, particularly in infants with immature immune systems.
- •
There are constraints associated with technological advancements in TB diagnostics.
- •
The urgent necessity to find finding biomarkers of high sensitivity and specificity in TB-infected children will save many lives.
Abstract
Background
Tuberculosis (TB) is one of the most common causes of mortality globally with a steady rise in paediatric cases in the past decade. Laboratory methods of diagnosing TB and monitoring response to treatment have limitations. Current research focuses on interrogating host- and/or pathogen-specific biomarkers to address this problem.
Methods
We reviewed the literature on host-specific biomarkers in TB to determine their value in diagnosis and treatment response in TB infected and HIV/TB co-infected children on anti-tuberculosis treatment.
Results and Conclusion
While no single host-specific biomarker has been identified for diagnosis or treatment responses in children, several studies suggest predictive biosignatures for disease activity. Alarmingly, current data on host-specific biomarkers for diagnosing and assessing anti-tuberculosis treatment in TB/HIV co-infected children is inadequate. Various factors affecting host-specific biomarker responses should be considered in interpreting findings and designing future studies within specific clinical settings.
Background and rationale for the review
Despite being declared a “global emergency” by the World Health Organization (WHO) in 1993, tuberculosis (TB) remains a significant health concern especially in developing countries . The burden of disease is high in children with an estimated 997 500 new cases identified globally in 2019 . In 2020, the WHO estimated that 9.9 million people were reported ill with TB; with more than 1.3 million deaths, eight percent of which were children . The paediatric burden of the disease may be an underestimate as it is difficult to definitively diagnose the condition . Younger children (< two years old) are at greater risk of progression to active TB disease than adults . Co-infection with human immunodeficiency virus (HIV) has been an important contributing factor in the resurgence of TB, especially if the CD4+ T cell and innate immune responses are compromised or defective . The improved accessibility of antiretroviral therapy (ART) has prompted greater focus on TB screening, leading to an influx of new patients being seen in the healthcare system. McLaren et al. found that during the 2004-2008 ART roll-out in South Africa, there was a significant increase of 3.7% in patients tested for TB and 3.2% in repeat testing, based on the National Health Laboratory Services (NHLS) data of 10,544,350 TB patients, aged 16-64 years, from January 2003 to December 2011.
The current laboratory methods of diagnosing pulmonary TB viz. the smear microscopy, the tuberculin skin test (TST) and interferon gamma release assays (IGRAs) based on in vitro T cell responses, though useful, have limitations in their ability to differentiate between active disease and latent infection . Culture is the gold standard for the diagnosis of TB, but confirmation can take weeks and is reliant on samples being appropriately taken . In children, sputum samples of adequate quantity and quality are difficult to obtain, and the disease is often paucibacillary . Molecular methods such as the Gene Xpert Ultra have improved diagnostic yields as compared to smear microscopy but are still inadequate in accurately confirming disease and require additional samples for culture .
Since the 1990s, cytokines and chemokines have been studied for their potential in TB diagnosis. Advanced detection methods like flow cytometry and Luminex, enabling rapid simultaneous detection of multiple cytokines, have fueled the exploration for host-produced biomarkers in response to Mycobacterium tuberculosis (MTB) infection in both adults and children. The intricate interplay between complex mycobacterial antigens and region of difference (RD) influences the activation of pro-inflammatory Type 1 T helper cell (Th1), and Th2 cytokines that are responsible for eliciting a range of protective and pathological responses . Identification of multiple cytokine profiles helps in reducing the impact of variability of cytokine synthesis and release in TB patients .
