Acquired Heart Disease
Structure of Coronary Arteries
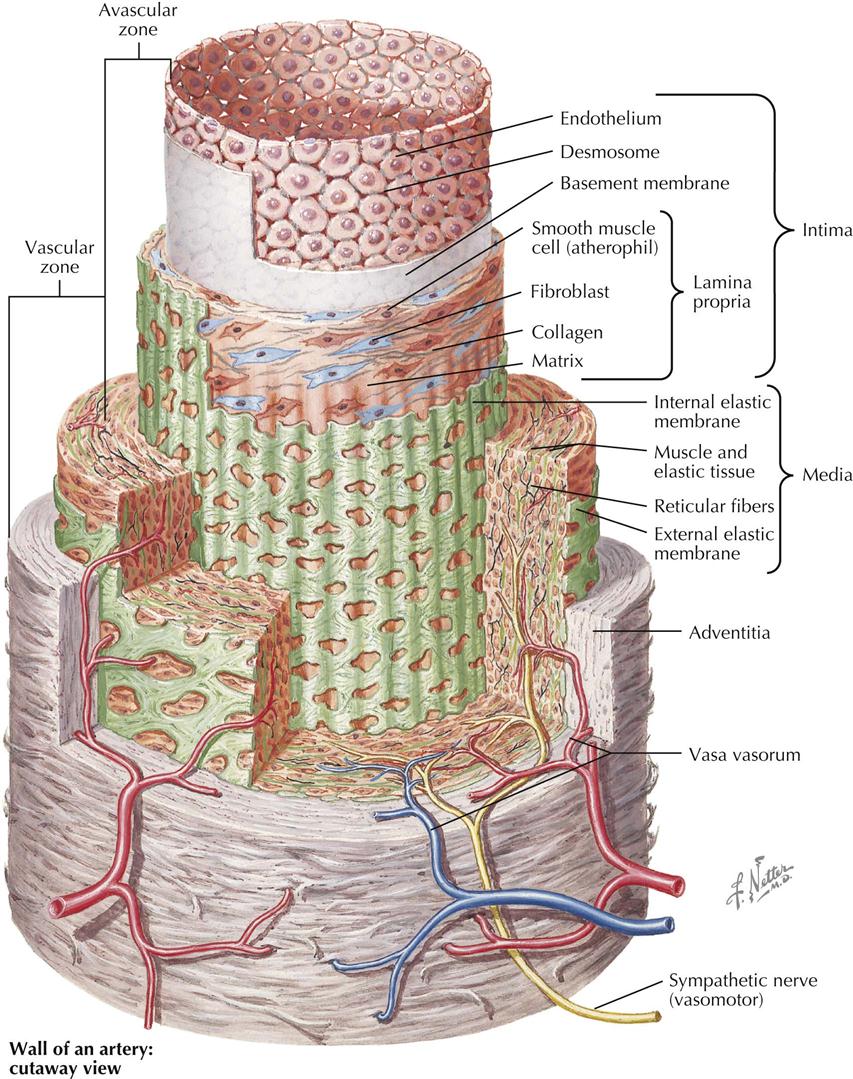
The coronary arteries are susceptible to atherosclerosis as well as its complications, particularly intravascular thrombosis, often resulting in myocardial infarction. Atherosclerosis is a form of arteriosclerosis characterized by initial involvement of the inner layer of the arterial wall, or intima. The intimal localization of early atheromas helps to differentiate the atherosclerosis type from other forms of arteriosclerosis, such as Mönckeberg’s medial sclerosis or periarteritis nodosa, that primarily involve the muscular or adventitial layers.
Recent histochemical, organ culture, and electron microscopy studies found further evidence of the complexity of the arterial wall. As Plate 6-1 shows, a medium-sized muscular artery, such as a main coronary vessel, consists of a series of concentric tubes or coaxial coats of differentiated cellular and extracellular components in three layers: tunica intima or intima, tunica media or muscular, and tunica adventitia. In the intima the innermost cell layer in direct contact with the bloodstream consists of a sheet of polygonal endothelial cells, usually less than 1 micron (µ) thick, except at the site of the cell nucleus, and elongated in a direction parallel to the vessel’s axis. Many of these endothelial cells have pinocytotic vesicles of caveolae in their cytoplasm, abundant mitochondria, well-developed granular endoplasmic reticulum, and Golgi complexes. Microscopically, the areas of contact between endothelial cells vary from simple mutual contact of the cell membranes to well-defined intercellular bridges, or desmosomes, corresponding to the so-called intercellular cement lines described in earlier light microscopy studies. A distinct basement membrane separates these endothelial cells from the subendothelial space, which varies in thickness according to age of the patient as well as size of the artery. In fetal life and shortly after birth the endothelium in the coronary arteries lies in direct contact with the internal elastic membrane, or lamina, without the subendothelial space, which appears by the end of the first decade of life.
In adulthood the intima consists of a matrix of ground substance containing small amounts of acid mucopolysaccharides and elastic and collagen fibers separating scattered intimal cells, or intimacytes. Because of morphologic and cytochemical difficulties in classifying intimacytes, their response to in vitro incorporation of serum lipids is used to identify them. Some intimacytes, or atherophils, incorporate lipid rapidly and show ultrastructural characteristics of modified smooth muscle cells, with typical bundles of myofilaments in their cytoplasm, pinocytotic vesicles, and parts of limiting basement membrane on the cell surface. On the other hand, cells called fibrophils are spindle shaped and have fingerlike cytoplasmic projections, with no basement membrane and few pinocytotic vesicles. Occasionally, large mononuclear ovoid cells appear, containing cytoplasmic inclusions with “single-unit” acid phosphatase–positive granules or lysosomes resembling macrophages.
The lamina propria is separated from the underlying smooth muscle cells of the media usually by a well-developed internal elastic membrane of tenacious matrix containing fibrils about 500 angstroms (Å) in diameter with abundant fenestrations. This internal elastic membrane is usually wavy in cross sections, and the fenestrations are oval or round openings extending across its surface. The underlying tunica media is characterized by concentric layers of smooth muscle cells 10 to 25 µ in length and oriented transversely to the artery’s main axis. Individual smooth muscle cells are surrounded by a network of collagenous and elastic fibers, which continue without transition into both internal and external elastic membranes. The external elastic membrane separates the media from the adventitia and is characterized by the presence of loosely packed collagen and elastic fibers. Small blood vessels or vasa vasorum are also found, as well as both sympathetic and parasympathetic nerve fibers from the autonomic nervous system.
These morphologic and functional characteristics of the coronary arteries are modified early in the presence of the intimal changes characteristic of atherosclerosis. The most important clinical difference between coronary and aortic atherosclerosis is the high incidence of acute thrombosis in coronary vessels, with infarction resulting in irreversible damage to the underlying myocardium.
Pathogenesis of Atherosclerosis
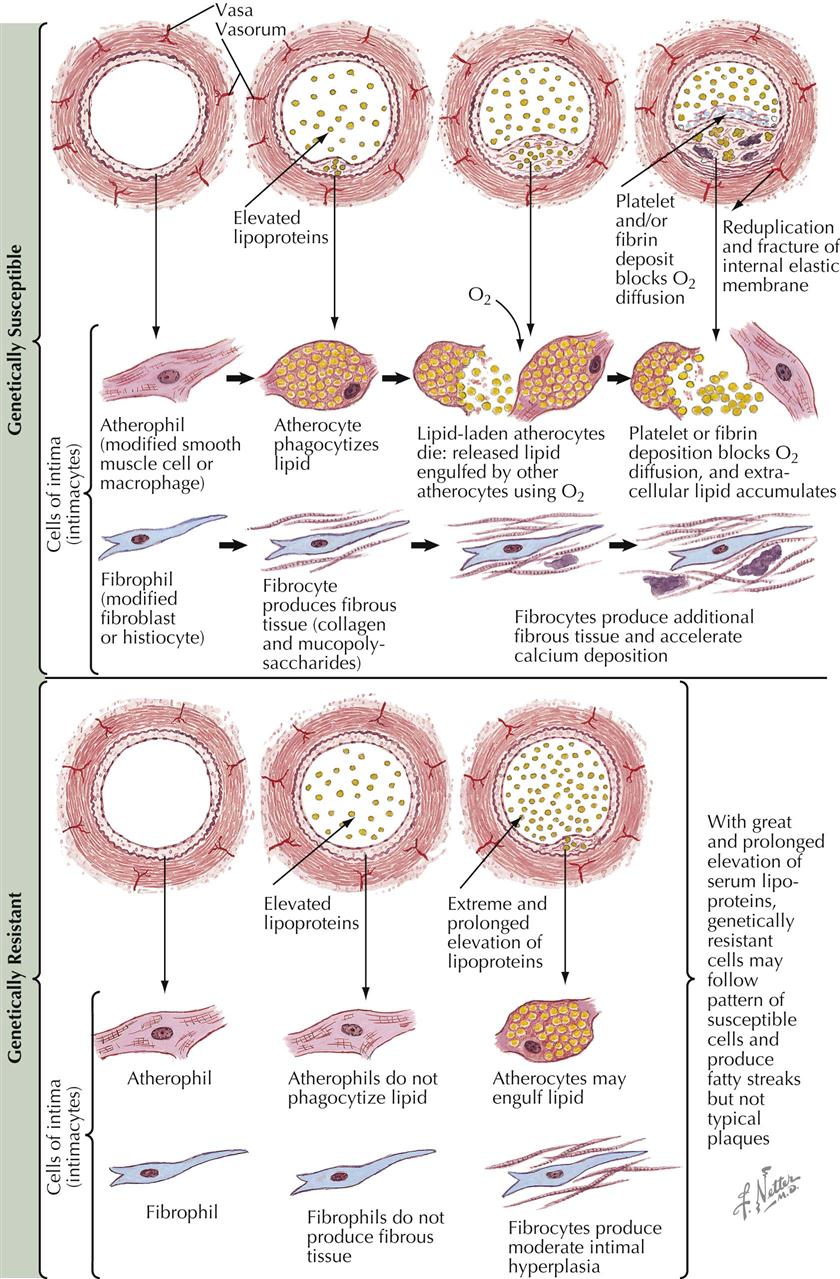
Histologic and functional characteristics of the intimal lining of the arteries help in understanding the histogenesis of spontaneous atheromas, often initiated shortly after birth, particularly the presence of different cell types in a ground substance matrix and the absence of a direct blood supply. The well-developed atherosclerotic plaque resulting from inflammatory and reparative processes is a complex lesion containing extracellular deposits of calcium salts, blood components, cholesterol crystals, and acid mucopolysaccharides. The initial changes, however, seem to occur at the cellular level, often accompanied by an abnormal intracellular storage of lipids, particularly cholesterol esters, fatty acids, and lipoprotein complexes. These findings on microscopy have strengthened the thesis that lipid infiltration from the bloodstream may be a significant factor in the growth of the atheromatous plaque. Furthermore, recognition by cytochemical techniques makes lipids helpful indicators of abnormal cell behavior, independent of their role in the etiology of atheroma.
Because of the frequency and severity of atherosclerosis, short-term organ culture techniques for the isolation of arterial intimal cells (intimacytes) identify susceptible cell populations, based on their response to incorporation of homologous serum lipids in vitro. Intimacytes from arteries, with and without histologic evidence of atherosclerosis, show two types of atherophils: genetically highly susceptible cells and genetically resistant cells to intracellular lipid accumulation.
In the presence of increased perfusion rates of plasma lipoproteins caused by local hemodynamic changes, hypertension, elevated serum lipids, or changes in endothelial surface permeability (trauma, fibrin deposition, platelet aggregation), genetically susceptible atherophils will be transformed into lipid-laden atherocytes. The secondary release of intracellular lipids from these cells will induce surrounding atherophils to incorporate them, creating a self-feeding process with cytologic changes that increase metabolic requirements, including oxygen consumption rates, which result in increased permeability of the cell membrane to lipids if not met. These events set up a cycle favoring further expansion of focal atherosclerotic changes. Collagen fibers are laid down by fibrophils, which are then transformed into fibrocytes surrounded by acid mucopolysaccharide deposits. This eventually results in a characteristic intimal hyperplasia after ground substance changes. These histologic lesions are self-perpetuating, resulting in the replacement of intimacytes by an acellular area of arterial intima that stimulates further surface involvement of the vascular wall in this interplay among deposition, inflammatory response, and scarring, with the eventual production of a typical atherosclerotic plaque.
In contrast, genetically resistant atherophils, even in the presence of elevated serum-lipid levels, do not respond (or respond minimally) to an increased perfusion of plasma lipids. If some atherocytes appear, they are few in number, and only superficial fatty streaks are developed, resulting in sparse intimal elevations with few fibrophils and no well-organized plaque. This type of cytologic response also seems less susceptible to the clinical complications of atherosclerosis, particularly thrombosis or rupture, because of the limited involvement of the vascular wall.
This approach to the pathogenesis of atherosclerosis emphasizes the clinical significance of the earliest possible identification of patients whose arteries are susceptible to atheroma, to modify or prevent environmental conditions that favor the acceleration of atherogenesis in the arterial intima cells. Local factors are also important in identification of the lesions, suggesting possible therapeutic approaches for their arrest or inhibition.
