Background
The aim of this study was to assess a novel transthoracic echocardiographic method to estimate the severity of pulmonary regurgitation (PR) in patients with surgically repaired tetralogy of Fallot.
Method
In 63 patients with operated tetralogy of Fallot, PR was evaluated by vena contracta width, jet deceleration, PR index, pressure half-time, and a new index, referred to as Pulmonary Regurgitation Index by M-mode echocardiography (PRIME), which is the systolic-to-diastolic variation in right pulmonary artery diameter. The results were matched to PR fraction (PRF) assessed by cardiovascular magnetic resonance imaging. PRIME cutoff values for selecting patients with mild, moderate, and severe PR were identified by maximizing PRIME sensitivity and specificity. Nonlinear regression by 3-parameter logistic function was used to estimate PRF by PRIME.
Results
The sensitivity and specificity of PRIME were high for all diagnostic targets: PRF ≥15% versus <15%, PRF ≥25% versus <25%, and PRF >40% versus ≤40%. The nonlinear regression model showed a good correlation between PRF and PRIME ( R 2 = 0.95).
Conclusion
PRIME is a simple and accurate method to estimate PR by transthoracic echocardiography in patients with operated tetralogy of Fallot.
Pulmonary regurgitation (PR) after the surgical correction of tetralogy of Fallot (TOF) is a common occurrence, resulting in a hemodynamic burden that leads to exercise intolerance, arrhythmia, heart failure, and even death, mainly from the third postoperative decade and thereafter. Because of the important prognostic and management implications in such a patient setting, it is important to provide an accurate quantification of PR. This is generally achieved using echocardiography, which, however, still offers only suboptimal performance. Doppler echocardiographic techniques can usually distinguish between mild and severe PR but are only semiquantitative methods and do not allow accurate measurements of PR volume or PR fraction (PRF). Several studies have demonstrated that accurate measurements of PR expressed as the PRF can be achieved using cardiovascular (CV) magnetic resonance imaging (MRI) by means of phase velocity contrast (PVC) acquisition. However, CV MRI is expensive, time consuming, and not always available.
In examining the cine MR sequences of patients with large amounts of PR, we have consistently noticed large variations in the diameter of the pulmonary artery branches from systole to diastole. This finding strikingly contrasts with the usual normal pattern of small cyclic diameter variation in patients with no or trivial PR. Although cine MR loops immediately show cyclic variations in pulmonary artery diameter ( Figure 1 ), the same can be easily derived from M-mode techniques as well, during standard transthoracic echocardiography (TTE), when appropriately searched for. Therefore, our aim was to formally test our empirical observation, correlating the PRF assessed on CV MRI with the right pulmonary artery (RPA) systolic-diastolic diameter variation assessed on TTE. We chose the RPA because its long axis is easily imaged using two-dimensional (2D) echocardiography from the suprasternal approach, and its systolic-diastolic diameter variation can be accurately measured using the M-mode technique.

Methods
The study initially considered 74 consecutive patients with TOF who had been operated and were scheduled for CV MRI; of these, 11 were excluded because of previous pulmonary artery stent implantation. The remaining 63 patients (see Table 1 for demographic and surgical data and Table 2 for clinical and instrumental characteristics) underwent comprehensive transthoracic echocardiographic and CV MRI exams on the same day. Eleven were evaluated both before and after pulmonary valve implantation, and only the preoperative evaluation was considered in the overall population study. Thirty age-matched and gender-matched healthy volunteers with trivial or physiologic PR were evaluated using TTE. Consideration was also given to all anatomic or surgical patient characteristics that could interfere with cyclic RPA diameter variation, such as (1) pulmonary artery stenosis (8 patients with single pulmonary artery stenoses, defined as left or right pulmonary perfusion <35% or 40%, respectively, by PVC MRI, and 2 patients with bilateral pulmonary artery stenoses, defined by means of MR angiography); (2) right ventricular (RV) outflow tract obstruction (8 patients), defined as maximal systolic Doppler gradient ≥ 40 mmHg; (3) dilated RPA (17 patients), defined as z score > 2; (4) hypoplastic RPA (9 patients), defined as z score < −1.5; (5) previous aortopulmonary shunt (17 patients; 2); and (6) poor RV function (8 patients), defined as RV ejection fraction < 45% on CV MRI (see Tables 1 and 2 ).