Rationale for host-specific biomarkers in tuberculosis
A biomarker is an objective indicator of normal biological processes that are altered in a disease state . A surrogate biomarker is one that predicts the effect of the pharmacological agent used as a therapeutic intervention . Biomarkers for TB should be able to differentiate between healthy and infected persons and between latent infection and active disease. They should have low levels in unstimulated samples, not respond to antigen from uninfected individuals, but show a strong response to antigen in infected individuals . Host-specific biomarkers refer to inflammatory indices produced by the human host in response to an invading pathogen or inflammation but are generally not disease-specific . One group of host-specific biomarkers are the cytokines and chemokines, which are molecules involved in regulating immune responses at the cellular level . Currently, there is no consensus on definitive host-specific biomarkers for active or latent TB disease, reactivation, treatment response, and diagnosis, despite several reported cytokine profiles and biosignatures in TB patients. The knowledge on the host-MTB interaction and immune mechanisms of paediatric TB cases is scanty . Baseline biomarker differences help categorise patients for tailored treatment . Testing multiple cytokines aids in active TB diagnosis and distinguishing it from latent infection, particularly in infants with immature immune systems . Biomarkers for early treatment response can replace repeat sputum culture or smear testing . They contribute to establishing effective clinical endpoints for cure and assessing the duration and efficacy of new anti-tuberculosis drugs , essential for effective treatment and control programs . Rapid advances in technology have allowed for novel insights into ribonucleic acid (RNA) sequencing and transcriptome profiles of whole blood of TB patients and investigating gene expression signatures predictive of TB disease . Limitations of these technologies includes lack of uniformity in methodologies around sequencing platforms, biases arising from cDNA library construction and sequence alignment that distorts the quantification of transcripts, and difficulties when attempting to achieve accurate sequence annotation, making accurate data interpretation difficult . This literature review examines host-specific cytokines/chemokines in relation to diagnosis and response to anti-tuberculosis treatment in children with pulmonary TB (PTB) from 2005 to 2022. However, we did not assess the literature for cytokine/chemokines based on disease severity, TB type (including extrapulmonary TB), relapse, reactivation, or reinfection. Additionally, transcriptomic markers, matrix metalloproteinase, tissue inhibitors of metalloproteinases, or gene expression signatures of TB in children are not covered.
Methods
Several databases (viz. ScienceDirect, Scirus, PubMed, Unboundmedicine, Google Scholar, Springer Online Archives, Elsevier) were accessed and search words “tuberculosis”, “TB”, “children”, “pediatric” or “paediatric”, “biomarkers”, “cytokines”, “host-specific”, “diagnosis”, “pulmonary”, “HIV” or “human immunodeficiency virus” and “response to treatment” were employed. Studies related to MTB specific and RNA transcription and host- and MTB-specific genetic biomarkers were excluded. Seventeen studies, published in the English language that directly referred to host-specific (i.e. cytokine) biomarkers in children (15 diagnostic; one response to anti-tuberculosis treatment; one HIV/TB coinfection) were included.
Host-specific biomarkers in the diagnosis of childhood pulmonary tuberculosis
Biomarkers in active TB as compared to latent TB infection
Here the data is conflicting.
Several studies have shown raised interferon gamma-induced protein (IP)-10 levels in both active and latent TB infected children with levels not being able to distinguish the difference . Interferon gamma (IFN-γ), which elicits a Th1 response to MTB, and regulates IP-10 production by neutrophils and monocytes, was found to be similar in active disease and LTBI . Plasma IP-10 levels were higher in children diagnosed with active TB and LTBI than those with non-tuberculous mycobacteria lymphadenopathy and other respiratory tract infections, suggesting that, in children, the IP-10 response is more potent in infection with MTB compared to other microorganisms . Significant increase in IFN-γ, interleukin (IL)-2, IL-5, IL-13 was observed by Armand et al. in PTB and LTBI in children but these could not distinguish between active and latent disease. The findings of Terbruegge et al. (IP-10) and Nausch et al. (IP-10, IL-13, TNF-α, and granulocyte-macrophage colony-stimulating factor (GM-CSF)) suggest that the selected host-specific biomarkers cannot differentiate active disease from LTBI.
The expression of IL-21 and soluble CD40 ligand (sCD40L) differed significantly in children with active disease and LTBI compared to healthy controls, as well as in those with active disease compared to LTBI patients . A possible explanation for this is that IL-21 produced by CD4 cells, regulates the production of immunoglobulins by B and T cells. When combined with CD40L, IL-21 stimulates memory B cells to produce IL-10. In addition, the expression of granzyme B by IL-10-producing B cells limits T cell proliferation leading to immune suppression .