Risk Factors in Etiology of Atherosclerosis
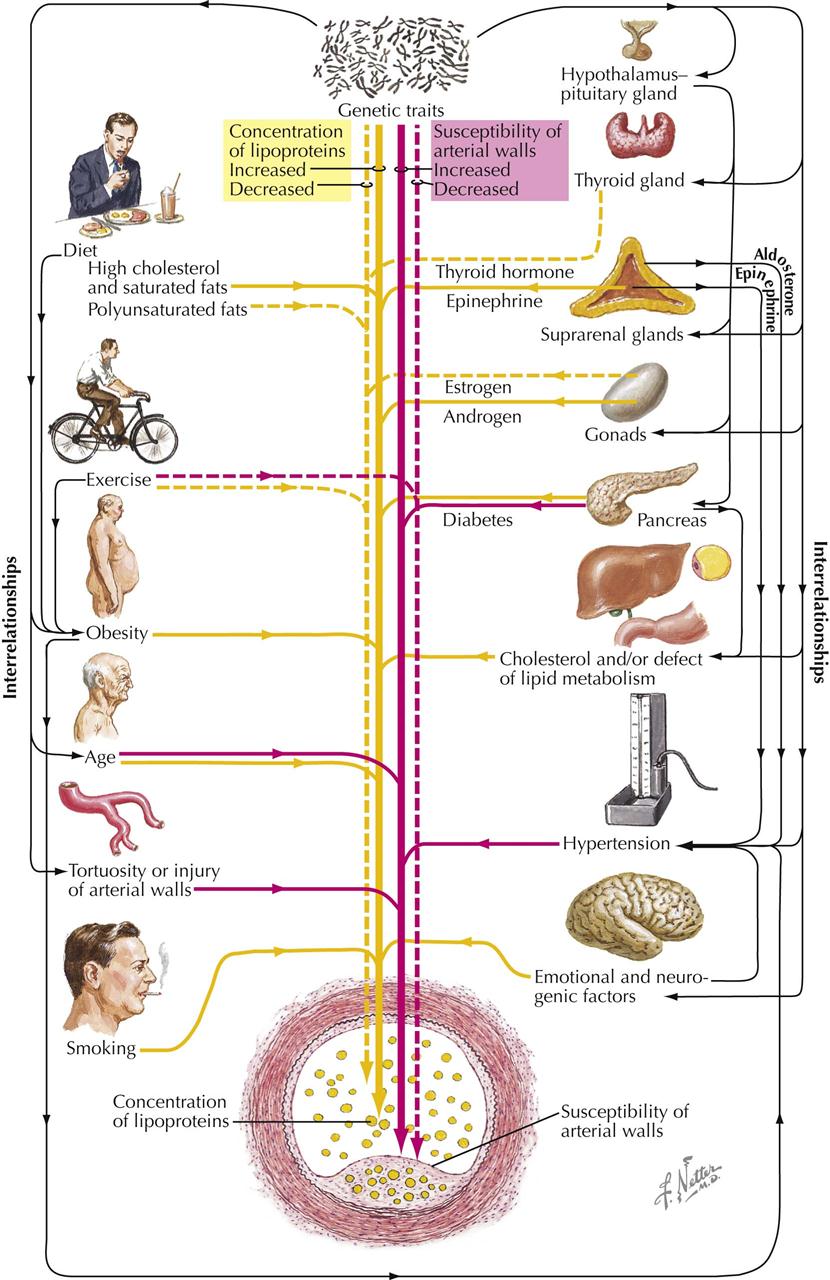
In terms of mortality, the most serious problem facing more developed countries is atherosclerosis of the cardiac and cerebral blood vessels. There is no single cause of atherosclerosis, which is often labeled a “multifaceted disease.” Atherosclerosis reflects the culmination of many factors acting over a lifetime and usually becomes clinically manifest only when angina pectoris, sudden cardiac death, myocardial infarction (MI), transient ischemic attack (TIA), or cerebrovascular accident (CVA, stroke) occurs. Heart failure is usually caused by MI.
Patients with a family history of premature manifestations of atherosclerosis may be at high risk for development of atherosclerotic disease. Older age, male gender, hypertension, diabetes, obesity, and tobacco smoking are associated with coronary atherosclerosis. Lack of exercise may interact with other clinical factors, and an exercise program (e.g., walking) is known to diminish symptoms in patients with known clinical manifestations of atherosclerosis. Excessive saturated fat in the diet is also involved in atherogenesis. High-fat diets increase blood lipid levels, particularly low-density lipoprotein (LDL) cholesterol. Elevated triglycerides and low levels of high-density lipoprotein (HDL) are also implicated in the etiology of atherosclerosis. Elevated serum uric acid may also contribute to atherogenesis, although further study is required. Unsaturated fatty acids with two or more double bonds help reduce cholesterol blood levels when their relationship to saturated fatty acids is increased.
Current concepts of atherogenicity are complex and involve monocytes, platelets, macrophages, foam cells, endothelial cells, smooth muscle cells, oxidized LDL, and many other subcellular substances. Interested readers should refer to current literature and textbooks on the subject. In general, the physician or clinician can profile a patient potentially prone to atherosclerosis, such as a hypertensive man who smokes cigarettes, exercises little, and has elevated blood cholesterol and triglyceride levels, low HDL levels, and abnormal glucose metabolism. Currently, in addition to diet and exercise, drugs used to treat lipid abnormalities include HMG CoA reductase inhibitors (statins), nicotinic acid preparations, ezetimibe, fibric acid derivatives, and bile acid sequestrants.
Pathologic Changes in Coronary Artery Disease
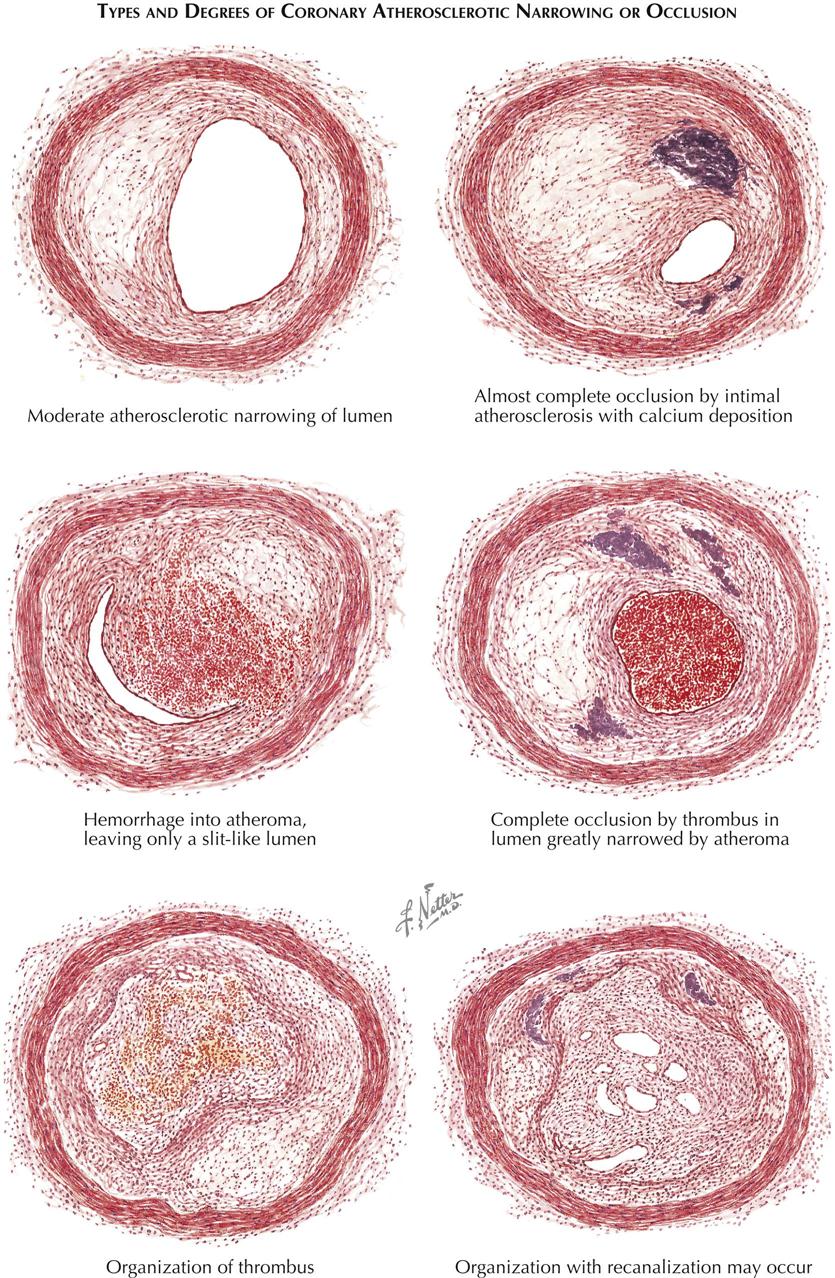
The earliest recognizable atheromatous changes in blood vessels are accumulation of aggregates of lipid-laden macrophages in the intima. The appearance of this lesion is similar to that seen in many experimental vascular lesions. Another apparently early phenomenon, difficult to correlate and possibly unrelated, is a subintimal deposit of collagen that often is primitive and thus rich in acid mucopolysaccharides. Lesions of this type shadow atherosclerosis wherever it occurs. The relationship of this vascular lesion to the type seen in Asian peoples has not been elucidated, but this disorder does occur in Asians. A further complication of this lesion is the deposition of fibrin on or within its superficial layers.
As more lipid accumulates, an atheromatous plaque with many constituents is formed. This plaque may involve only a segmental portion of the artery or its entire circumference. When the macrophages die, their lipid is released and becomes an inflammatory irritant. Fibrin accumulates, as do other blood constituents; calcium deposits form; and fibroblasts proliferate. Stenotic lesions may accumulate more surface material with deposited layers of thrombus. These lesions regress with lipid-lowering agents and antiplatelet therapy.
Even a segmental atheroma can cause atrophy of the underlying arterial wall, and the concentric type seems associated with major destruction of the lamina and parts of the media. Such changes appear secondary to interference with the nutrients permeating the intima. The stages of atheroma reveal another major feature in the lesion’s development: an inability to clear blood constituents that have permeated the media. This dynamic event is demonstrated by perfusing isolated segments of living canine arteries and observing the quantity of lipid in the vasa vasorum of the adventitia.
Hemorrhage into the lesion can result from surface blood dissecting into the plaque or from vasa vasorum bleeding. Thrombosis may occlude the artery; if the person survives, organization of the fibrin clot can progress and occasionally recanalize the artery. However, the ability of these small channels to supply any appreciable volume of blood beyond the area of obstruction is insufficient in most patients to revascularize the downstream myocardium. Rarely, an occluding thrombus completely disappears.
In addition to atheromatous and thrombus blockade of the coronary arteries, emboli can obstruct an otherwise normal coronary artery. Such emboli may consist of thrombi, valvular calcification, pieces of tumor, and occasionally even small foreign bodies. Various types of aortitis can involve the proximal vessels; syphilis of the aortic wall can occlude the ostia. The atheroma is the main disorder, however, because of its distribution. The lesions closest to the aorta (proximal) are the plaques most likely to impede flow to the cardiac microcirculation.
End-Organ Damage by Vascular Disease
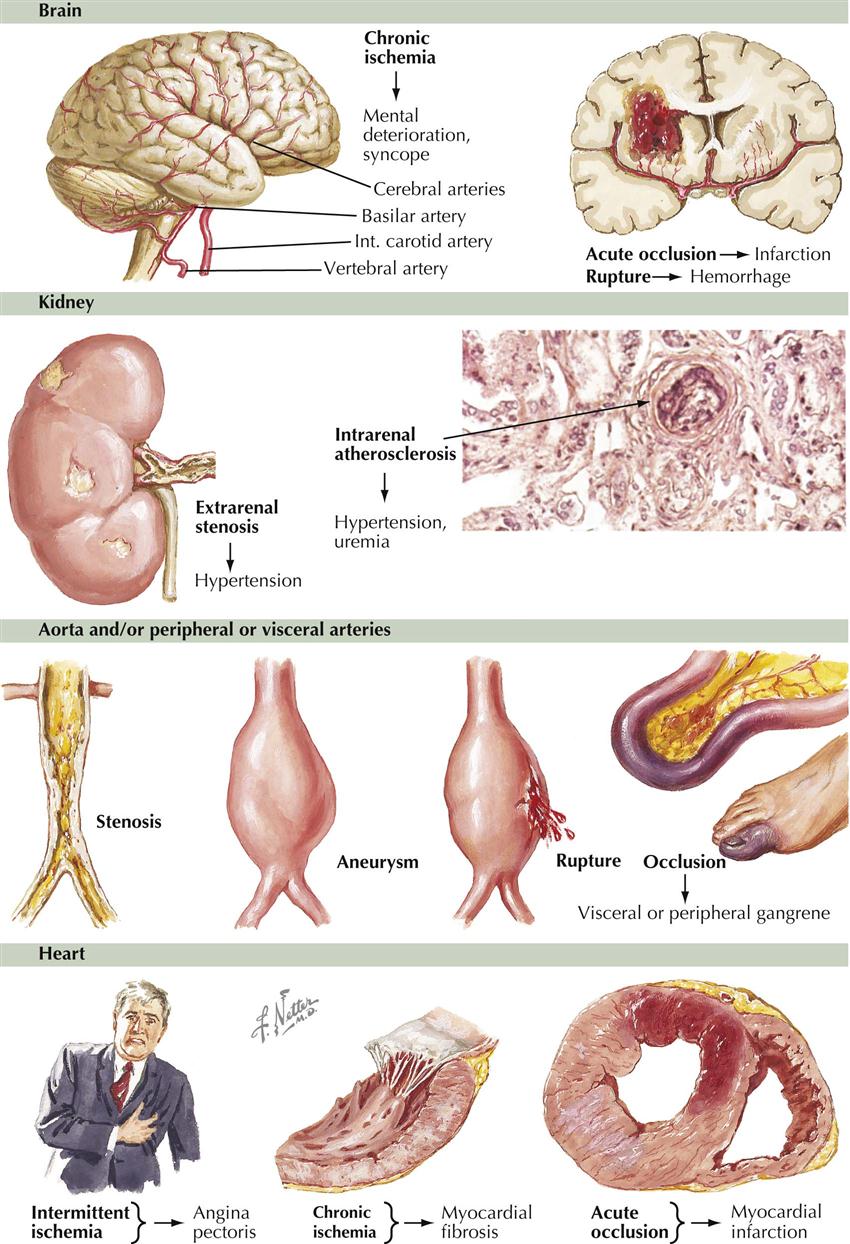
The cerebral circulation is particularly vulnerable in hypertensive patients. Stroke is the major cause of death. Hypertensive vascular disease is usually a combination of hypertension and atherosclerosis, the latter accelerated by elevated blood pressure. Infarction of the brain is more common than subarachnoid bleeding. Bleeding results from rupture of microaneurysms of the small arteries or from trauma. The coronary vessels are next most vulnerable. Angina pectoris and MI are common results of accelerated atherosclerosis, necessitating concurrent treatment of the hypertension and atherosclerosis. Many patients reduce their high blood pressure and return to normal levels, only to have an MI or die from complications of atherosclerosis. The results of the increased rate of atherogenesis are also seen in other large vessels besides the coronary arteries. Thus, aneurysms of the aorta and renal arteries are common, leading to distal embolization, dissection, and possible rupture. Reducing blood pressure has a beneficial action on these lesions. Drugs, surgery, and interventions are now available to treat the clinical effects of both hypertension and atherosclerosis, although the goal should be prevention.
