Fig. 7.1
Transesophageal echo images demonstrate a patent foramen ovale and atrial septal aneurysm bowing into the right atrium in a 38-year- old man presenting with cryptogenic stroke. (a) The agitated saline bubbles outline the tunnel shape of the PFO
Provocative Maneuvers
While TEE with color flow Doppler imaging is an accurate means for viewing an existing left-to-right shunt such as an atrial septal defect (ASD), a RLS through a PFO is often absent at rest. Visualizing a RLS through a PFO requires injection of a contrast agent to visualize the blood flow, associated with a method to provoke the opening of the PFO. The most commonly used contrast agent is agitated saline which produces microbubbles that are echogenic under ultrasound insonation. A frequently used provocative method is the Valsalva maneuver (expiratory effort against a closed glottis), or sometimes transient obstruction of venous return to the heart is induced by coughing or applying external abdominal pressure lasting for 5–10 seconds. The objective of the provocative maneuver is to increase venous return to the right atrium upon release of the Valsalva maneuver, which transiently raises right atrial pressure above left atrial pressure. This produces lifting of the septum primum flap from its contact with the septum secundum. This shifts the septum primum towards the left atrium with consequent passage of contrast from the right atrium through the PFO and into the left atrium. An adequate Valsalva maneuver is imperative to initially impede venous return, but when an increase in venous blood returns to the right atrium upon release of straining, the inter-atrial pressure gradient is transiently reversed, it transiently reverses the inter-atrial pressure gradient. A PFO is confirmed by passage of bubbles into the left atrium and visual confirmation of atrial septal opening (Figs. 7.2 and 7.3, Video 7.3). However, an ineffective Valsalva maneuver is not uncommon during a TEE since it may be difficult for the patient to perform under sedation and with a TEE probe placed in the esophagus.



Fig. 7.2
Transesophageal echo with positive bubble study through a patent foramen ovale

Fig. 7.3
Bubble study with Valsalva through a defect in the atrial septum consistent with an atrial septal defect (area of shunting inferior to the position expected for a patent foramen ovale)
Contrast Injection
The most common type of contrast agent used during echocardiography is a mixture of saline agitated with air. A standard protocol includes attaching two 10-mL syringes to a three-way stopcock (9 mL of saline combined with 0.5–1 mL of air) connected to an intravenous access line with one full and one empty syringe. The saline mixture is rapidly moved from the full syringe to the empty one 4–5 times for adequate agitation. The entire agitated saline mixture is then injected into the patient’s vein as a bolus. If the patient has a central line, even 5 mL of contrast may suffice considering the more direct venous access. However, the amount of contrast needed in patients with a central line also depends on the type and size of the central line with larger lines requiring more saline flush due to the presence of a larger anatomical dead space. Depending on the type of central line, the amount of saline required may vary from 2 to 15 mL of fluid.
Some studies have suggested that mixing a small amount of the patient’s blood with agitated saline may increase the sensitivity of the test [6, 7]. The proteins in blood allow smaller microbubbles to form per unit volume with increased surface area for echogenic reflections. The protein mixed bubbles last longer than when agitated saline alone is used. Other contrast agents that have been used include Echovist, hydroxyethylamidon, D-Galactose, Gelifundol, and various forms of gelatin based solutions [1, 8–11].
Criteria for Diagnosing an Intracardiac Right-to-Left Shunt
The cut-off for what constitutes a positive RLS, in terms of the number of microbubbles that pass and the timing of the shunt, varies among experts [12–18]. Most clinicians accept that a positive intracardiac RLS by echocardiographic imaging constitutes the passage of one or more microbubbles into the left atrium within three cardiac cycles after complete opacification of the right atrium after contrast injection and provocation; bubbles passing after three cardiac cycles are often attributed to a pulmonary shunt. However, published studies demonstrate that the cut-off for a positive intracardiac RLS used at different institutions ranges from 1 to 5 microbubbles within 1 to 5 cardiac cycles [3, 14, 19–21].
Site of Injection (Antecubital Versus Femoral Vein)
Contrast injection through the femoral vein increases the detection rate of PFO [22, 23]. Injection of contrast into the antecubital vein results in flow of contrast from the superior vena cava into the right atrium but away from the atrial septum, through the tricuspid valve and into the right ventricle. Especially with a persistent Eustachian valve, contrast injected into the femoral vein follows the path of oxygenated blood in the fetal circulation from the placenta, up through the inferior vena cava, and directly towards the atrial septum and the PFO, facilitating contrast entry into the left atrium.
However, most clinicians do not use femoral injections due to the impracticality of attaining femoral venous access for a bubble study. Femoral venous injections may be useful if studies are performed in the cardiac catheterization laboratory. Furthermore, femoral injections result in longer venous transit time with spontaneous destruction of microbubbles. The Center for Disease Control and Prevention guidelines [24] encourage use of upper versus lower extremity peripheral venous catheters to minimize the risk of infections and embolization.
Accuracy of TEE for the Detection of PFO
TEE allows visual interpretation of the PFO anatomy, the presence and direction of interatrial shunting, and assessment of the PFO post-closure. With current imaging advances, operators are able to evaluate the atrial septal anatomy by 3-D imaging. This may be especially helpful for measuring the size of the PFO or for assessing an implanted device after PFO closure (Figs. 7.4, 7.5, and 7.6, Videos 7.4 and 7.5). While it is considered the gold standard technique for the diagnosis of PFO, studies that have compared TEE to autopsy or intraoperative detection of PFO have demonstrated that the diagnosis of a PFO is sometimes missed by TEE [25, 26]. Other studies that have compared the accuracy of TEE in the detection of PFO to catheterization and/or surgery as the gold standard, have demonstrated a sensitivity of 91–100 % and accuracy of 88–97 % [21, 27]. A recent meta-analysis of prospective studies demonstrated the accuracy of contrast TEE in the detection of PFO compared to autopsy, intraoperative detection and/or catheterization as the gold standard; the sensitivity and specificity of TEE were 89 % and 91 % respectively [28]. This means that about 10 % of PFOs are not picked up if one depends solely on TEE for the diagnosis.