Chegou et al. were able to show a biosignature of IL-1RA, IP-10, and vascular endothelial growth factor (VEGF) being able to distinguish active disease from LTBI. A plausible reason could be that interferon alpha two (IFN-α2), a key player in the differentiation of monocytes into dendritic cells, increases the production of IP-10 while high levels of VEGF are produced by macrophages and other inflammatory cells near tubercular lesions .
Sudbury et al. reported increased levels of IFN-γ, IL-1RA, IL-2, IL-13, IP-10, tumor necrosis factor (TNF)-α, and macrophage inflammatory protein-1beta (MIP-1β) in children with active disease compared to those with LTBI. It is possible that abnormal levels of these biomarkers could indicate a subclinical inflammatory response to MTB and potentially predict a risk of progression from LTBI to active disease in children . They also reported increased levels of IL-1RA in children with active disease compared to those with LTBI in a TB endemic setting in Peru, similar to a finding by Anbarasu et al. that IL-1RA may have a role as a “stage-specific marker” as its values differ significantly in latent and active disease. Dreesman et al. found that the ratio of proportions of early-secreted-antigenic target-6 (ESAT-6)-induced IFN-γ and TNF-α CD4+ T lymphocytes was able to discriminate active disease from LTBI in children three-15 years of age while IL-17 CD4+ T lymphocytes was associated with progression to active disease in those under three years of age. This finding suggests that the immaturity of the immune system needs to be taken into consideration when evaluating cytokine responses in the diagnosis of young children with active TB.
Kumar et al. showed a significantly increased CCL1, CCL3 (MIP-1α), CXCL1, CXCL2 (MIP-2α), CXCL9 and CXCL10 (IP-10) levels in children with active TB compared to LTBI. The potential role of IL-13 as a diagnostic marker for active TB infection in children still requires further investigation as this was not confirmed by Dhanasekaran et al. who observed increased expression of this cytokine in children with LTBI.
Latent TB infection as compared to TB uninfected controls
Limited data was reported in the literature when comparing these two conditions. A significant increased expression of IL-2 and IL-13 was found in children with LTBI than in uninfected controls .
Active TB as compared to unconfirmed TB cases and controls with other respiratory illnesses
Significantly higher levels of IP-10, TNF-α, IL-1RA, IL-2, IL-13, and MIP-1β were found in LTBI and active TB cases than in TB-uninfected children . IFN-γ, IL- 2, IP-10, IL-13, IL-1α, TNF-α and GM-CSF were significantly raised in children with TB and LTBI compared to non-infected controls . Togun et al. found a biosignature of IL-1RA, IL-7 and IP-10 in blood samples unstimulated by MTB antigens that could distinguish active TB from other non-TB respiratory diseases. Significantly elevated levels of Th1 cytokines viz. IFN-γ, TNF-α, IL-2, IL-17A, IL-1α and IL-6 were reported by Kumar et al. in confirmed ( n = 44) and unconfirmed TB ( n = 47) versus unlikely TB cases ( n = 76). A recent study reported higher baseline levels of (CC chemokines) CCL1, CCL3 (MIP-1α), CXCL1, CXCL2 (MIP-2α) and CXCL10 (IP-10) in children with active TB ( n = 44) compared to those where TB was thought to be unlikely ( n = 76) . CCL1, CCL3, CXCL1, CXCL10 were significantly higher in those with bilateral or cavitary disease compared to unilateral cases or those without cavitary lesions. Since increased IL-1RA and IL-6 were significantly associated with cavitary disease in adults , it is possible that these biomarkers are also produced in higher concentrations at the site of the lesions in children and are predictive of the severity of the disease.
A biosignature of increased IFN-γ, IL-1RA, IL-2, IL-13, IP-10, MIP-1β and TNF-α was observed in children with active TB compared to unconfirmed cases . These findings increase the specificity for active disease of these cytokines, when combined as a biosignature. Kumar et al. found increased CCL1, CCL3 (MIP-1α), CXCL1, CXCL2 (MIP-2α), and CXCL10 (IP-10) in children with confirmed and unconfirmed active TB.