Unstable Plaque Formation
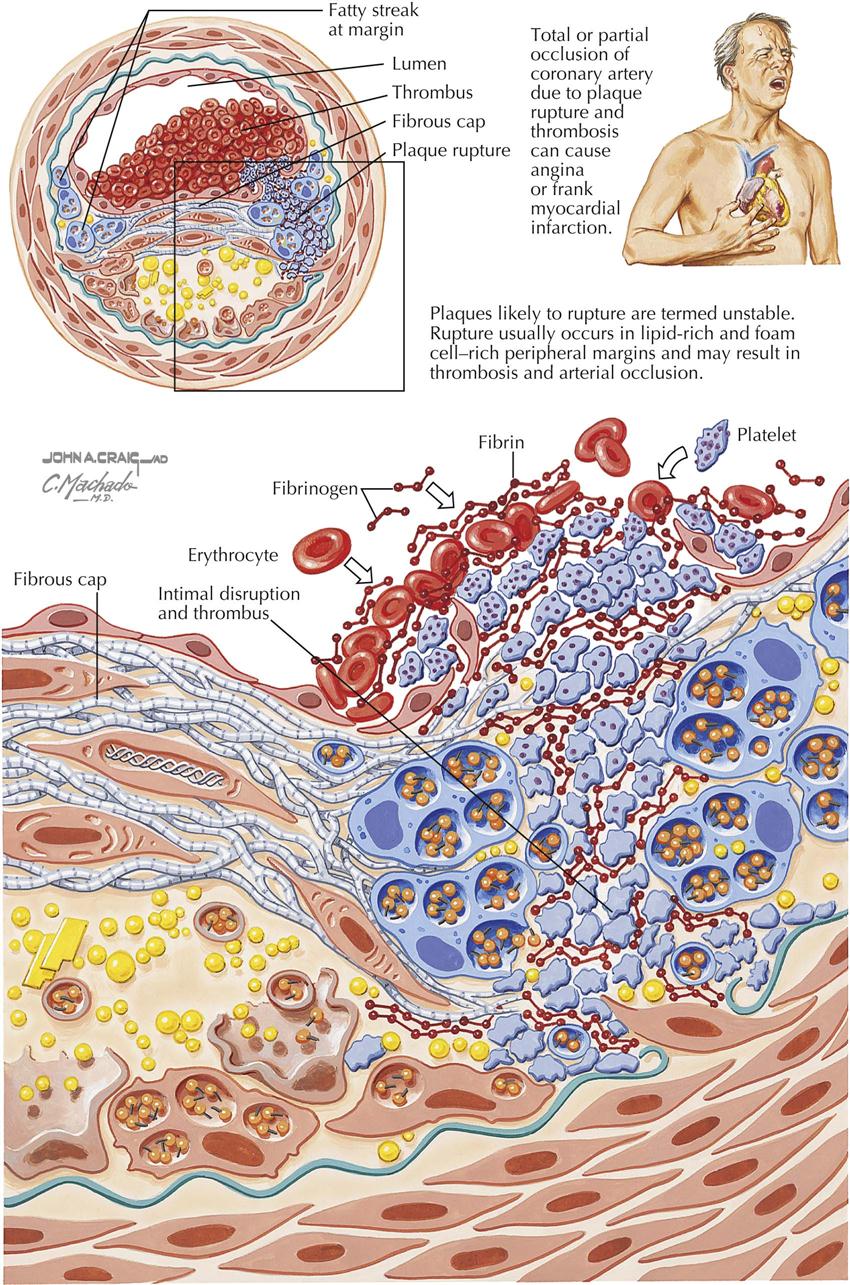
Plaques likely to rupture are called “unstable” and are the main cause of acute coronary syndromes. Plaques with a thin cap and a lipid core associated with inflammation and cap fatigue may be associated with rupture at the edges of the plaque. The plaques are rich in lipids and foam cells, found at the peripheral margins. On rupture, plaques extrude thrombogenic material, which then can trigger thrombosis on the plaque, in turn occluding the coronary artery and resulting in MI.
If the vessel only is almost occluded, a condition known as unstable angina may result. Risk factors such as cigarette smoking, hypertension, diabetes mellitus, and dyslipidemia contribute to endothelial injury, causing smooth muscle cell proliferation, inflammation, and deposition of lipid within the blood vessel wall. These events may lead to the development of unstable plaques. Other physical factors may alter plaque composition and make a plaque vulnerable to rupture; cold temperature (32°-34° C) may promote the crystallization of liquid cholesterol, and these sharp crystals may perforate the cap. Cytokines, growth factors, and oxidative stress are other factors in progression of atherosclerosis and instability.
Thrombosis related to these plaques may be caused by endothelial erosion or plaque disruption. In general, plaque erosion occurs over high-grade stenoses, whereas thrombosis related to plaque disruption is often seen in patients with few stenoses. In addition, multiple triggers of plaque disruption are probably related to increased tension, bleeding originating from the vasa vasorum, bending and twisting of the arteries, and increased systolic and pulse pressures.
Angiogenesis and Arteriogenesis
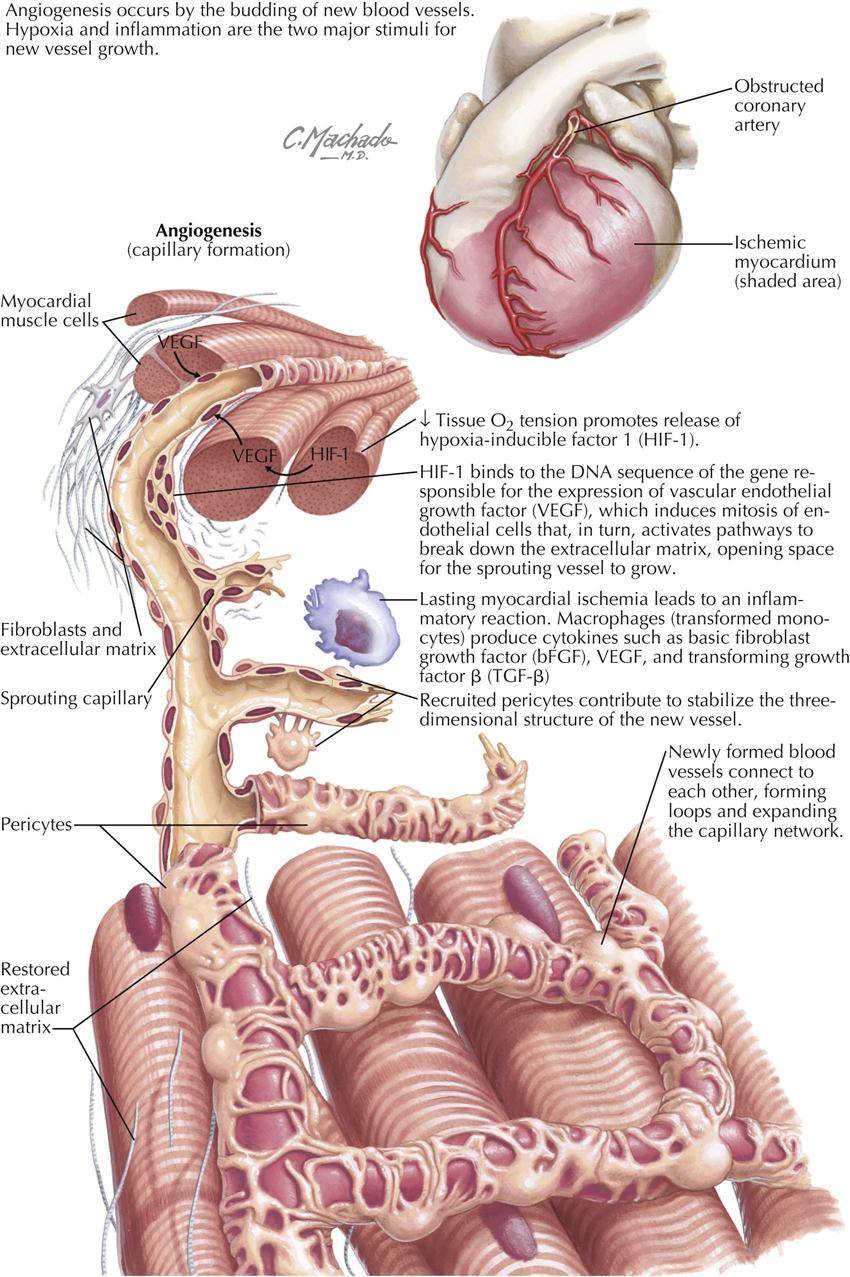
Unfortunately, the terms angiogenesis and arteriogenesis are often are confused and interpreted to have the same meaning. These processes are really two separate entities representing blood vessel changes in the heart.
Angiogenesis
Angiogenesis is essentially the budding of new blood vessels from sprouts of existing vessels in order to expand the capillary network (the microcirculation). Current investigations focus on creation of new blood vessels in areas of critical need, as in patients with large areas of infarcted myocardium surrounded by ischemic zones. The two major stimuli for new vessel growth are hypoxia, which activates hypoxia-inducible factor-1, and inflammation, which stimulates macrophages to secrete cytokines in MI patients, including basic fibroblast growth factor (bFGF) and transforming growth factor beta (TGF-β). As a result, newly formed blood vessels arise. This is particularly evident in animal models and is being tested in human trials (e.g., stem cell therapy in MI patients).
Arteriogenesis
In arteriogenesis, collateral vessels are recruited to increase blood flow to ischemic tissue. Coronary artery collateral vessels are thought to be stimulated and generated as a result of “demand ischemia” in areas of the myocardium. For example, the distal portion of a left anterior descending (LAD) coronary artery with high-grade stenosis or obstruction may receive blood from the right coronary artery (RCA) through septal collaterals or from the posterior descending coronary artery. Another potential source of collateralization is from the conus branch of the RCA, essentially one coronary artery supplying another artery, either by capillary-sized (microcirculation) collaterals or large muscular collaterals that are epicardial vessels (e.g., conus artery of RCA). This blood vessel is known to provide collateral blood flow to a severely stenotic proximal LAD artery.
Collaterals are not seen in patients without evidence of myocardial ischemia, possibly because no dormant collateral vessels are present. Also, in patients with balloon occlusion of an epicardial vessel and resultant myocardial ischemia, collateral flow through a previously dormant collateral vessel can be seen immediately. This indicates that these collateral vessels are dormant and visualized only when there is demand ischemia. Collateral vessels easily visualized at coronary angiography are categorized under arteriogenesis.
Overview of Myocardial Ischemia
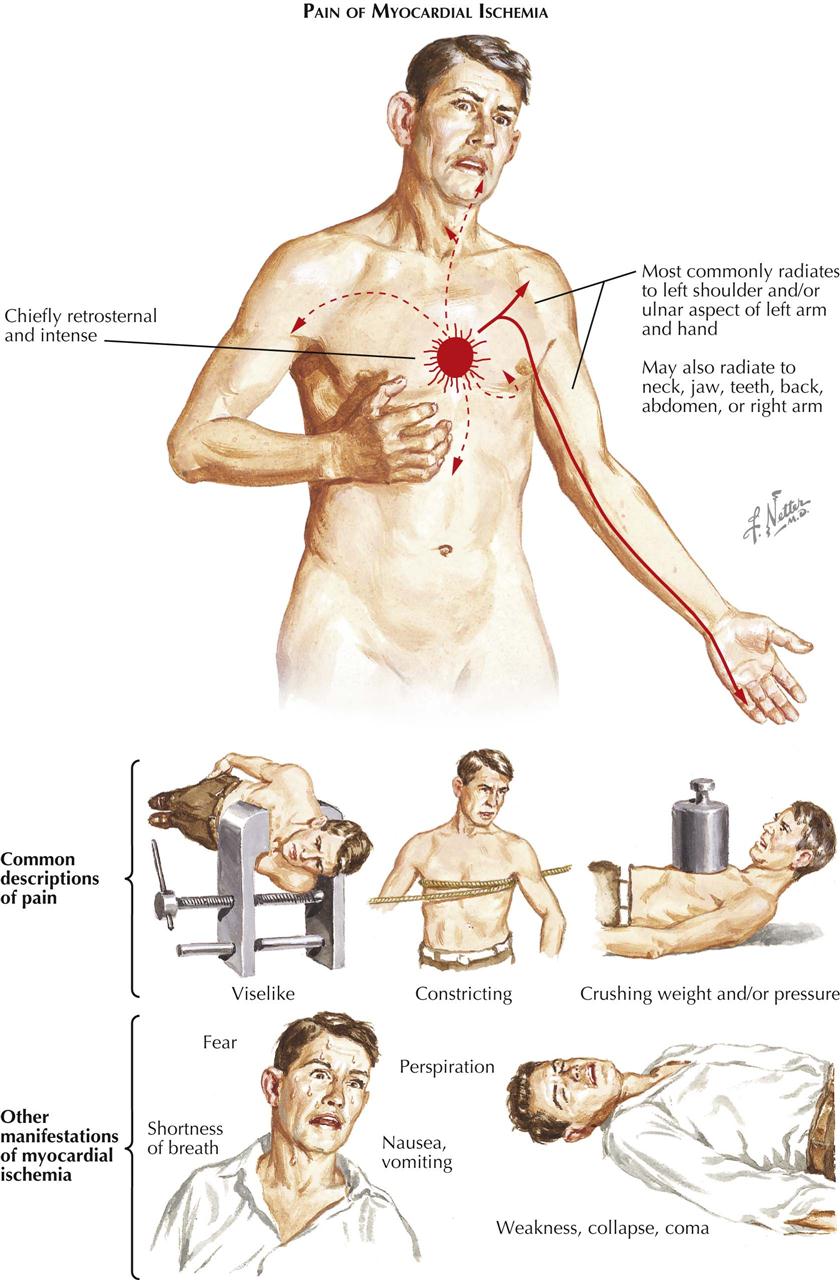
In patients without myocardial ischemia, myocardial oxygen demand is balanced by myocardial oxygen supply. Determinants of myocardial oxygen supply are related to aortic diastolic pressure, left ventricular end-diastolic pressure (LVEDP), and coronary artery resistance. Determinants of myocardial oxygen demand are related to heart rate, systolic blood pressure (BP), left ventricular (LV) tension, and LVEDP. Myocardial ischemia related to balloon occlusion of a coronary artery involves a sequence of decreased coronary blood flow, as detected by perfusion scintigraphy; altered regional flow/demand ratio resulting in decreased coronary sinus oxygen saturation; LV diastolic relaxation abnormalities, detected by cardiac ultrasound; systolic contraction abnormalities, detected by cardiac ultrasound or angiography; increased LVEDP; ST-segment depression on electrocardiography; and finally angina pectoris or its equivalent symptoms (e.g., breathlessness).