Variable | Value |
---|---|
Number of patients | 63 |
Patients aged <18 y | 36 |
Patients aged ≥18 y | 27 |
Age (y) | 17 ± 9.8 (3-43) |
Diagnosis at birth | |
TOF | 53 (84%) |
Pulmonary atresia + VSD | 8 (13%) |
DORV (Fallot type) | 2 (3%) |
Mean age at total repair (y) | 2.3 ± 2.8 (0.1-13) |
Previous palliative intervention | 20 (31.5%) |
Aortopulmonary shunt | 17 (27%) |
Surgical MAPCA closure | 2 (3%) |
Outflow patch | 1 (1.5%) |
Patients with ≥2 CPB operations at study | 18 (28%) |
Type of RV outflow tract at study | |
Transannular patch | 47 (74.5%) |
Prosthetic valve | 6 (9.5%) |
Homograft | 4 (6.5%) |
Heterograft | 1 (1.5%) |
Biologic valve | 1 (1.5%) |
Infundibular resection with patch | 6 (9.5%) |
Infundibular resection without patch | 4 (6.5%) |
Follow-up from total correction (y) | 14.5 ± 7.8 (2-32) |
Variable | Value |
---|---|
Number of patients | 63 |
QRS duration on ECG (s) | 0.14 ± 0.03 (0.08 to 0.2) |
MRI | |
Indexed RV EDV (mL/m 2 ) | 133 ± 43 (48 to 278) |
Indexed RV ESV (mL/m 2 ) | 61 ± 26 (16 to 133) |
RV EF (%) | 55 ± 8 (30 to 73.5) |
Indexed LV EDV (mL/m 2 ) | 77 ± 14.5 (45 to 123) |
Indexed LV ESV (mL/m 2 ) | 29 ± 9 (12 to 60) |
LV EF (%) | 63 ± 7 (44 to 77) |
PRF (%) | 32 ± 16 (0 to 60) |
RV outflow tract obstruction † | 8 (14%) |
Site of obstruction | |
Valvular | 5 (9.8%) |
Infundibular | 3 (4.2%) |
Pulmonary branch stenosis | 10 (16%) |
Left pulmonary stenosis ‡ | 4 (6.5%) |
Right pulmonary stenosis ¶ | 4 (6.5%) |
Bilateral pulmonary stenosis § | 2 (3%) |
Right pulmonary artery diameter z score | 1 ± 1.98 (−2.9 to +7.7) |
Dilated RPA ∗ | 17 (27%) |
Hypoplastic RPA # | 9 (14%) |
Tricuspid regurgitation on Doppler echocardiography | |
Absent | 4 (6%) |
Trivial | 37 (57%) |
Mild | 19 (32%) |
Moderate | 3 (5%) |
RV systolic pressure/systemic pressure | 0.43 ± 0.16 (0.23 to 1.1) |
† Defined as Doppler gradient ≥ 40 mm Hg.
‡ Defined as a left perfusion on CV MRI <35% of total pulmonary flow.
§ Evaluated by MR angiography.
¶ Defined as right pulmonary perfusion at CV-MRI less than 40 % of total pulmonary flow.
Echocardiography
TTE was performed on all patients using System Five and Vivid 7 scanners (GE Medical Systems, Milwaukee, WI) interfaced with a multifrequency transducer. In accordance with the recommendations of the American Society of Echocardiography, PR was evaluated in several ways: by PR jet deceleration time; by vena contracta width; by PR index, as proposed by Li et al ; and by pressure half-time, as suggested by Silversides et al. In addition, we tested a new index, which we termed the PR index by M-mode echocardiography (PRIME), which measures the systolic-to-diastolic RPA diameter variation assessed by 2D targeted M-mode echocardiography.
To evaluate this latter index, we first visualized the RPA long-axis from the 2D suprasternal approach, as described by Snider et al. We then performed M-mode echocardiography after positioning the cursor perpendicularly to the proximal RPA ( Figure 2 ) The RPA systolic and diastolic diameters, which were measured using the leading edge–to–leading edge convention, were defined as the maximum and the minimum vessel diameters, respectively. Finally, the ratio between the systolic and diastolic diameters was calculated and defined as PRIME.

From continuous-wave Doppler interrogation of the RV outflow tract, we calculated PR jet deceleration time as a slope of the line drawn between the peak velocity and the velocity at end of diastole. Moreover, the vena contracta (narrowest jet width) of the PR jet at the pulmonary annular level was also evaluated by means color Doppler; however, we succeeded in visualizing it in only 31 patients because of the lack of an identifiable PR jet in several patients with “free PR.”