Fig. 7.4
3-D TEE image of the left atrial side after placement of a 25 mm Gore Helex septal occluder

Fig. 7.5
3-D TEE image of 25 mm Gore Helex septal occluder during agitated saline bubble study. Bubbles are visualized in the right atrium, but not on the left atrial side

Fig. 7.6
3-D TEE reconstruction of the PFO; the arrows point at the limbus of the septum secundum. The PFO canal is receding into the left atrium along the pathway of the septum primum (long arrow)
One of the advantages of TEE includes its ability to distinguish a PFO from an ASD, a determination that is important for the clinical management of the patient.
Limitations of TEE
While TEE is useful for the detection of PFO due to its high accuracy and ability to visualize the atrial septum, it also has several disadvantages. From the patient perspective, it is time consuming and uncomfortable. In addition to minor complications such as intra-procedural throat discomfort, gagging and post-procedural sore throat, on rare occasions esophageal bleeding or perforation may occur. TEE is relatively contraindicated in conditions that make the esophagus more susceptible to mucosal trauma during probe insertion and manipulation. TEE is often avoided in patients with a higher risk of esophageal perforation, including esophageal or gastric varices, Barrett’s esophagus, Zenker’s diverticulum, systemic amyloidosis, esophageal or pharyngeal carcinoma, strictures, Mallory-Weiss tears, patients with a serious risk of bleeding, and patients with recent radiation therapy of the esophageal area.
In addition, many stroke patients may have dysfunctional swallowing or poor cooperation making the test difficult to perform, affecting the test accuracy due to an inadequate Valsalva maneuver. False negative TEE tests may also be explained by ineffective Valsalva secondary to sedation or due to the presence of the TEE probe in the patient’s esophagus [29–31].
Transthoracic Echocardiography
Transthoracic echocardiography (TTE) is the most frequently used technique for diagnosing a PFO due its cost-effectiveness and easy availability. As opposed to TEE, on TTE the PFO is not visualized directly. The diagnosis of PFO is usually made by visualizing the apical four-chamber view of the heart during the intravenous introduction of agitated saline. A PFO is suspected when bubbles enter the left atrium from the right atrium (Fig. 7.7, Video 7.6).