At the cellular level, the current hypothesis is that myocardial ischemia increases the late sodium current, which increases the sodium content of the myocardial cells. The sodium/calcium exchanger then increases the calcium content of the cell. As a result, the LV stiffens, increasing LVEDP, which decreases endomyocardial blood flow, propagating the myocardial ischemia.
Myocardial ischemia and its usual manifestation angina pectoris result from an imbalance between myocardial oxygen supply and myocardial oxygen demand. Myocardial ischemia manifested by angina pectoris can be acute or chronic. In general, patients with an acute clinical presentation have a crescendo pattern of angina and are considered to be in an unstable or “acute coronary syndrome” state.
Although most patients with myocardial ischemia are symptomatic, 25% may be asymptomatic. However, most symptomatic patients have many episodes of asymptomatic myocardial ischemia. Laboratory manifestations of myocardial ischemia other than electrocardiographic (ECG) changes are abnormalities of perfusion, as indicated by nuclear studies, and transient regional wall motion abnormalities, usually detected by echocardiographic studies or other noninvasive imaging techniques (e.g., MRI). Clinical manifestations of myocardial ischemia are generally chest discomfort (angina pectoris), arrhythmias, and LV dysfunction in both systole and diastole.
Angina Pectoris
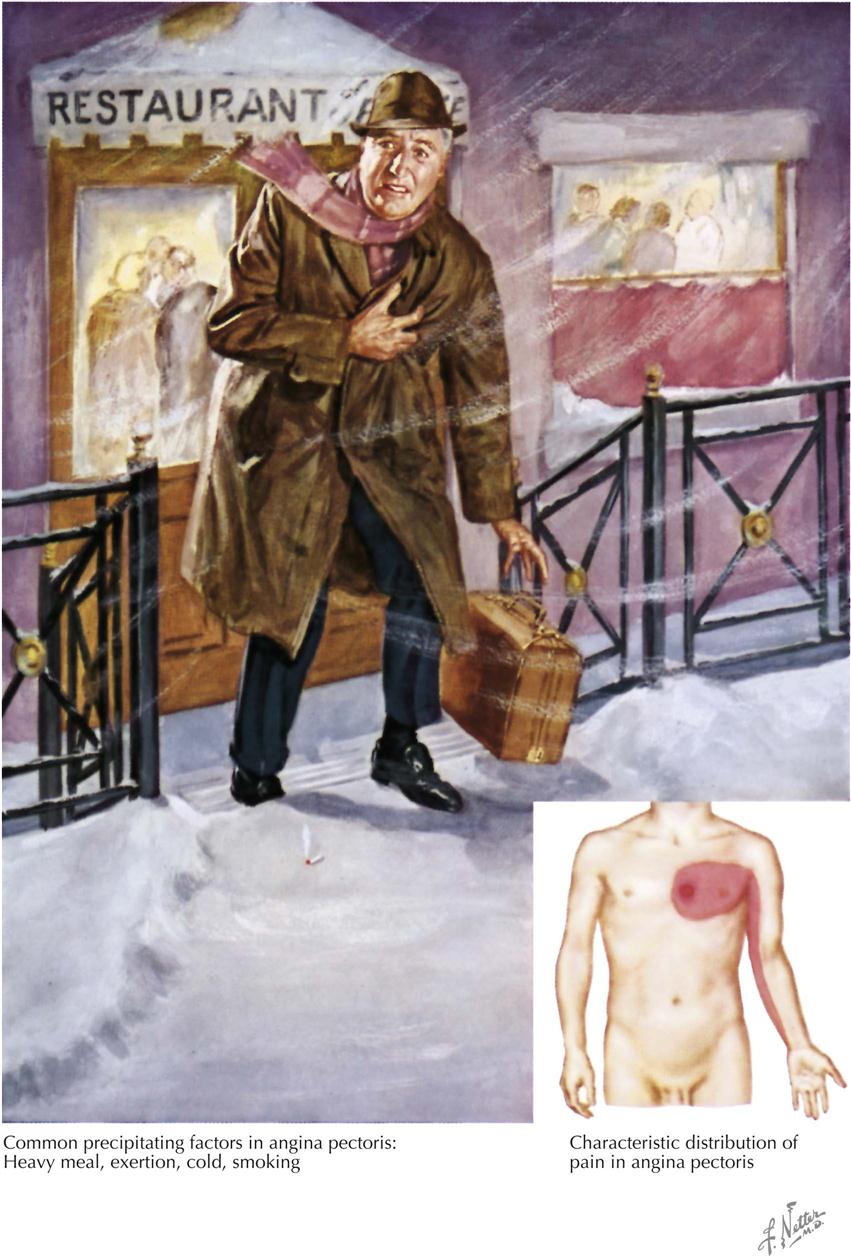
Angina pectoris (“strangling of the chest”) was first described as a serious symptom by Heberden in 1768, although he did not associate angina with coronary artery disease, as discovered later by Jenner. Angina is usually of short duration, associated with effort or emotion, and usually relieved in minutes by rest or sublingual nitroglycerin administration.
Angina pectoris varies somewhat from patient to patient, but the usual description of symptoms includes a feeling of a heavy weight, oppression, or a choking sensation under the middle of the chest and pain extending occasionally to the arms, especially the left, and almost never to the back and rarely to the neck and jaw. Many patients do not describe angina as a pain, but rather as a discomfort in the chest. It is produced, as a rule, by effort or emotion, especially in cold weather and particularly after meals or smoking. Angina rarely lasts more than 2 or 3 minutes and is relieved in a few minutes by sublingual nitroglycerin, also often used preventively, particularly if the patient knows a given situation will provoke cardiac pain. Angina must be differentiated particularly from gastrointestinal, musculoskeletal, and costochondritic origins of the discomfort.
Angina pectoris and the pain of acute MI are caused by the same disease process, but the prolonged pain in angina is associated with coronary artery occlusion and not the coronary artery stenosis of MI. The prognosis in the patient with angina pectoris varies greatly, but with medical management, many patients can continue to lead a normal life. Over months or years, their angina pectoris may diminish or disappear through natural development of collateral circulation to the ischemic zone of myocardium. However, angina is always an important symptom, and may be life threatening because of the potential for ventricular arrhythmias. Treatment of angina pectoris always includes medical therapy and often includes percutaneous coronary intervention (PCI, angioplasty/stent) or surgical bypass of a stenotic artery. Diet, blood pressure control, lipid management, nitrates, beta blockers, angiotensin-converting enzyme (ACE) inhibitors, aspirin or other antiplatelet agents, smoking cessation, and exercise are considered optimal therapy before and after a revascularization procedure.
Prevention of the underlying disease that causes angina pectoris is paramount. For those at risk, as shown by family history and high serum cholesterol, the avoidance of obesity, a diet low in animal fat, routine exercise (if no heart disease), and smoking cessation deserve first priority in any health promotion and heart disease prevention program and should start in childhood.
Detection of Myocardial Ischemia
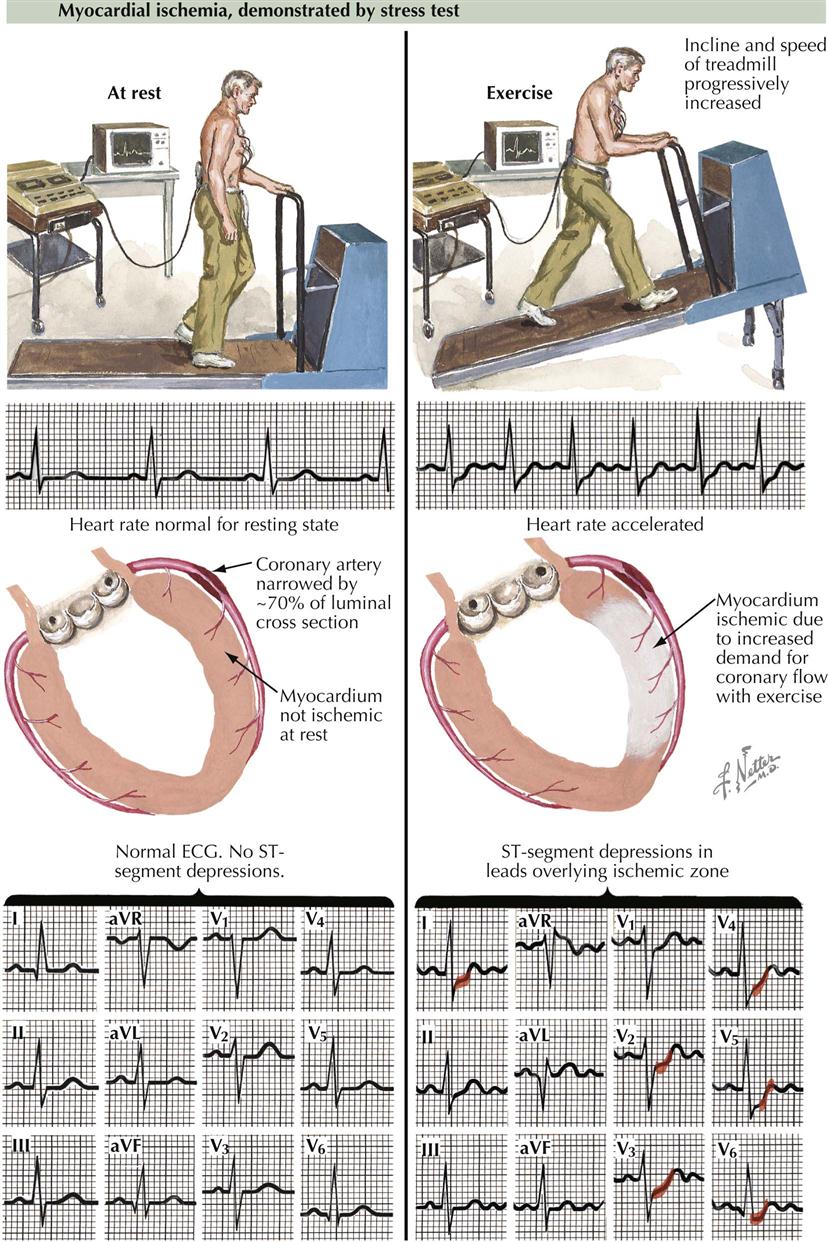
The simplest and least expensive way to diagnose the presence or absence of myocardial ischemia and to quantitate functional impairment in patients with chronic stable angina is exercise stress testing with ECG monitoring. Exercise testing is typically performed using a treadmill or bicycle to provoke chest discomfort and obtain diagnostic evidence of myocardial ischemia. It is important to record 12 leads before, during, and after exercise testing in order to detect transient changes suggesting ischemia. Under resting conditions the heart rate is usually normal and the ST segment on the electrocardiogram usually isoelectric. Thus the 12-lead ECG may be normal even if a coronary artery has 70% stenosis, the usual percentage that results in myocardial ischemia when myocardial oxygen demand is increased. The ECG is normal because the myocardium is not ischemic under these resting conditions; myocardial oxygen supply is balanced by demand, and the patient does not complain of angina pectoris or equivalents such as breathlessness. The treadmill increases speed and incline progressively, thus stressing the patient, who needs to increase heart rate and blood pressure to maintain appropriate cardiac output to meet the demand. During bicycle exercise, resistance to pedaling is increased to accomplish the same increase in heart rate and blood pressure. Patients who develop myocardial ischemia show the classic ECG changes of ST-segment depression. In the patient with 70% stenosis, under conditions of increased myocardial oxygen demand, the balance of oxygen supply and increased demand will be upset, and myocardial ischemia will result. In some patients, myocardial ischemic changes on the ECG may not be accompanied by angina pectoris, termed silent myocardial ischemia. Despite the lack of symptoms, the prognostic importance of silent or asymptomatic myocardial ischemia is the same as symptomatic disease.
Chronic stable angina is often called stable ischemic heart disease. Myocardial ischemia results when the oxygen needs of the myocardium exceed the supply of oxygen derived from coronary blood flow. The oxygen needs of the myocardium are increased by aggravating or precipitating factors such as hypertension, tachycardia, heart failure, hypermetabolic state, and sympathomimetic drugs. Myocardial oxygen demand depends on heart rate, blood pressure, and LV size, thickness, and contraction. Other factors, such as severe anemia, may result in tachycardia and increased oxygen demand.