The PR index was calculated as the ratio between PR duration and total diastolic time, as published by Li et al. PR duration was measured from its onset in early diastole to the end of the regurgitant Doppler signal. The total diastolic time was measured from the end of forward pulmonary flow (coinciding with the onset of the retrograde PR flow) to the beginning of the next forward pulmonary flow curve. In the control group, PRIME alone was evaluated. The study complied with the Declaration of Helsinki and was approved by ethics committee of our institution. Informed consent was obtained from all subjects or their parents.
MRI Protocol
A Signa CV/i 1.5-T scanner (GE Medical Systems; maximal gradient, 40 mT/m; slew rate, 150 mT/m/s) with a surface 4-channel cardiac phased-array coil was used. A comprehensive MRI evaluation was performed following a published protocol for patients with operated TOF. Related to the flow determination, a free-breathing gradient-echo velocity mapping electrocardiographically triggered sequence called PVC was used. PRF was calculated as (retrograde flow/anterograde flow) × 100, expressed as a percentage. In case of distortion of the pulmonary trunk, the through-plane flow was measured at the infundibular level just below the RV–pulmonary artery junction. The following acquisition parameters were used: velocity-encoded values, 150 to 450 cm/s, depending on the expected blood velocity; matrix size, 256 × 256; field of view, 28 to 34 cm; thickness, 5 to 6 mm; cardiac phase/cycle, 40; echo time ≈ 3.5 ms; repetition time ≈ 8 ms; flip angle, 15° to 20°; number of signals acquired, 1; views per second, 2. A PVC MRI sequence at the level of both branches of the pulmonary arteries to assess differential pulmonary perfusion was performed as well. All other acquisitions were performed during breath-holds. The PVC MRI sequences were transferred to a workstation (Advantage Windows 4.1; GE Medical Systems) and analyzed using commercially available software (CV Flow; MR Analytical Software Systems, Leiden, The Netherlands).
The MRI exam was completed using a contrast-enhanced (gadopentate dimeglumine 0.4 mL/kg) three-dimensional MR angiographic sequence for the anatomic evaluation of the pulmonary arteries. Eleven patients aged < 8 years were sedated using titrated propofol; in these patients, echocardiography was performed immediately after CV MRI in the awake room under the same conditions as during CV MRI.
Statistical Analysis
PRIME discrimination capacity was evaluated using receiver operating characteristic (ROC) analysis. PRIME performance was tested by the area under the ROC curve (AUC) using the Swets rule: if the AUC was >0.9, PRIME was considered to be highly accurate.
Maximizing PRIME sensitivity and specificity, PRIME cutoffs were identified to select subjects with mild PR (PRF < 15%), those with moderate PR (PRF ≥ 25%), and those with severe PR (PRF > 40%). Positive and negative predictive values were also determined.
Two observers (P.F. and L.A.) independently assessed PRIME in a random sample of 15 digitally recorded tracings. One investigator (L.A.) assessed intraobserver variability repeating measurements within a period of 4 weeks on the same tracings. The second round of intraobserver measures was blinded to the results obtained by the first observation. In 10 patients, PRIME was repeated in serial examinations. Interobserver, intraobserver, and interstudy variability of PRIME were evaluated using Bland-Altman analysis and Pitman’s test of difference in variance. Cronbach’s α coefficient was used to test interobserver, intraobserver, and interstudy reliability.
All analysis were performed on the entire population study, comparing two age subgroups (<18 and ≥18 years).
In 31 patients evaluated using all echocardiographic methods, the differences between their performance and PRF were evaluated using AUC comparisons and tested using χ 2 analysis. The standard error for the AUC curve was calculated using the method suggested by De Long et al.
PRIME performance by ROC analysis was also evaluated in the 6 subgroups of patients with conditions that could interfere with PRIME accuracy, as outlined above. However, because only a few cases were available, it was not possible to assess performance analysis for each subgroup, and, when feasible, results had low power. Nonparametric correlation analysis was then used to generate hypothesis of PRIME’s good performance in each patient subgroup. Therefore, Spearman’s rank correlation coefficient (ρ) was used to measure the correlation between PRF determined by PVC MRI with PRIME.