Active TB as compared to healthy controls
Sudbury et al. found increased IFN-ɣ, IL-1RA, IL-2, IL-13, IP-10, MIP-1β and TNF-α response in children with active TB and LTBI than in both healthy controls. Children with active TB presented with markedly decreased levels of IFN-γ, TNF-α, IL-4, IL-13 and IL-17 compared to healthy controls . Druszczynska et al. reported decreased expression of IL-17 A, and pentax 3 in children with active disease than in healthy controls, thus indicating that the severity of active disease may impact on the cytokine response. This is supported by the findings of Kumar et al. of significantly increased CCL1, CCL3 (MIP-1α), CXCL1, CXCL2 (MIP-2α), CXCL9 and CXCL10 (IP-10) levels in children with active TB compared to healthy controls. IFN-α2, IL-1RA, sCD40 and VEGF were identified as distinguishing active disease ( n = 19) from no disease in children ( n = 57) . Moreover, it was reported that these biomarkers were especially useful when combined as biosignatures.
Summary
Although results of biomarkers and biosignature are encouraging, reaching a definitive clinical diagnosis by these tests can be challenging as there is some overlap in the different biomarker signatures that are used to reach a diagnosis of confirmed active TB as compared to LTBI, respiratory illness with unconfirmed TB in children and healthy controls. IFN-γ, IP-10, MIP-1α, CCL1, CXCL1, and IL-1RA ( Table 1 ) emerge as the best and robust candidates for diagnostic markers in active paediatric PTB. Some of this overlap is related to the inability to clearly define the diagnosis of TB in children due to the lack of a consistent universal finding of a positive culture in all cases of childhood TB.
