As mentioned in previous chapters in this unit, aging is the major risk factor for death from all age‐related adult chronic diseases. By 2034, for the first time in American history, it is projected that older adults will outnumber children. A major challenge associated with advanced adult age is the relationship between significant changes in many physiologic functions, the development of several impairments, the decline in overall functional capacity, the subsequent morbidity and mortality, and the resulting loss of independence that most older adults fear more than death (Frontera, 2017). Aging procedures in the human body are complex and yet not fully understood. At a biological level, aging is associated with the gradual accumulation of a wide variety of molecular and cellular damage. Over time, this accumulative damage increases the risk for many diseases while leading to general decline in several body functions. Ultimately, all these changes lead to death. FIGURE 9.1 The spectrum of intrinsic capacity from the perspectives of organ and systemic level and their clinical manifestations. In the first stages, there are changes in isolated organs that can still be reversed and do not impact function due to a high functional reserve. As the condition progresses, other organs start to deteriorate, due to the aging processes or to accumulation of chronic diseases that share similar mechanisms of damage. At this stage, frailty status appears; there is still enough functional reserve to maintain functional autonomy, although some deterioration in performance‐based tasks can be observed if carefully assessed. If the condition continues progressing, functional reserve is depleted and disability takes hold, with few chances for recovery. Source: (Angulo et al, 2020). Progressive cellular damage is expressed as a detrimental change in maximal organ function with different trajectories across organ systems (Khan, Singer, & Vaughan, 2017). Aging affects all organs and depends on individual’s genetics, developmental programming, and living or environmental conditions. All these factors combined determine the body’s ability to respond to stress, hence maximal organ functionality, which explains the influence that aging has on intrinsic capacity (Figure 9.1) and significant differences in age‐related responses between individuals (Khan et al., 2017). As mentioned in Chapter 7, intrinsic capacity comprises all the mental and physical capacities that a person can draw on and includes their ability to walk, think, see, hear, and remember. For example, because maximal physiologic capacities are greatly decline with age, the ability to perform any physical task at the same absolute level of energy expenditure or muscular force becomes limited (Frontera, 2017). Aging varies from organ‐to‐organ and person‐to‐person, and it appears to be an organ‐specific or organ‐differential resilience and vulnerability through aging (Figure 9.2). In the next paragraphs, the physiological changes observed in multiple organ systems through aging will be described. FIGURE 9.2 Relative rates of decline in maximal organ function across organ systems. Each organ system may carry a specific vulnerability to age. For example, the cardiovascular system appears to suffer biological aging more rapidly than the gastrointestinal system. Source: (Khan et al., 2017) Even though cardiovascular disease (CVD) is not necessarily related to advanced age, it is the leading cause of morbidity and mortality among older adults. The elderly are more likely to develop heart disease than younger adults due to an increased prevalence of age‐associated co‐morbidities such as hypertension, diabetes mellitus, and dyslipidemia. Thus, the incidence of coronary artery disease (CAD), valvular disease, rhythm disorders, and heart failure increases with age (Khan et al., 2017). Indeed, an aging cardiovascular system has an increased level of stiffness in the aorta and large vessels in general. This results in widened pulse pressure with raised systolic blood pressure (due to an increased resistance to blood ejection from the left ventricle [LV]) and lowered diastolic pressure (due to a steep pressure drop in diastole). Consequently, changes are an increased LV afterload, mass, wall thickness, and LV end‐diastolic volume. Further changes in calcium influx cause reduced LV compliance and delayed LV relaxation or decreased diastolic function as assessed by Doppler echocardiography parameters. Intrinsic heart rate also drops significantly due to both atrial pacemaker cellular loss (50% to 75% by the age of 50) and His bundle fibrosis. Fibrosis and calcification occur at the aortic valve cusp bases, annular valvular rings, and fibrous trigones. Finally, older individuals demonstrate decreased responsiveness to b‐adrenergic receptor stimulation in cardiomyocytes, decreased reactivity to baroreceptor and chemoreceptor output, and increased circulating catecholamines that results in reduced exercise