Fig. 7.7
Positive bubble study on transthoracic echo
Due to the posterior location of the atria, TTE images of the septum often have low resolution. For better imaging, the subcostal (subxyphoid) four-chamber view can be utilized. Alternatively, the subxyphoid view of the inferior vena cava and hepatic vein can be used for PFO imaging. It allows better visualization of the inferior vena cava and superior vena cava draining into the right atrium, and the neighboring left atrium. However, when the patient performs a Valsalva maneuver, the inflating lungs and shifting diaphragm often lead to a brief loss of image, usually when the agitated saline has already been introduced and the bubbles are about to cross the septum.
Though cost-effective and frequently used for a PFO diagnosis in the outpatient setting, TTE has a low sensitivity. A recent meta-analysis of prospective studies comparing fundamental TTE to TEE as the reference determined a sensitivity of 46 % and specificity of 99 % for the diagnosis of intracardiac RLS [34]. In addition, the differentiation between intracardiac and intrapulmonary RLS can be difficult using the standard TTE technique.
TTE with Second Harmonic Imaging
Harmonic imaging is a commonly used echocardiographic technique that receives ultrasound signals at twice the transmitted frequency. With its ability to improve the quality of conventional two-dimensional transthoracic images, second harmonic imaging can be used to diagnose PFO with more accuracy compared to fundamental imaging with contrast TTE [9, 35].
In addition to significantly enhancing the image quality of the septum, harmonic imaging improves visualization of the bubbles as the second harmonic signal from microbubbles is stronger than the signal from the surrounding tissue and blood [34]. This allows better visualization of the PFO and discrimination of the source of the RLS (inter-atrial septum vs. pulmonary veins). In one recent study, TTE with second harmonic imaging increased the sensitivity of TEE above 90 %, with some studies demonstrating accuracies that are comparable to TEE [14, 37, 38]. The use of harmonic imaging with TTE has now become standard use in most centers.
Transcranial Doppler
Transcranial Doppler (TCD) is an alternative method for indirectly diagnosing PFO by assessing the presence of a RLS. It employs the functional assessment of the shunt using insonation of the middle cerebral arteries bilaterally during a venous injection bubble study and Valsalva maneuver. The optimal way of assessing for RLS is using the so-called “blind TCD”. Modern TCD machines are equipped with power M-mode software, which allows better imaging, and therefore, a more accurate quantification of shunting. Studies that have compared Power M-mode TCD to older TCD models have found that Power M-mode TCD has a higher sensitivity and accuracy [27]. A recent meta-analysis of 27 prospective studies that assessed the accuracy of TCD compared to TEE as the reference found TCD to have a sensitivity of 97 % and specificity of 93 % for the diagnosis of intracardiac RLS [39]. Spencer et al. demonstrated that the sensitivity of Power M-mode TCD may even be higher than that of TEE (98 % sensitivity using Power M-mode TCD compared to 91 % sensitivity using TEE) when PFO probing during catheterization was used as the reference [27] (Fig. 7.8).


Fig. 7.8
(a) Transcranial Doppler machine and setup. (b) Dr. Spencer demonstrates the technique for TCD that he developed. The patient is supine with a headband on and an intravenous line in the right antecubital fossa. The power M-mode equipment shows the headband, ultrasound transducers, and the arterial waveform on doppler (© Spencer Technologies, Inc. All Rights Reserved. Reprinted with Permission)
Evaluating the counts of saline bubbles reaching the middle cerebral arteries provides a relative assessment of the amount of shunting, which is a major benefit compared to TEE. The Spencer scale used for the grading of the shunt is logarithmic, with grade 3 being the cut-off for a positive study (Fig. 7.9). The choice of a cutoff is somewhat arbitrary, but is based on the observation that grade 3 TCD shunts are usually seen with small PFOs, whereas grade 1 or 2 TCD shunts are often found in people without a PFO. The shunt in these cases is due to transmission of some bubbles through the pulmonary circulation. While the sensitivity of power M-mode TCD surpasses that of TEE, this also results in some false positive studies.