Active PTB | Active PTB compared to LTBI | Active PTB compared to HC* | LTBI compared to UIC** | Active PTB compared to UCC*** & SC**** | HIV/TB co-infection compared to TB infection |
---|---|---|---|---|---|
Biosignature:Active PTB compared to no disease:IFN-α2 ,sCD40 ,IL1-RA ,Active PTB compared to other respiratory diseases:IL-1RA ,IL-7 ,IP-10 Increased in active PTB compared to unlikely TB:CCL1 ,CXCL1 ,MIP-1α ,MIP-2α ,IP-10 | Increased in active PTB & LTBI but no significant difference:IP-10 ,IL-1RA ,IL-13 ,TNF-α ,GM-CSF ,VEGF ,Significantly increased in active PTB compared to LTBI:IFN-γ ,IL-1RA ,IL-2 ,IL-13 ,IP-10 ,TNF-α ,MIP-1β ,CCL1 ,CXCL1 ,CXCL9 ,MIP-1α ,MIP-2α Increased in LTBI compared to active PTB:IL-13 | Decreased in active PTB compared to HC:IFN-γ ,TNF-α ,IL-4 ,IL-13 ,IL-17 ,Pentax 3 Increased in active PTB compared to HC:IFN-γ ,IP-10 ,TNF-α ,IL-1RA ,IL-2 ,IL-13 ,MIP-1β ,GM-CSF ,CCL1 ,CXCL1 ,CXCL9 ,MIP-1α ,MIP-2α | Increased in LTBI compared to UIC:IL-2 ,IL-13 | Increased in active PTB compared to UCC:IL-1α ,IL-6 ,IL-16 ,IL-17 CCL1 ,MIP-1α ,CXCL1 ,MIP-2α ,IP-10 ,IFN-γ ,IL-1RA ,IL-2 ,IL-13 ,MIP-1β ,TNF-α | Decreased in TB/HIV co-infection compared to TB infection:IL-21 ,IP-10 |
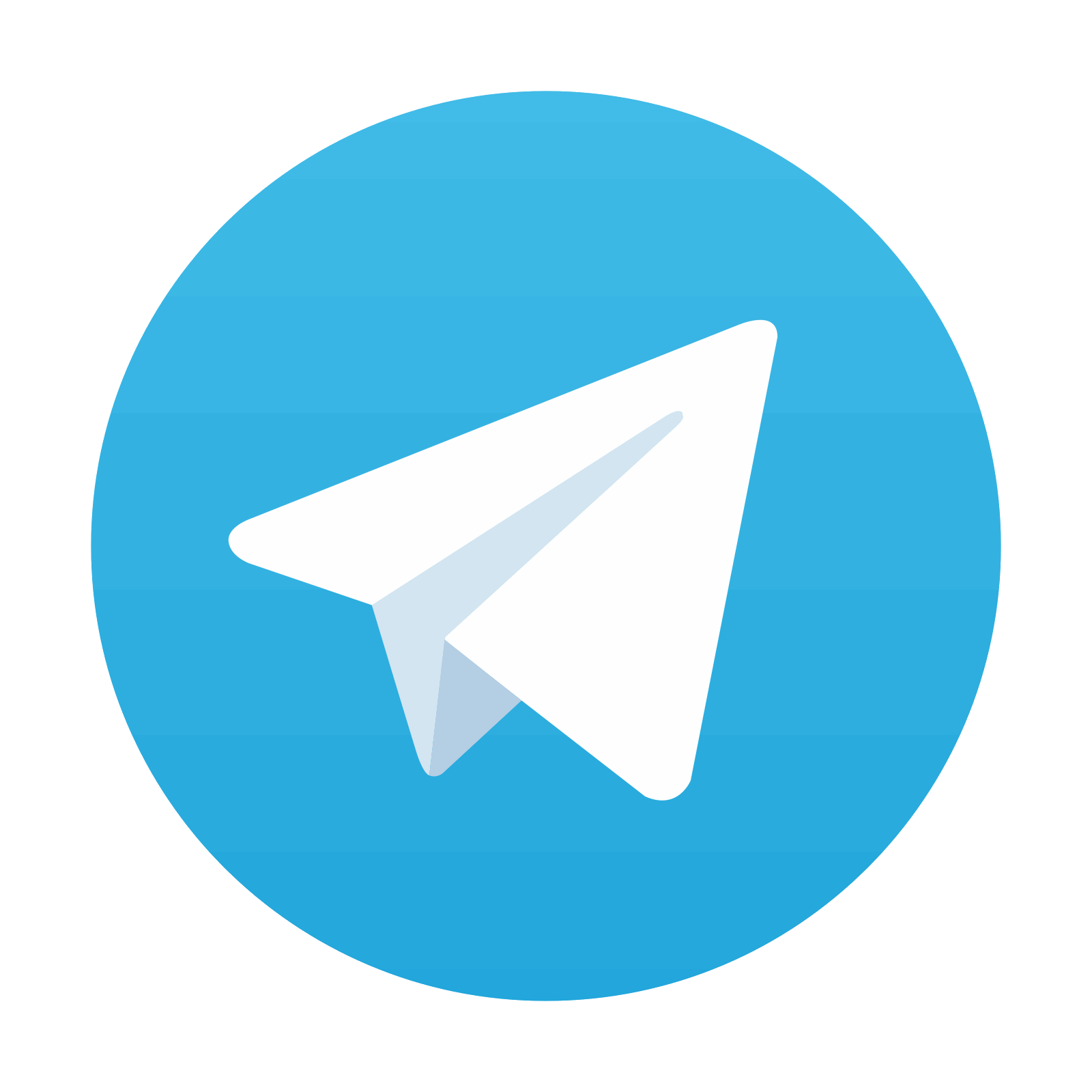
Stay updated, free articles. Join our Telegram channel

Full access? Get Clinical Tree
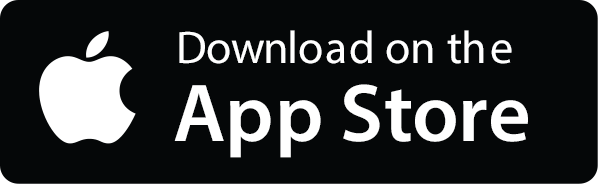
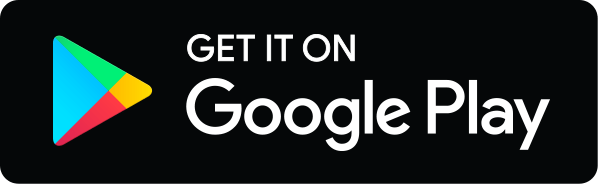