tolerance and decreased cardiac output. These changes increase the heart’s vulnerability to the development of age‐related cardiovascular pathology such as hypertension, congestive heart failure, atrioventricular block, and aortic stenosis. Moreover, atherosclerosis is linked to premature biological aging with senescent cells identified in CAD plaques (Khan et al., 2017). Human lungs have the largest surface area in the body and represent one of the few consistently reliable physiological markers of aging. Lung cells face ongoing chemical, mechanical, biological, immunological, and xenobiotic stress over a lifetime. Aging causes progressive impairment of lung function in otherwise healthy individuals, marked by structural changes (Schneider et al., 2021). With advancing age, peak aerobic capacity falls with a greater than 20% decline per decade after age 70. Due to loss in lung‐tissue elasticity, there is a severe decrease in surface availability for gas exchange (increased anatomic dead space). Chest wall compliance decreases and dominates the increase in lung compliance, functional residual capacity decreases because of the fall in total respiratory system compliance. Forced vital capacity (FVC) declines 0.15 to 0.30 L per decade in nonsmoking men, and the forced expiratory volume in one‐second (FEV1) falls 0.20–0.30 L/s per decade with a steeper decline in the seventh and eighth decades. Residual volume increases by about 10%per decade due to an increased closing volume: the lung volume at which small airways in dependent lung zones begin to collapse during exhalation. Ventilation–perfusion mismatching increases with age, as airways in the better‐perfused dependent lung zones have an increased likelihood of closure during exhalation. Diffusion capacity decreases around 5% per decade, although hypoxemia does not typically develop. Further, advancing age is associated with a diminished central drive to the respiratory muscles in response to hypoxemia, hypercapnia, and mechanical load; exercise training may attenuate this hypo‐responsiveness. The above changes, when combined with decreased respiratory muscle strength and reduced efficacy of mucociliary clearance, result in higher risk of pneumonia to occur (Khan et al., 2017). One of the most well documented consequences of aging is the decline in the immune function. The aging immune system tends to under‐ or malfunction over time, a condition collectively described as immunosenescence, which leads to increased vulnerability to infection, malignancy, and autoimmunity (Khan et al., 2017; Aiello et al., 2019). While elderly individuals are known to be immunodeficient, they often do not respond efficiently to novel or previously encountered antigens. For example, individuals 70 years of age and older have increased vulnerability to influenza, a situation that is exacerbated by their poor response to vaccination (Montecino‐Rodriguez, Berent‐Maoz, & Dorshkind, 2013). The adaptive and innate immune systems both exhibit functional decline with aging, although innate immunity appears better preserved (Aiello et al., 2019). Regulatory T‐cells lose their suppressive function and accumulate in visceral adipose tissue (Khan et al., 2017). Age‐associated, chronic low‐grade inflammation, “inflamm‐aging”, is considered a pillar of the aging process and the most common pathogenetic mechanism of age‐related diseases as well as the worst prognostic factor for all causes of death (Aiello et al., 2019). Inflamm‐aging is a consequence of activated inflammation‐signaling networks, which are regulated by cytokines like nuclear factor κ‐light‐chain enhancer of activated B‐cells (NF‐κb) transcription factor and tumor necrosis factor (TNF)‐α as well as other inflammatory stimuli such as senescent cells, obesity, circulating mitochondrial DNA, gut microbiota, and diet (Aiello et al., 2019). The aging immune system is not efficient enough to respond to chronic stimuli sufficiently, especially toward stressors that occur later in life (Aiello et al., 2019). Chronic production of pro‐ inflammatory cytokines is suggested to be involved in muscle atrophy that correlates with lower mobility capabilities through muscle wasting and weight loss as well as the presence of malignancies and neurodegenerative diseases in older adults (Suzuki, 2019; Aiello et al., 2019). Cognitive decline is considered a hallmark of the aging nervous system and it is associated with increased memory loss and deteriorating mental ability, which in turn increases the risk for neurodegenerative diseases (Harman & Martín, 2020). Cognitive decline with aging is multifactorial and related to changes in structure as well as synaptic plasticity (Khan et al., 2017). The total brain volume declines at an approximate rate of 5% per decade after the age of 40 (Panagiotou, Michel, Meijer, & Deboer, 2021). Cerebral tissue atrophy and diminished cerebral perfusion result in significant white‐matter loss, but neuronal dropout varies by brain