Fig. 7.9
Trancranial Doppler grading with microembolic signals that measure degree of right-to-left shunting ranging from grade 1 (left) to grade 5 (right)
In addition, it is not always easy to distinguish a pulmonary shunt from a cardiac shunt with TCD. Authors describe the “late appearance” of bubbles (>3 cardiac cycles after the injection) which is often associated with a pulmonary shunt. However, large pulmonary arterio-venous malformations such as those seen in Hereditary Hemorrhagic Telangiectasia (HHT) do not follow this rule. They produce a pattern very similar to that of a PFO, with almost immediate “white-out” of the signal. Fortunately, the frequency of PFO is much higher than pulmonary arterial-venous malformations which only account for approximately 1 % of positive TCD’s in a population that is screened for PFO related conditions such as migraine or cryptogenic stroke. Therefore, the presence of a RLS by TCD is almost always due to a PFO.
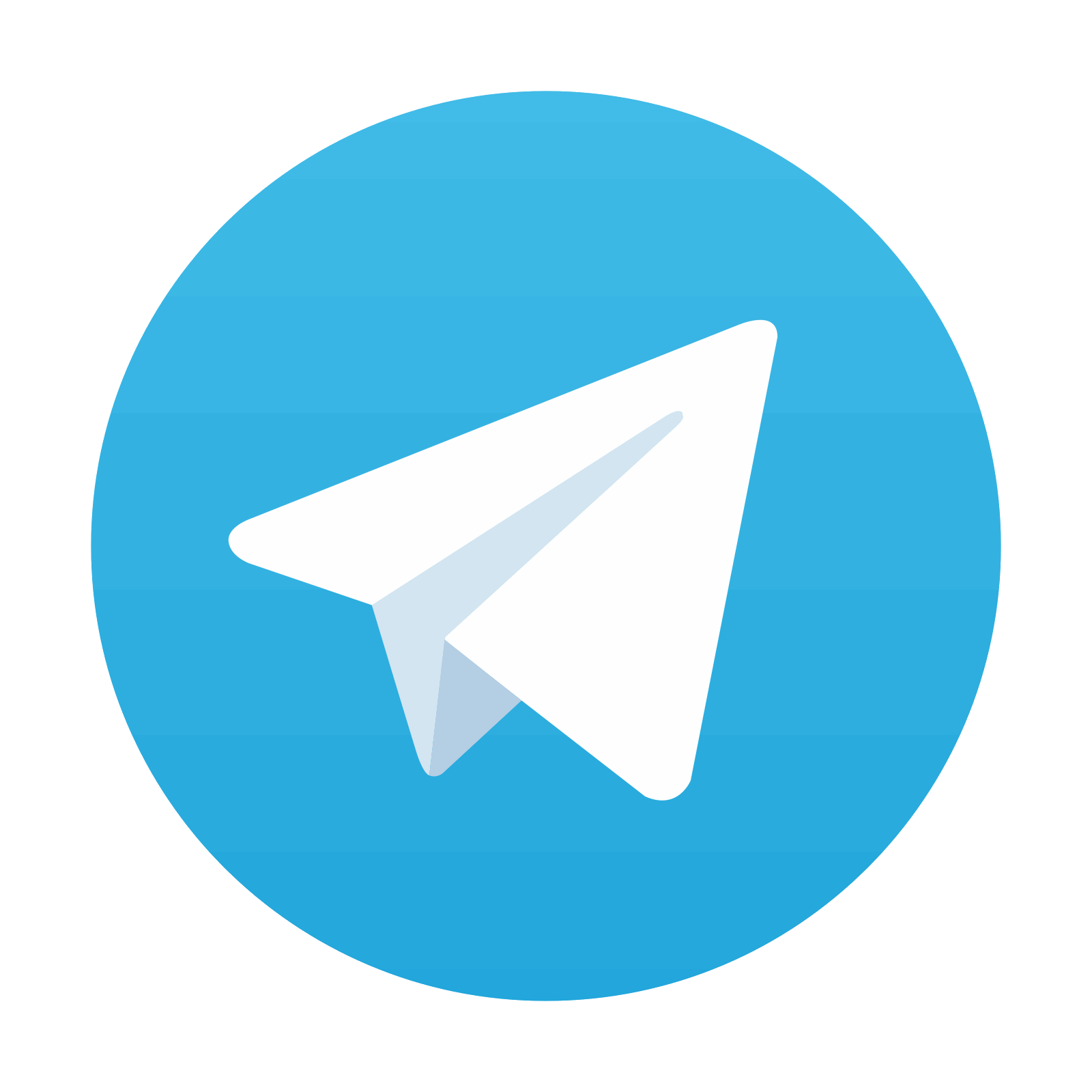
Stay updated, free articles. Join our Telegram channel

Full access? Get Clinical Tree
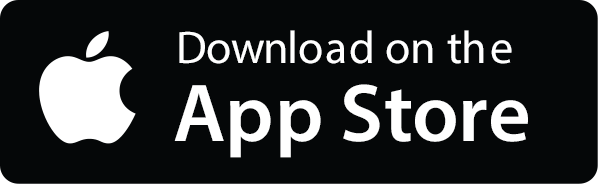
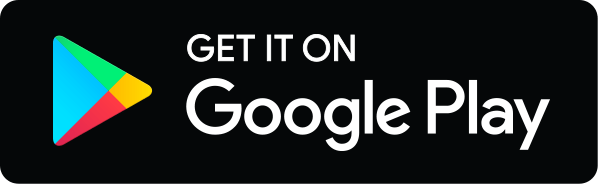