The choice of an adequate model for fitting PRF by PRIME was made by residual analysis of the observed data. From this analysis, nonlinearity of the data was observed (a scatterplot of the data showed the typical S shape), which enabled us to discard linear regression models in favor of nonlinear models (S-shaped curve). Among all the nonlinear models, the generalized logistic regression that presented the best goodness of fit ( R 2 ) was chosen. Therefore, to estimate PRF by PRIME, nonlinear regression using a 3-parameter logistic function was used:
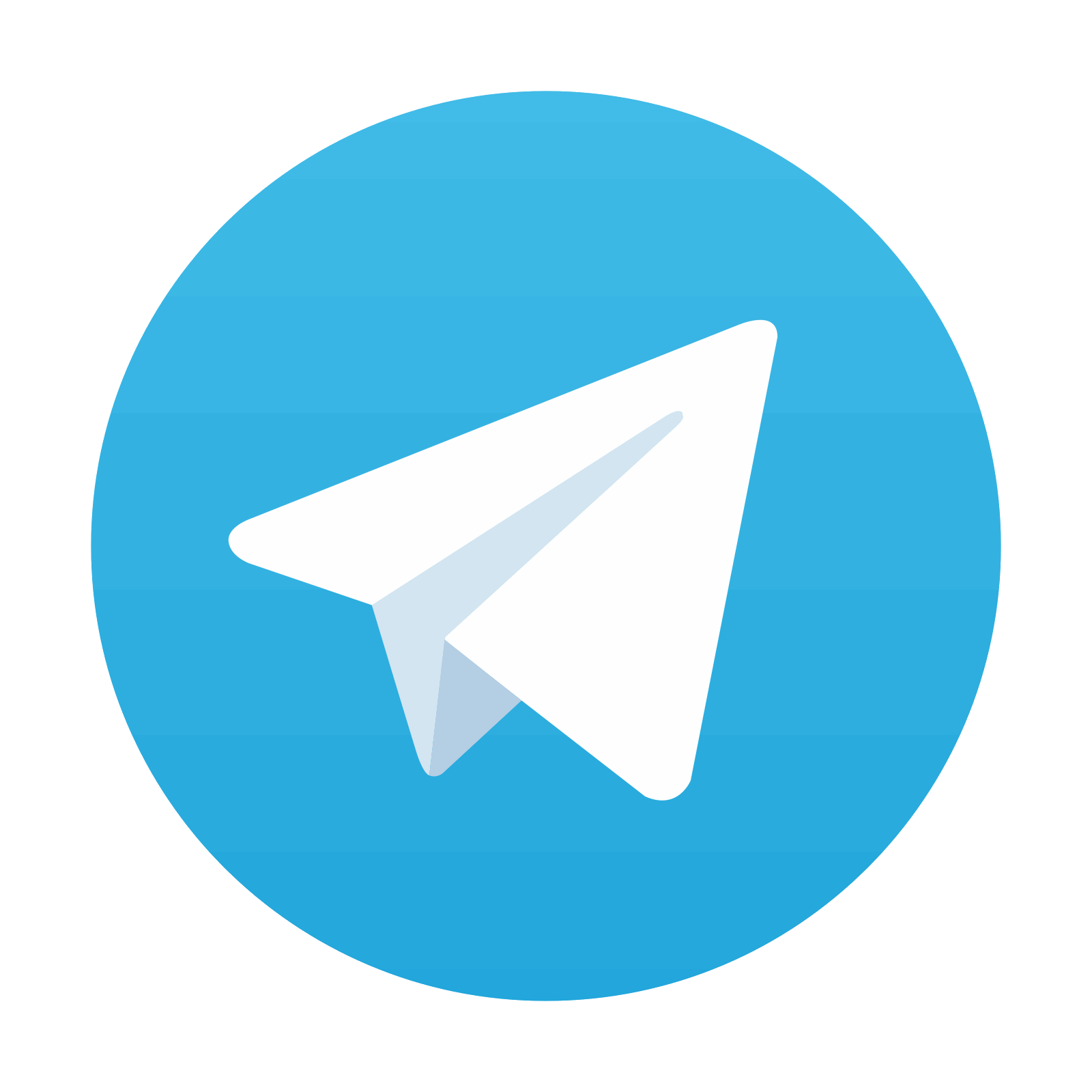
Stay updated, free articles. Join our Telegram channel

Full access? Get Clinical Tree
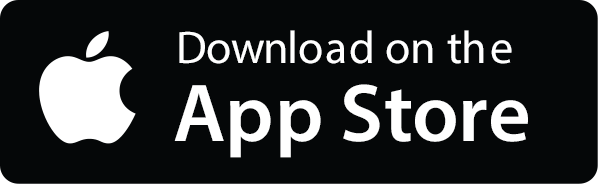
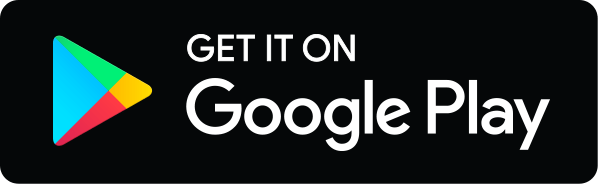