region with little or no loss in some regions (Khan et al., 2017). Notably, white‐matter volume changes in aging are nonlinear with more rapid changes as age advances in humans, whereas gray matter has a smaller and more linear decrease. In addition to the prefrontal cortex, one of the most affected areas in healthy aging, the hippocampus is another greatly affected area. Advanced age is associated with widespread thinning of the cerebral cortex (Panagiotou et al., 2021). In addition, dopaminergic signaling progressively decreases via the D2 receptor. Functional MRI studies demonstrate less coordinated activation in brain regions focused on higher‐order cognitive functions, which suggests a global loss of integrative function with aging. Gene expression profiling studies show that significant changes in synaptic gene expression contribute to altered higher‐order integration (Khan et al., 2017; Panagiotou et al., 2021). These alterations in synaptic plasticity and loss as well as impaired neurogenesis may predispose aged individuals to neurodegenerative disorders such as Alzheimer’s and Parkinson’s disease (Khan et al., 2017). Alterations in structure and function of the musculoskeletal system can lead to functional loss leading to impairment and disability. If left untreated, several life‐threatening complications can occur, such as falls and bone fractures associated with muscle weakness (Frontera, 2017). The musculoskeletal system is a large group of tissues including muscles, bones, tendons, ligaments, and cartilage, which work simultaneously to ignite and maintain physical function and undergoes multiple changes with aging (Khan et al., 2017). In older adults, type II muscle fibers or fast twitch fibers, tend to shrink in size and number. This results in lower strength and muscle tone, which lessens contractile force and therefore, limits mobility (Khan et al., 2017; Walston, 2012; Eckstrom, Neukam, Kalin, & Wright, 2020). Longitudinal studies demonstrate an up to 1.5% reduction in muscle strength per year, mainly in the lower (knee extensors and flexors), rather than the upper, limbs (Frontera, 2017). Age‐related loss of muscle mass (sarcopenia) occurs involuntarily along with qualitative changes in muscle characterized by fat and connective tissue infiltration, increased levels of lipofuscin, and the accumulation of other cellular waste products (Walston, 2012; Eckstrom et al., 2020). Findings from the AGES‐Reykjavik study suggest that muscle composition may be associated with mortality risk (Khan et al., 2017). Moreover, sarcopenia is also associated with acute and chronic disease states, increased insulin resistance, fatigue, and risk of falling (Eckstrom et al., 2020). Sarcopenia (Greek “sarx” or flesh + “penia” or loss) is a highly common geriatric syndrome characterized by the involuntary loss of skeletal mass and strength that affects millions of older adults around the world (Walston, 2012). It is also considered a key component for the development of frailty in older adults (Clegg, Young, Iliffe, Rikkert, & Rockwood, 2013). In its revised definition, the European Working Group on Sarcopenia in Older People (EWGSOP2) uses low muscle strength as the primary parameter of sarcopenia; muscle strength is the most reliable measure of muscle function. Specifically, sarcopenia is probable when low muscle strength is detected. A diagnosis is confirmed by the presence of low muscle quantity or quality. When low muscle strength, low muscle quantity/quality, and low physical performance are all detected, sarcopenia is considered severe (Tables 9.1 and 9.2 provide the operational definition of sarcopenia and the most commonly used tools to assess the definition’s criteria in clinical and research environments, respectively) (Cruz‐Jentoft et al., 2019). Untreated or underdiagnosed sarcopenia increases the risk of falls and bone fractures and impairs the ability to execute activities of daily living (ADLs). It also correlates with cardiac and respiratory disease, cognitive deterioration, ambulatory disorders, and an overall worse quality of life (Cruz‐Jentoft et al., 2019). It is one of the most important causes of functional deterioration and loss of independence in older adults, since muscle mass accounts for up to 60% of body mass (Walston, 2012). On average, 5% to 13% of older persons over 60‐years old have low muscle mass, increasing up to 50% in people over the age of 80 (Morley, Anker, & von Haehling, 2014; Meier & Lee, 2020). The prevalence differs between organizations due to the lack of a universal definition (Meier & Lee, 2020). In 2016, sarcopenia was recognized as an independent condition by the ICD‐10‐CM Code, meaning that it is recognized as a pathological entity of its own, underlining the importance of its early diagnosis and treatment (Anker, Morley, & von Haehling, 2016). The causes of sarcopenia are multifactorial and include increased inflammation rates, age‐related molecular changes (i.e., mitochondrial