Degree of Flow-Limiting Stenoses
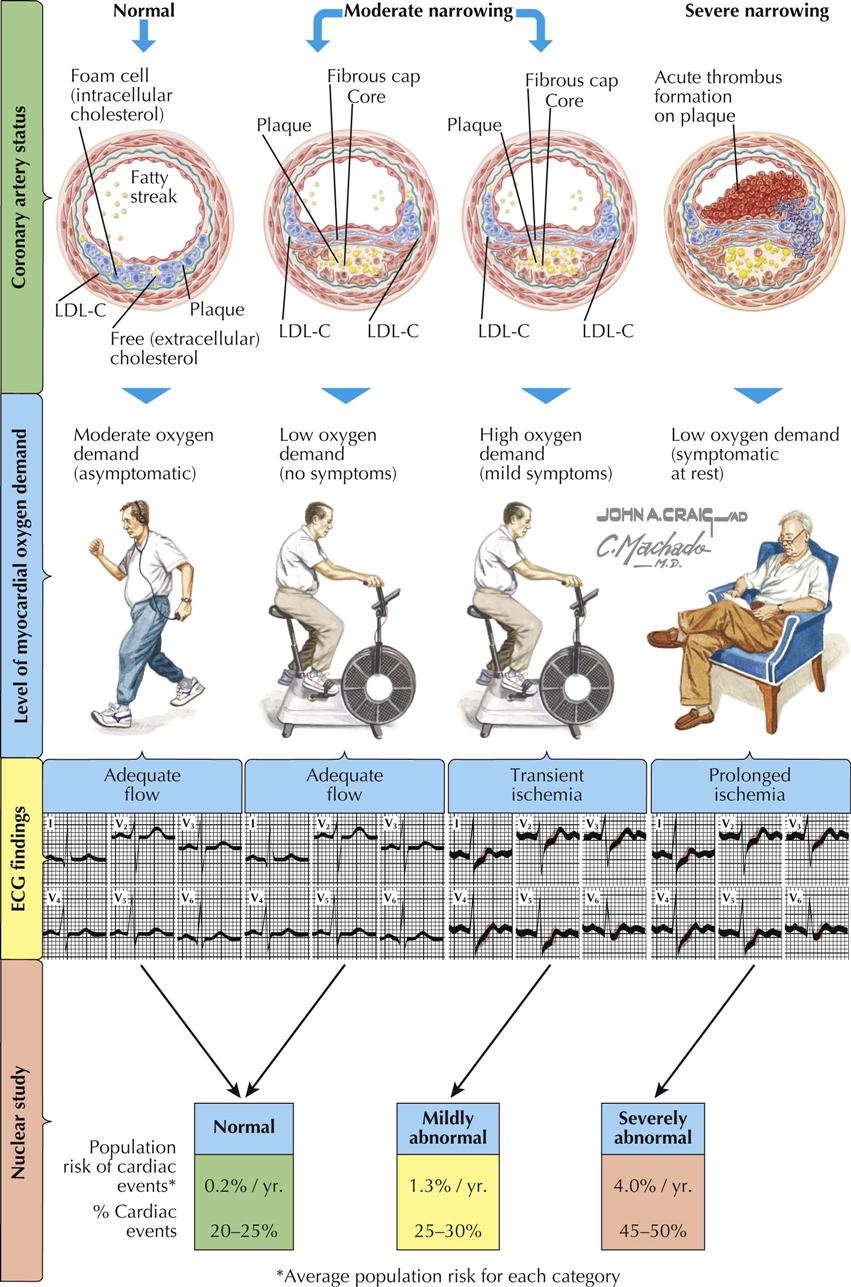
Patients with normal coronary arteries have no flow-limiting epicardial stenoses and remain asymptomatic under conditions of moderate oxygen demand, or even high oxygen demand in most cases. Patients with moderate narrowing caused by coronary atherosclerosis may also be asymptomatic or symptomatic (angina, myocardial ischemia) depending on the degree of flow-limiting stenosis to the myocardium. When flow is adequate under moderate myocardial oxygen demand, the ECG may be normal with isoelectric ST segments in all 12 leads. However, when there is high myocardial oxygen demand in this same patient with moderate coronary atherosclerosis, ECG changes in the appropriate leads (e.g., aVL, V2-V6) in patients with left-sided coronary disease demonstrate abnormal ST-segment shifts during transient ischemia and abnormal changes during prolonged ischemia at rest. In Plate 6-11, LDL-C refers to low-density cholesterol.
Thus a normal ECG correlates with widely patent coronary arteries providing adequate flow and no evidence of ischemia. Similarly, moderate coronary artery disease (CAD) under mild stress conditions shows no ECG changes. Under high oxygen demand in patients with moderate CAD, however, transient ST-segment shifts can be found. In addition, patients with severe narrowing often have symptoms and ECG changes under resting or near-resting conditions or during trivial exercise, particularly with severe high-grade proximal stenoses (e.g., left main or proximal LAD stenosis). These patients usually have severe proximal multivessel epicardial CAD.
If done to detect myocardial perfusion abnormalities, nuclear imaging studies are usually normal if the coronary arteries have mild or no stenosis. If there is moderate stenosis with high oxygen demand, myocardial perfusion may be mildly abnormal. The treatment and prognostic implications are related to the severity and extent of the perfusion defects. For severely abnormal myocardial perfusion in patients with high-grade stenoses, aggressive medical therapy is often combined with PCI (angioplasty with stenting) or bypass surgery.
To make appropriate decisions about prognosis and therapy in the patient with symptomatic CAD, knowledge of coronary pathology must be coupled with physiologic assessment of the lesions.
Left-Sided Heart Angiography
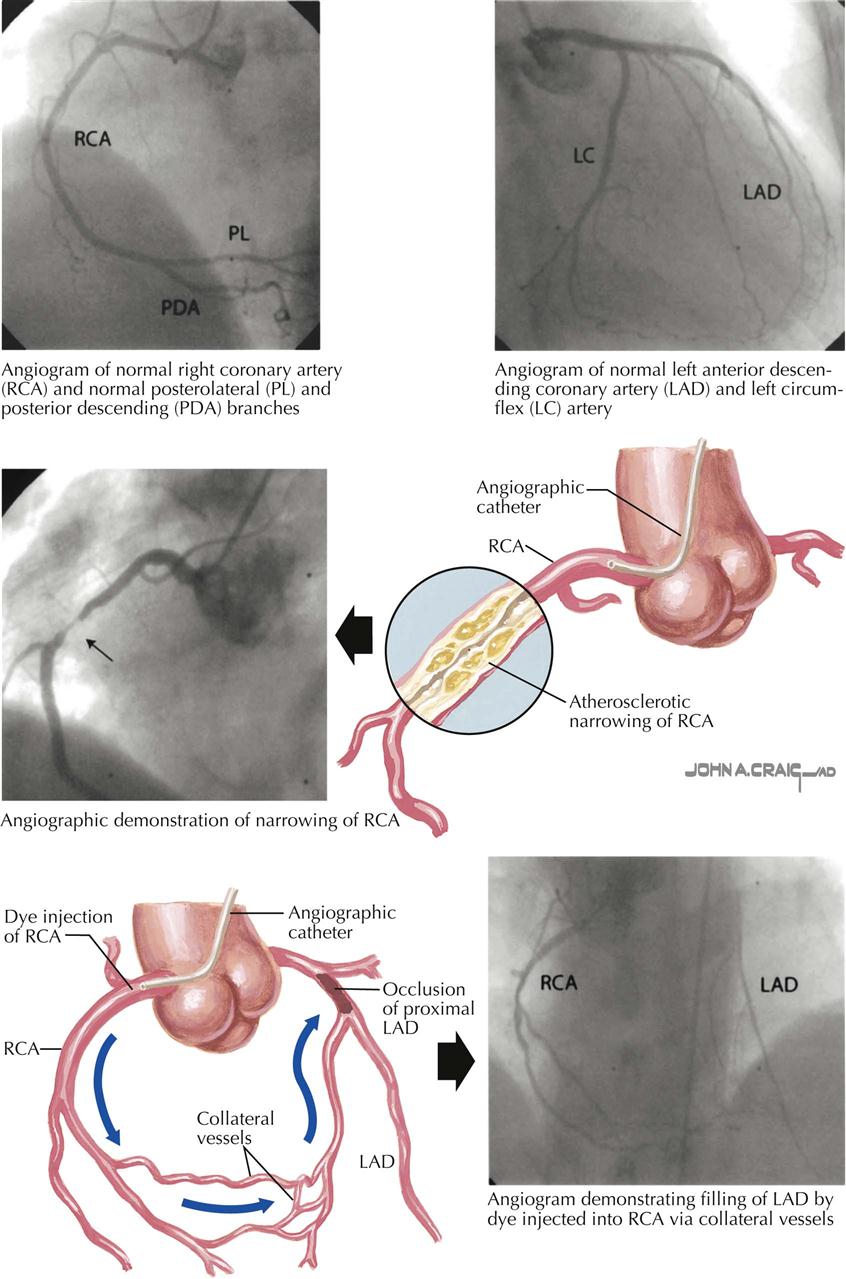
Left-sided heart catheterization and angiography includes injection of iodinated radiopaque contrast into the aorta, left ventricle, and coronary arteries to visualize the status of the aorta and its branches, presence or absence of aortic insufficiency, patency of conduit coronary bypasses, LV function and anatomy, and condition of the coronary circulation. Coronary angiography is a precise method of determining the extent, location, and severity (percent stenosis) of CAD. Multiple views of the coronary arteries are necessary to assess the significance of a specific lesion and the distribution of the right coronary, circumflex, and anterior descending arteries and the septal and diagonal distal circulation. The classic views of the right and left coronary arteries are the right anterior oblique (RAO) and left anterior oblique (LAO) projections. Multiple angulated views (caudal and cranial) are particularly important if PCI (angioplasty) is planned. One must remember that catheter-based coronary angiography is lumenography; only the vessel’s lumen is seen. The vascular wall must be visualized by intravascular ultrasound or optical coherence tomography; despite its limitations, this is a prerequisite for coronary artery surgery and in many patients allows determination of management: medical therapy (alone or plus angioplasty/stent) or bypass surgery.
Ventriculography is part of the evaluation of patients undergoing coronary angiography, to obtain the best possible assessment of ventricular function. I prefer to have ventriculography performed in the RAO and LAO views to assess the global function of the ventricle, particularly in patients with LV dysfunction.
Fractional Flow Reserve
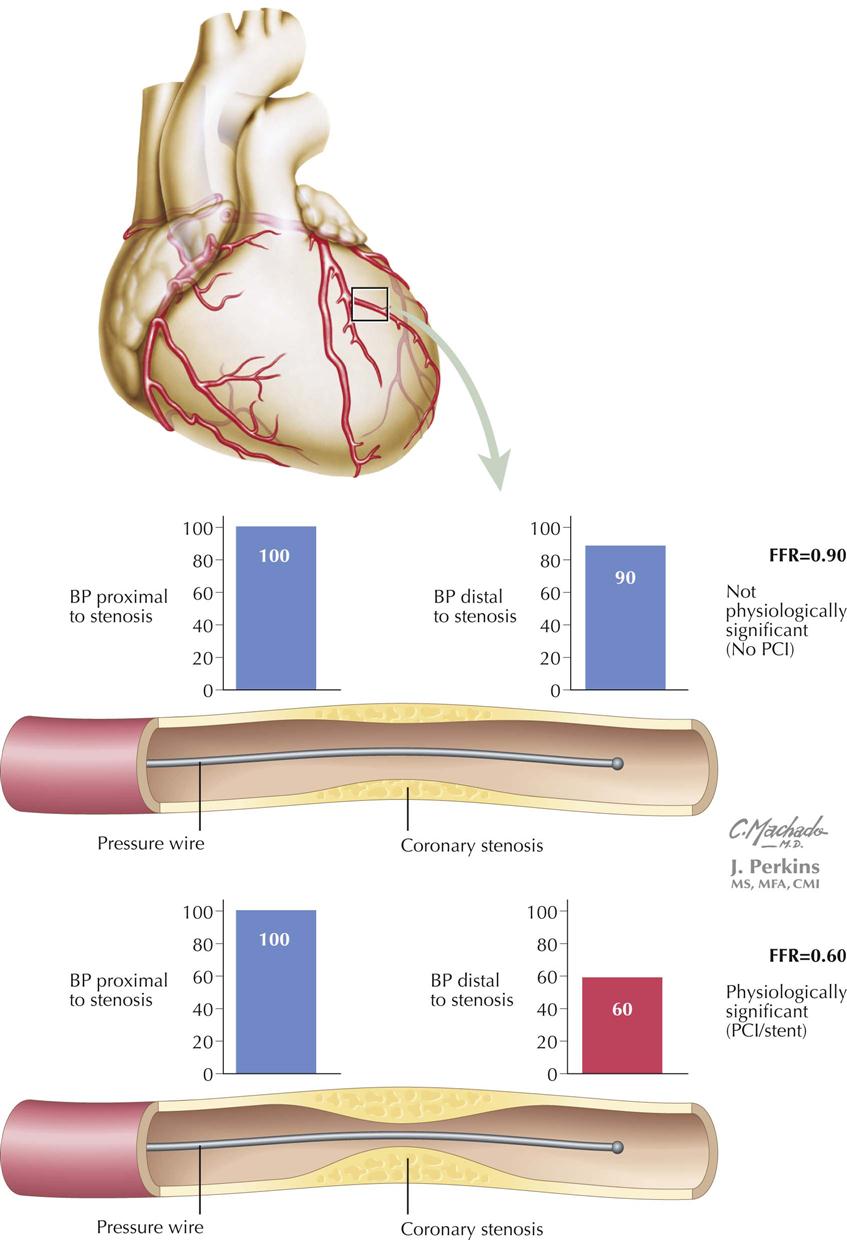
In the catheterization laboratory, interventional cardiologists can determine the physiologic significance of a specific coronary artery stenosis by passing a small wire (0.014-inch pressure guidewire) with a pressure transducer at the tip, through the stenosis in question, which allows them to measure systolic blood pressure (BP) proximal and distal to the stenosis. The systolic BP distal to the stenosis is divided by the pressure proximal to the stenosis; the resulting fraction is the percentage of BP decrease across the stenosis. Measurements are made at baseline and after infusion of adenosine, which increases coronary blood flow. For example, if BP across the stenosis drops by 50%, the fractional flow reserve (FFR) will be 0.50. The formula is FFR = Pd/Pa, where Pd is pressure distal to the stenosis and Pa is pressure proximal to the stenosis. This technique is particularly useful when the visual estimate of the clinical significance of a specific stenosis by angiography is uncertain.
If FFR is combined with intravascular coronary ultrasound, the interventional cardiologist will be able to determine the physiologic significance of the stenosis as well as the nature of the plaque being investigated, i.e., stable or unstable vulnerable plaque. FFR could also be called “functional flow reserve” because it supports that measurements reflect the pathophysiologic significance of a stenosis. The technique obviously requires cardiac catheterization and a specially designed pressure guidewire in which the pressure is measured proximal to and distal to a given epicardial coronary artery stenosis. Based on clinical trials, a cut-off point of 0.75—0.80 has been used to indicate a significant pathophysiologically important coronary artery stenosis. In my view, this measurement is equivalent to our measurements of noninvasively measured ankle-brachial index (ABI), in which a difference in pressure between the arm and leg suggests pathologic decrease in leg perfusion. An advantage is that this technique can provide immediate information for angioplasty/stent being considered for an epicardial stenotic vessel. If FFR is less than 0.75, the stenosis appears to be causing downstream myocardial ischemia, and angioplasty/stent seems appropriate. The DEFER clinical trial used FFR to determine if PCI with stenting was necessary in patients with FFR greater than 0.75. In these patients, stenting of the coronary stenosis did not influence clinical outcome. In another study, guided by FFR, fewer stents were used in patients who had FFR in the normal range. After 1 year, death, MI, or repeat revascularization occurred less in the FFR group, and hospital stay was shorter with less procedural cost.
Chronic Angina Revascularization Procedures
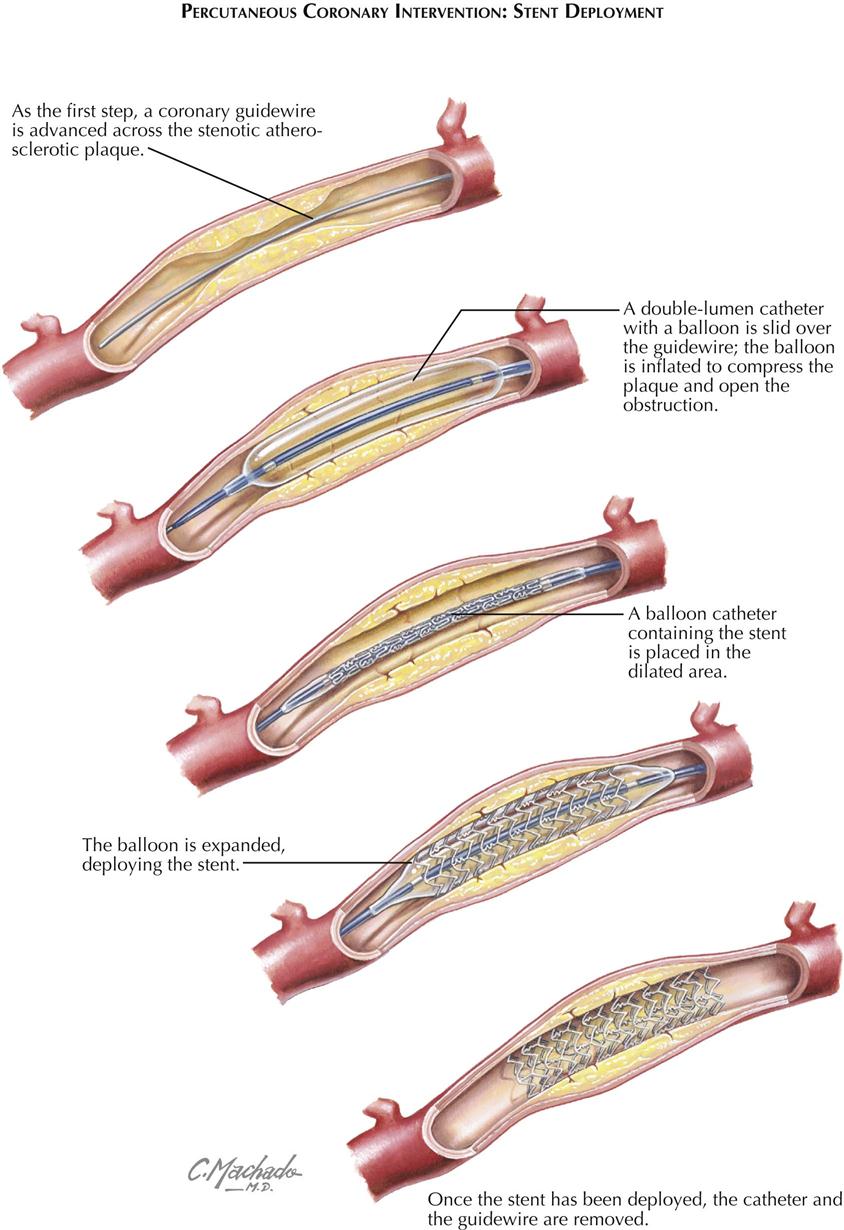
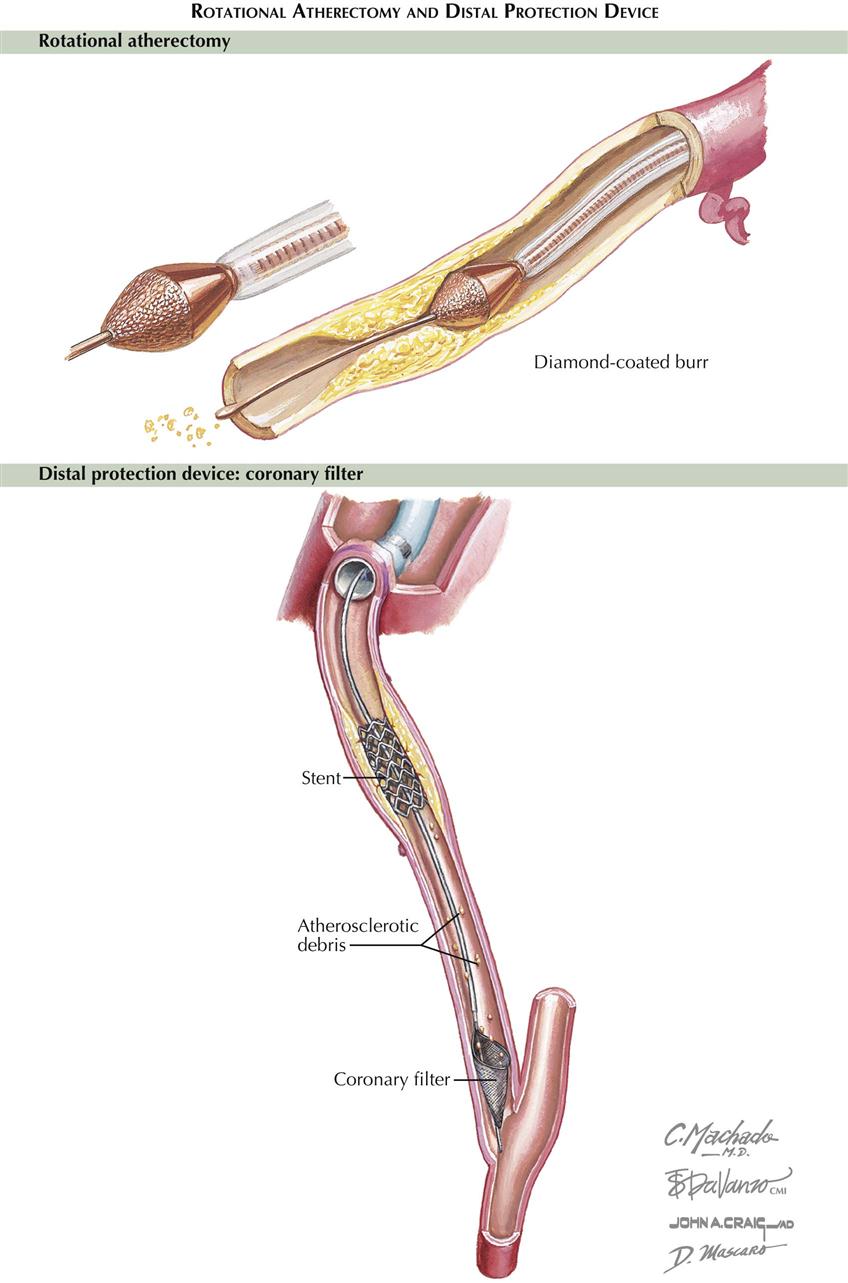
Revascularization involves either percutaneous coronary intervention (PCI), as first performed by Gruenzig in 1978, or coronary artery bypass graft surgery (CABG).
Percutaneous Coronary Intervention for Chronic Angina
Stent Deployment
The PCI is performed through a guiding catheter introduced into the ostium of a coronary artery. A guidewire is advanced into the catheter and passed across the stenosis, and a balloon catheter is then placed at the stenosis. The balloon is inflated and the stenosis dilated. If a stent surrounds the balloon, as the balloon is inflated, the stent is deployed and forms a scaffold to maintain patency of the artery (see Plate 6-14). Stents can be bare metal (BMS) or drug eluting (DES). The balloon is deflated and removed, and the stent remains in place.
Rotational Atherectomy and Distal Protection Device
Other devices such as rotational atherectomy can be used before stenting, particularly in calcified stenoses (see Plate 6-15). A diamond-coated burr is used to fragment the plaque, widening the stenosis. Occasionally, distal protection devices are used to filter some of the detritus and atherosclerotic debris that migrate distally.
Surgery for Chronic Angina Disease
In 1968, Favaloro performed the first successful coronary artery bypass graft (CABG) at the Cleveland Clinic, where Sones had done the first selective coronary angiogram in 1958. Selective coronary angiography permitted for the first time a realistic appraisal of the patient suspected of having coronary disease because it established the presence or absence of disease, localized an existing myocardial-perfusion deficit, and assessed LV functional status. Postoperatively, Sones’ technique allowed assessment of the disease course as well as surgical success or failure. This procedure also led to percutaneous catheter-based myocardial revascularization procedures.
Before CABG was developed, several procedures were used, but none is still performed, including implantation of the internal thoracic artery directly into the myocardium (Vineberg procedure) and a Vineberg variant (Sewell procedure) in which the internal thoracic artery with a pedicle (including artery, vein, muscle, and fibrous tissue) was implanted into the myocardium.
Ventricular Aneurysmectomy
Ventricular aneurysm may occur in patients who sustain a large MI. Postinfarction aneurysm usually affects the anterior wall and the LV apex perfused by the left anterior descending branch of the left coronary artery. Aneurysm of the inferior left ventricle rarely occurs, although an infarction of sufficient magnitude to produce a ventricular aneurysm usually results in mitral incompetency secondary to papillary muscle dysfunction.
Ventricular aneurysmectomy is usually an elective procedure that is not undertaken until several months after MI, unless the patient has intractable heart failure, intractable ventricular arrhythmias, or severe mitral regurgitation not responsive to acute vasodilator therapy. Early intervention in these patients greatly increases the surgical risk.
Coronary Artery Bypass Grafts
The concept of direct coronary artery surgery uses the saphenous vein graft or an internal thoracic artery to bypass a proximally stenotic coronary artery. CABG requires target vessels either free of disease or minimally diseased. Vein graft conduits are “free grafts” and connect the ascending aorta to the coronary vessel. In contrast, internal thoracic artery conduits remain connected to the subclavian artery and connect to the coronary artery distal to the stenosis. CABG not only promotes perfusion of the distal microcirculation of the epicardial vessel, but also provides perfusion of other stenotic vessels by enhancing flow through collateral circulation.
Follow-up Studies
Angiograms months or years after CABG show patency in a high percentage of grafted vessels. However, not all saphenous vein grafts are as patent at 10 years as they were initially. The use of the internal thoracic artery as a conduit to bypass a proximal stenosis has proved to be the best CABG procedure to date. Often, bilateral internal thoracic arteries are used. Although rare, this type of conduit can stenose or thrombose, probably related to technical intraoperative problems.
Pathophysiology of Acute Coronary Syndromes
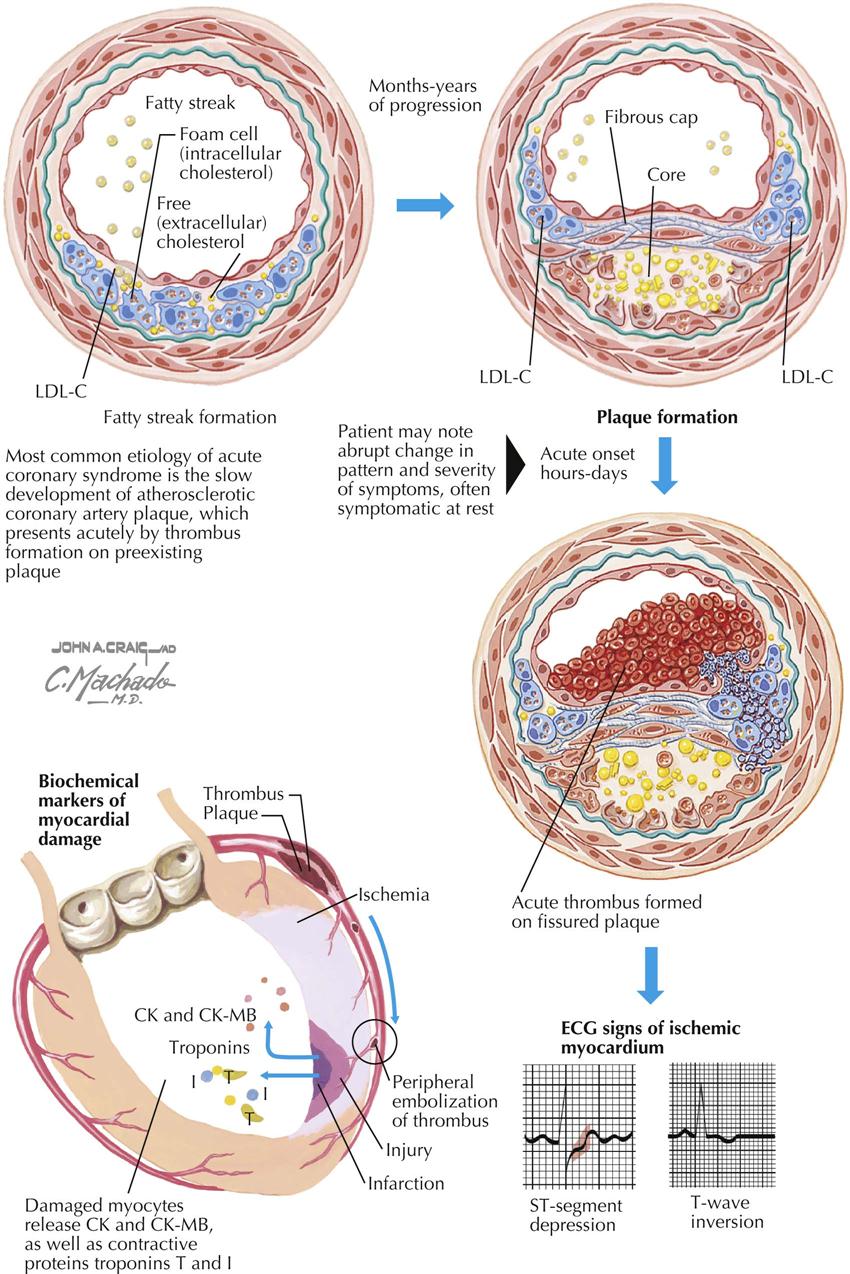
Patients with acute coronary syndrome (ACS) present in three different ways: unstable angina, non–ST-segment elevation myocardial infarction, and ST-segment elevation MI. Potential causes for the development of an ACS include extracardiac factors in the patients with severe coronary atherosclerosis, such as hypertension, tachycardia, and anemia. In 1858, Virchow proposed that injury to the inner wall of a blood vessel, possibly caused by fat, might lead to inflammation and secondary plaque formation, similar to current theory. ACS is also caused by plaque disruption resulting in transient platelet aggregation and release of vasoactive substances in diseased vessels, dynamic or intermittent coronary artery thrombosis, hemorrhagic dissection into an atheromatous plaque, and progression of coronary stenoses from plaque healing.