function and TGF‐β pathway downregulation as well as apoptosis activation), a loss of anabolic hormones and insulin resistance, decreased size, lower total number and firing rates from motor neurons, lower activity levels, and lower energy intake (Walston, 2012). Table 9.1 Concensus by the European Working Group on Sarcopenia in Older People (EWGSOP2) ‐ 2018 operational definition of sarcopenia. Source: (Cruz‐Jentoft et al., 2019). Table 9.2 Choosing tools for sarcopenia case finding and for measurement of muscle strength, muscle mass and physical performance in clinical practice and in research. Source: (Cruz‐Jentoft et al., 2019 / with permission of Oxford University Press). SARC‐F = Strength, assistance with walking, rising from a chair, climbing stairs, and falls. 1 Sometimes divided by height2 or body‐mass index (BMI) to adjust for body size. Frailty is a clinically recognizable condition accompanied by increased vulnerability. It is a result of the aging‐related decline in maintenance and functionality across several physiological systems that define the ability to deal with everyday struggles as well as trauma or stress due to chronic disease (Figure 9.3) (Xue, 2011; Clegg et al., 2013). Its prevalence increases with age and reaches 9.9% in community‐based older adult populations. It is estimated to be more frequent in women than in men (Sabine, 2020; Xue, 2011; Clegg et al., 2013). Frailty often leads to reduced autonomy and independence in older adults (Nascimento et al., 2019) and increases the chances of falls, disability, and acute confusion (Clegg et al., 2013). For a diagnosis of frailty, three of five phenotypic criteria should be met: compromised energetics, low grip strength, exhaustion, slowed walking speed, low physical activity, and unintentional weight loss, which are known as the Fried phenotype score (FPS) (Xue, 2011). Detailed cutoff points for each parameter are suggested but are not the result of an overall consensus (Cederholm et al., 2017). Apart from the FPS, the frailty index is also used to screen, classify, and assess improvements made after interventions in populations that are at risk of or experience frailty (Cesari, Gambassi, Van Kan, & Vellas, 2014). FIGURE 9.3 Vulnerability of frail elderly people to a sudden change in health status after a minor illness. Frailty is a long‐established clinical expression that implies concern about an elderly person’s vulnerability and outlook. The green line represents a fit elderly individual who, after a minor stressor event such as an infection, has a small deterioration in function and then returns to homeostasis. The red line represents a frail elderly individual who, after a similar stressor event, undergoes a larger deterioration, which may manifest as functional dependency and who does not return to baseline homeostasis. The horizontal dashed line represents the cutoff between dependent and independent. Source: (Clegg et al., 2013 / with permission of Elsevier). Despite their common etiologies, frailty differs from sarcopenia. The main differences lie in the loss of muscle performance, hence the amelioration of a person’s functional capabilities, which is a step beyond the loss of muscle mass, strength, and power alone (Nascimento et al., 2019). The presence of frailty is associated with several changes:
CHAPTER 9
Physiological Changes in Multiple Organ Systems Through Aging: Measuring and Monitoring Aging
INTRODUCTION
PHYSIOLOGICAL CHANGES IN MULTIPLE ORGAN SYSTEMS THROUGH AGING
CARDIOVASCULAR
PULMONARY
IMMUNE SYSTEM
NEUROLOGIC FUNCTION
MUSCULOSKELETAL SYSTEM
Sarcopenia
Probable sarcopenia is identified by Criterion 1.
Diagnosis is confirmed by additional documentation of Criterion 2.
If Criteria 1, 2 and 3 are all met, sarcopenia is considered severe.
Variable
Clinical practice
Research studies
Case finding
SARC‐F questionnaire
Ishii screening tool
SARC‐F
Skeletal muscle strength
Grip strength
Chair stand test (chair rise test)
Grip strength
Chair stand test (5‐times sit‐to‐stand)
Skeletal muscle mass or Skeletal muscle quality
Appendicular skeletal muscle mass (ASMM) by Dual‐energy X‐ray absorptiometry (DXA)*
ASMM by DXA
Whole‐body skeletal muscle mass (SMM) or ASMM predicted by Bioelectrical impedance analysis (BIA)1
Whole‐body SMM or ASMM by Magnetic Resonance Imaging (MRI, total body protocol)
Lumbar muscle cross‐sectional area by CT or MRI
Mid‐thigh muscle cross‐sectional area by Computed Tomography (CT) or MRI
Lumbar muscle cross‐sectional area by CT or MRI
Muscle quality by mid‐thigh or total body muscle quality by muscle biopsy, CT, MRI or Magnetic resonance Spectroscopy (MRS)
Physical performance
Gait speed
Gait speed
Short physical performance battery (SPPB)
Timed‐up‐and‐go test (TUG) 400‐meter walk or long‐distance corridor walk (400‐m walk)
TUG400‐m walk
Frailty
Stay updated, free articles. Join our Telegram channel

Full access? Get Clinical Tree
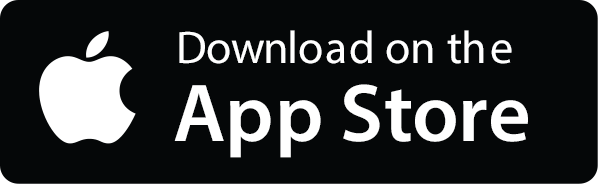
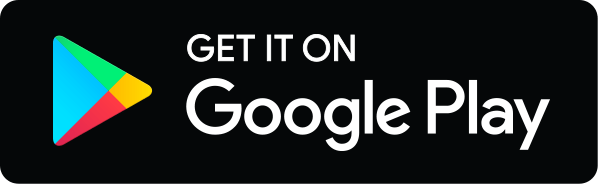