Acute coronary syndromes result from myocardial ischemia initiated by two primary factors: platelet and thrombus formation and resulting intense vasoconstriction from accumulation of local thromboxane A2 and serotonin, reduction of local concentrations of endothelium-derived releasing factor (EDRF), and inhibitors of platelet aggregation. These events are usually preceded by rupture or erosion of the lipid-laden plaque and involve the balance between thrombosis and thrombolysis. Patients with ACS may stabilize clinically, but this may not be associated with stabilization of the plaque. Identifying these patients requires observation for recurrent ischemia and assessment of troponin levels and possibly C-reactive protein (CRP) levels. Abnormal test results indicate increased risk for future cardiac events.
The acute coronary syndromes are not a homogeneous condition. Clinical variables evident in many patients include ECG changes, release of creatine kinase and troponins, CRP level, and differences in LV function, as well as pathology on coronary angiography. In patients with ACS, the TIMI risk score helps to predict the risk for death, MI, or recurrent ischemia at 14 days. The risks are age over 65, three risk factors for CAD, prior 50% coronary stenosis, ST-segment change on admission, angina twice in 24 hours, aspirin use within 7 days, and increased serum cardiac markers. The more risk factors present, the higher is the rate of composite endpoints in 14 days.
Myocardial Infarction—Changes in the Heart
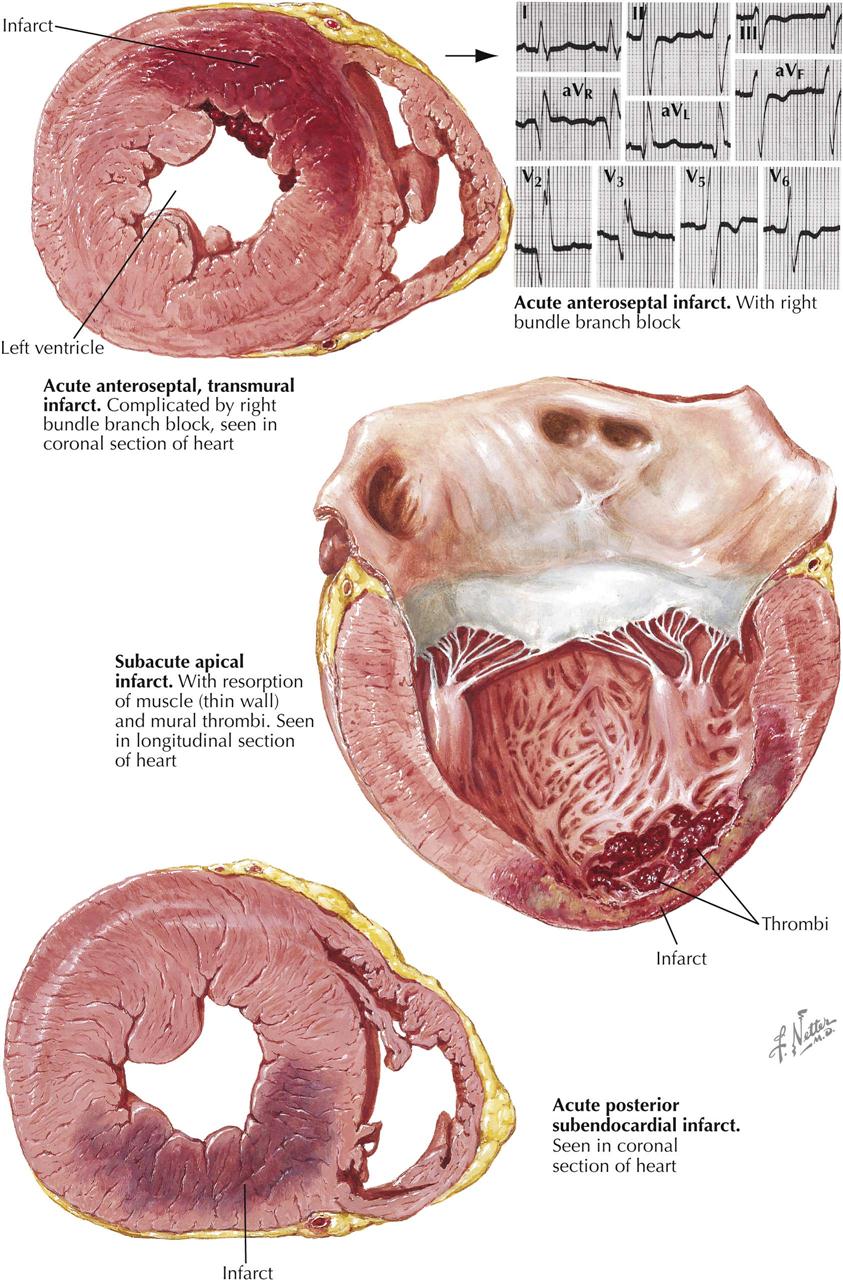
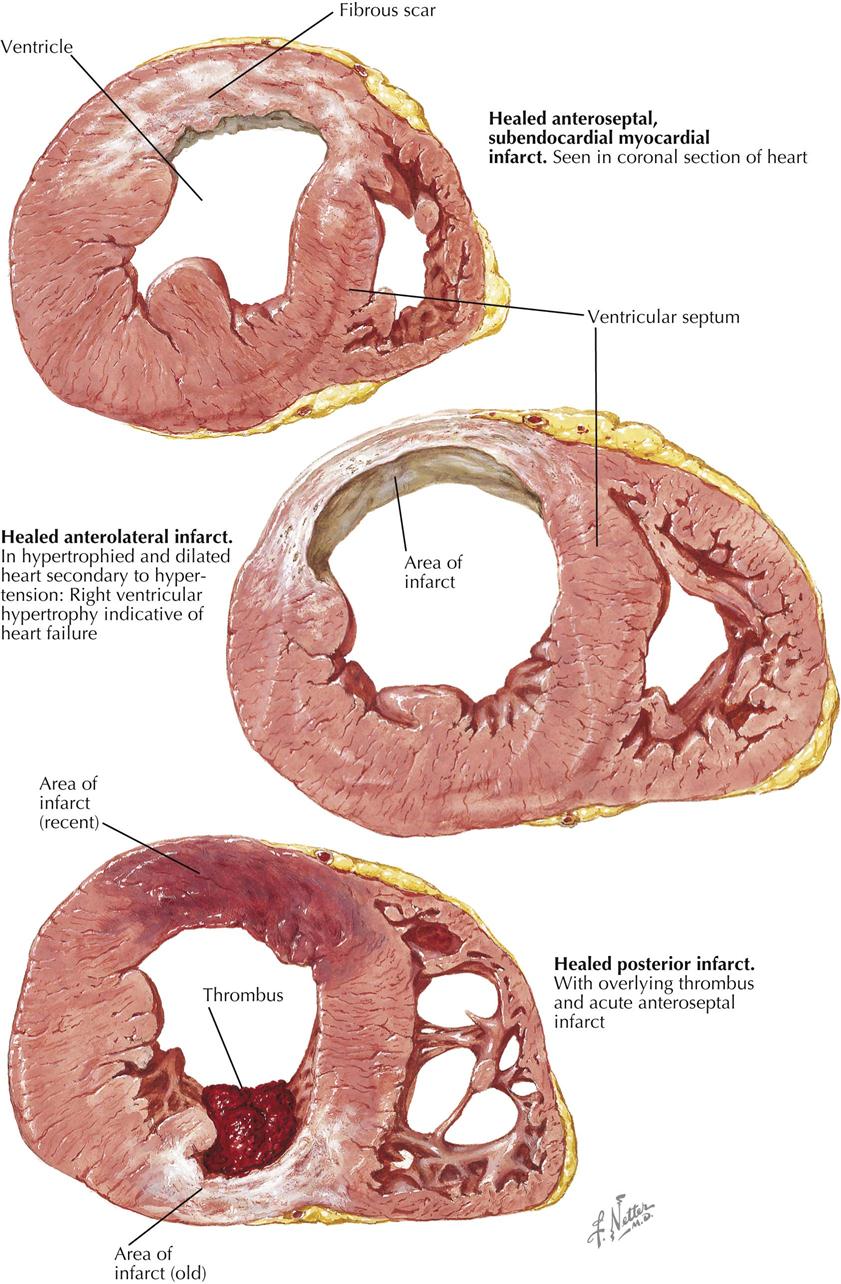
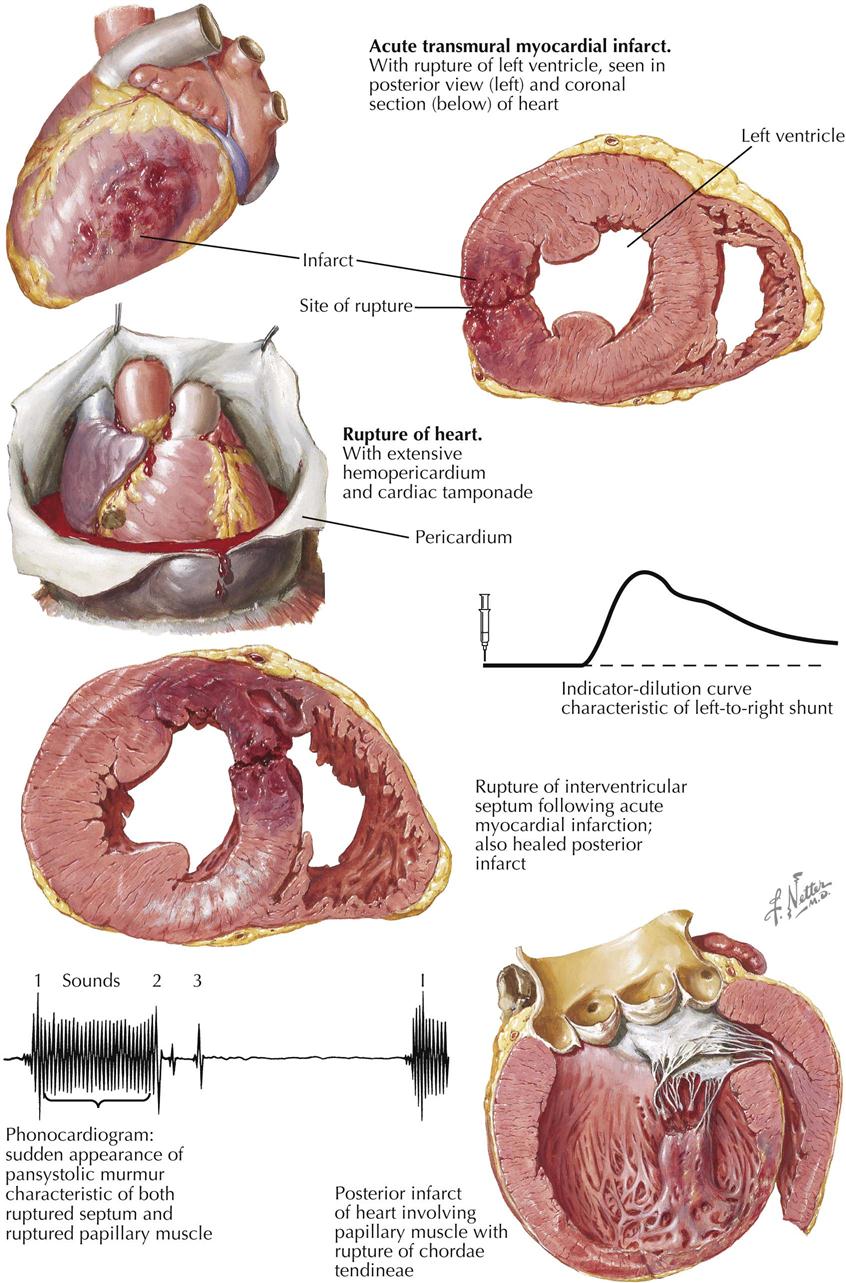
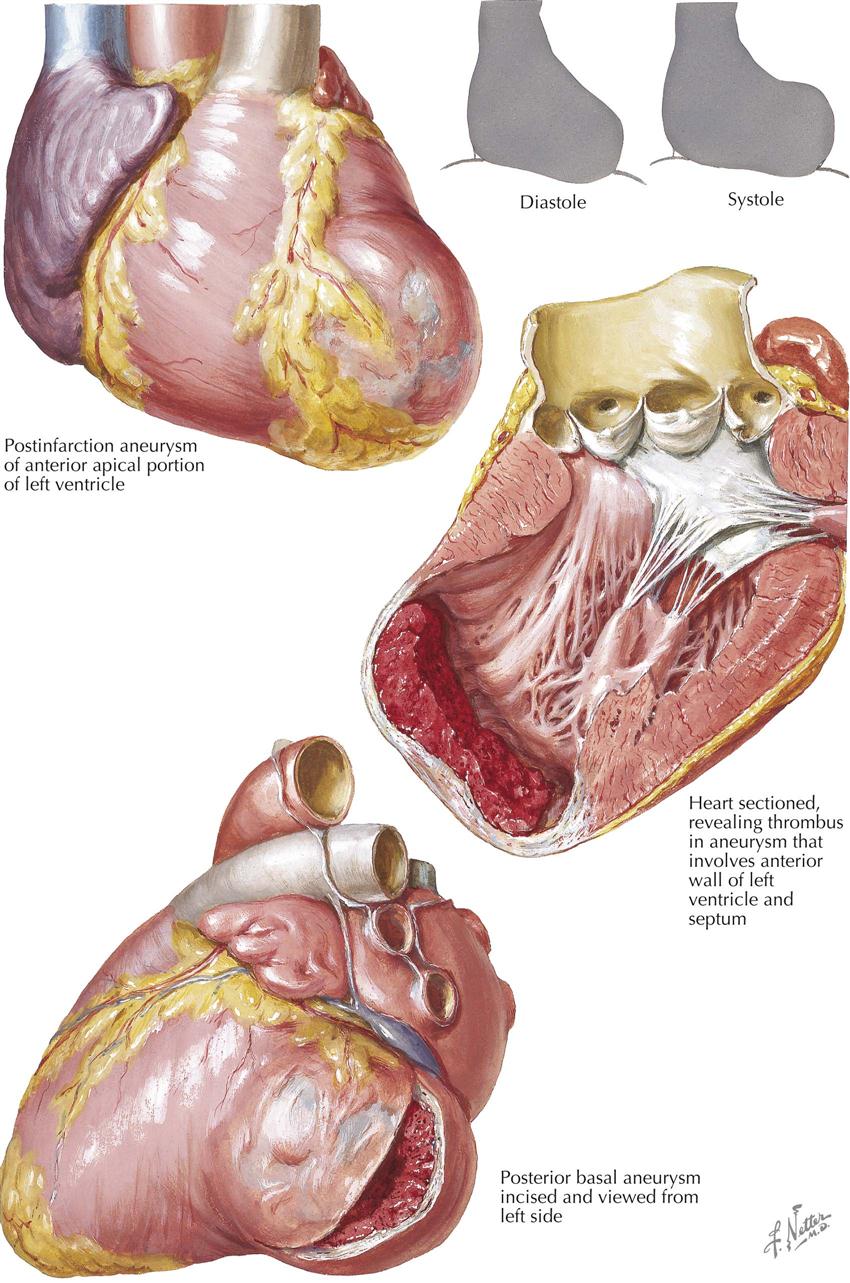
Myocardial infarction can result in an extensive panorama of changes. Death of the cardiac muscle may occur in a single zone or as multiple foci scattered diffusely throughout the heart. The latter can be found after suboptimal perfusion of the heart using a pump oxygenator, in which all the minute necrotic foci are of the same age. More often, however, the multiple minute foci can be found as destroyed zones of varying ages. In the acute phases, these microinfarcts may be extremely difficult to see and may be recognizable only microscopically as areas showing variable myofibril destruction and replacement by collagen. Chronically, these small foci may be minute white patches salted throughout the myocardium.
A larger myocardial infarct shows a variety of changes that depend in part on the duration of the patient’s survival after the episode. The earliest changes are associated with the resulting paralysis of vessel walls, engorgement of the capillaries, and migration of polymorphonuclear leukocytes (PMNs). Some autolytic changes then occur, and the dead muscle fibers can be recognized as showing a loss of striations and an increase in eosinophilia, fragmentation, and the gradual disappearance of nuclei. The dead muscle becomes an irritant, and more PMNs appear. Finally, the subsequent activities of repair include macrophage infiltration and replacement fibrosis. If the infarct is minute, such a sequence is of relatively short duration, but if large, remnants of necrotic muscle may be found several years later. It appears that such dead tissue has been effectively sealed away from physiologic activities.
The infarct may be named according to the area of the heart involved. Most posterior infarcts affect areas of the septum as well, and many anterior infarcts involve anterior portions of the septal wall, termed anteroseptal and posteroseptal (see Plate 6-17). A lateral infarct is found, in a small percentage of the cases. The sequence of events—muscle death, removal, and repair—may or may not result in thinning of the myocardium. Infarcts may also be classified according to the thickness of the ventricular wall involved. If all layers are affected (transmural), the term ST-segment elevation myocardial infarction (STEMI) is used, most often associated with occlusion of the coronary artery. In about 90% of these lesions, occlusion is demonstrated, as contrasted with a lesser incidence in non–ST-segment MI. In NSTEMI the zone of infarction is usually limited to the endocardial surface of the ventricle. Mural thrombosis may be a more common accompaniment of either STEMI or NSTEMI because both infarcts involve the endocardium.
A major problem with coronary artery disease is the lack of many correlations between CAD and MI in a patient who has died. Although the appearance of a lesion in the anterior descending or the main left coronary artery is a common finding in sudden cardiac death and occasionally may be the only lesion discovered, the status of the underlying cardiac muscle is not known because morphologic MI cannot be demonstrated. In about 25% of MIs encountered at autopsy—either acute or chronic but, especially, chronic—no history can be elicited as to when such a disorder occurred. In hearts with acute MI, only about half show occlusion of the coronary artery, although the other half exhibit a significant to marked stenosis of the infarcted vessel. In addition, when the immediate cause of death was believed to be CAD, no recent acute MI is found (see Plate 6-18).
Exercise may be extremely valuable in all these MI patients. Studies indicate that although the incidence of MI is not decreased, its severity is less in those who have maintained cardiac fitness.
Acute damage to areas of the conduction system, such as hemorrhage or infarction, can be demonstrated in only some cases. However, other complications are common. The immediate results of the changes in the circulatory status and constituents of the blood apparently can lead to a deposition of thrombus at numerous sites, including the coronary arteries, with a further extension of the MI. Mural thrombi form on the endocardium (probably related to healing of infarcted tissue), in the atrium (much more common on the left than the right), and possibly at all sites where previous atheromas had existed. Therefore, cerebral anoxia can result not only from the decreased cardiac output, but also from superimposed thrombosis during the early stages. Thrombosis also occurs in the systemic veins.
Embolism is a natural sequela of thrombosis, and systemic embolization occurs in an unknown number of patients with thrombi in the left side of the heart. Cerebral embolism is the most severe and damaging, although saddle embolism of the distal aorta is also serious, as is embolization in an extremity. Smaller emboli may produce any focal embolus, including splenic and renal arteries. Less often, mesenteric embolism may occur, which can be a medical disaster if not recognized early. Unfortunately, mural thrombi persist for a long period, and systemic embolization may occur at various periods following apparent recovery from the acute infarction. Pulmonary embolism is also often related to venous stasis.
As with the hypertensive heart in failure, the hypoxic heart can dilate, with resultant valvular insufficiency and subsequent regurgitation.
Rupture of the heart (ventricular septal defect, papillary muscle, free wall) is another variable autopsy finding (see Plate 6-19). The incidence of cardiac rupture ranges from 1% to 10% in deaths from acute MI. The area of rupture often shows a large increase in PMNs and at a much later time than such cellular elements are usually encountered; ruptures of the heart usually occurs in the first week. The classic patient prone to rupture is hypertensive, is having a first infarction, and has no visible collaterals to the infarcted artery. In addition to free rupture of the heart into the pericardial cavity and the resulting cardiac tamponade, rupture of the interventricular septum occasionally occurs. Rarely, papillary muscles rupture. The posterior papillary muscle is more likely to rupture than the anterior muscle because it does not have dual blood supply.
As a chronic complication, anterior and apical aneurysm may be more prone to mural thrombi, and thus the patient is continually exposed to the risk of embolism (see Plate 6-20).
In addition to all the complications of embolism, cardiac aneurysm, sudden death, and rupture of the heart, there is the problem of reinfarction. Some hearts demonstrate not only the zone of a previous infarct, but also areas of new infarct. The new infarct may be adjacent to the previous one or can occur in a different area of other coronary arteries.
Manifestations of Myocardial Infarction
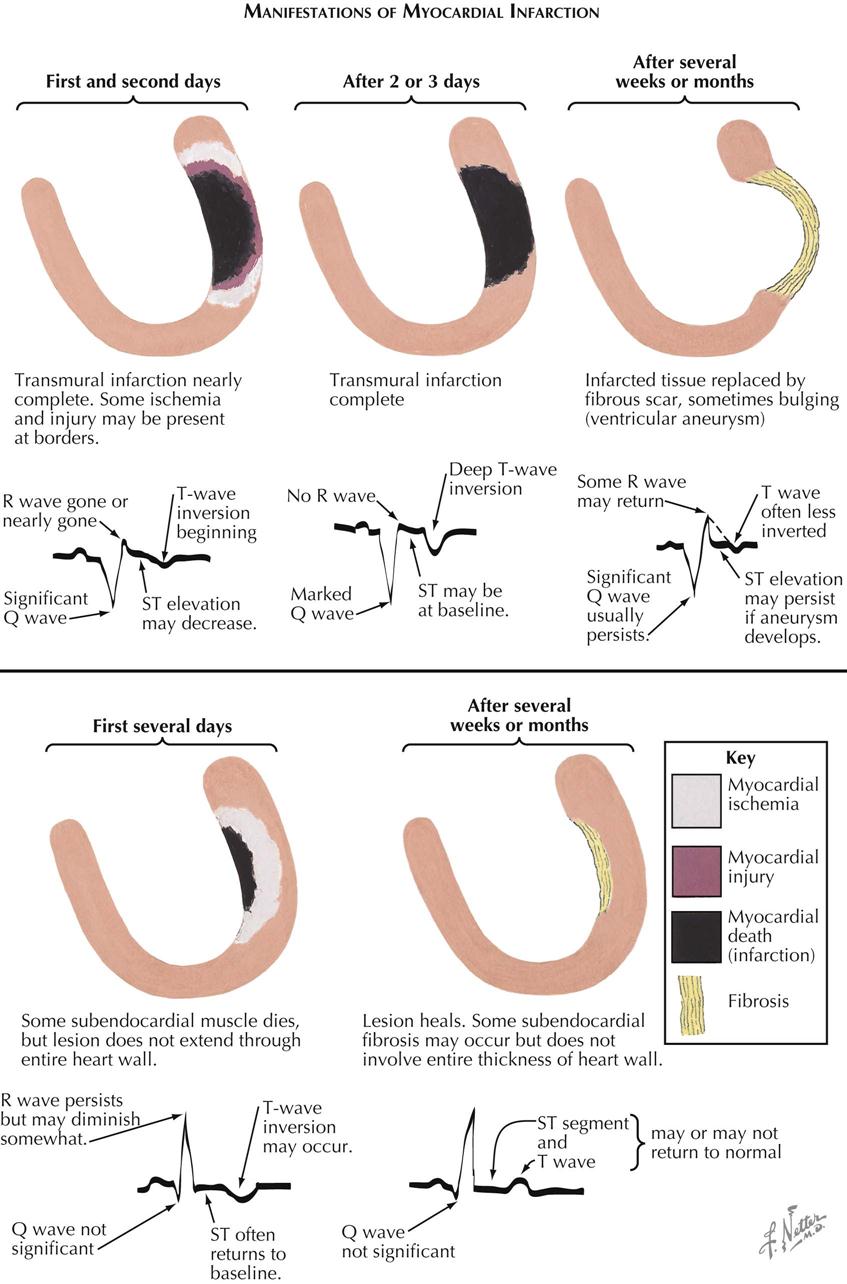
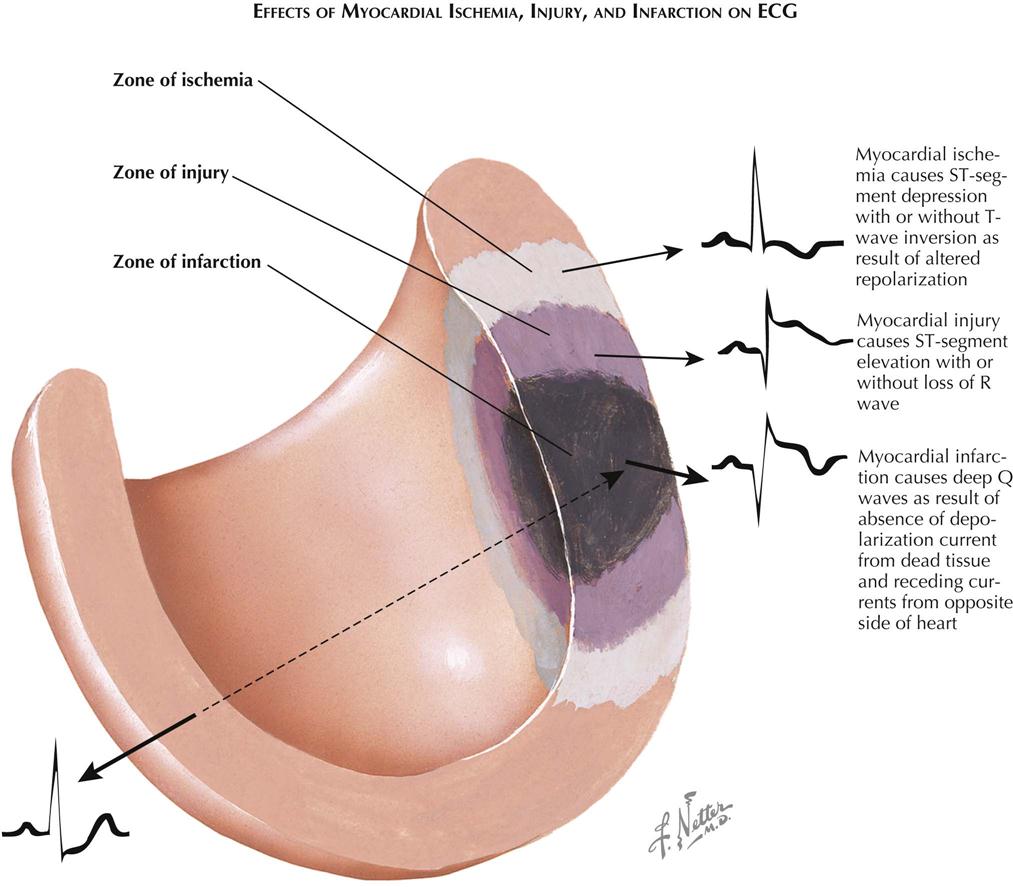
Pathologic and ECG Manifestations of Myocardial Infarction
In patients with acute MI, particularly with STEMI, pathologic changes in the heart may reveal necrosis from endocardium to the subepicardial myocardium. In the first 24 hours the ECG manifestations are variable, but usually the R wave is gone or almost gone, a Q wave is present, the ST segment is elevated, and the T wave may be inverted (see Plate 6-21). Several days later, pathology may reveal a complete infarction of the ventricular wall (transmural). The ECG continues to show Q waves, no R wave, and T-wave inversion. The ST segments may return to baseline in some cases. Several weeks or months later, the infarcted tissue may be replaced with fibrous scar, which occasionally results in a bulging ventricular aneurysm. If the aneurysm is obvious on ventriculography or cardiac ultrasound, the ST segment may be elevated. Other ECG changes usually persist (e.g., Q wave; inverted T waves, less than earlier seen), and the R wave may somewhat return.
In contrast, a patient with NSTEMI in the first 24 hours shows subendocardial necrosis, and the ECG often reveals persistent R wave, small Q wave, isoelectric ST segment, and T wave inversion. The infarcted myocardium is surrounded by a periinfarction ischemic zone. Days, weeks, or months later, the subendocardial necrosis heals, and subendocardial fibrosis replaces infarcted myocardium. This fibrosis is limited to the subendocardial area and does not extend transmurally to the epicardium. The ECG changes may or may not return to normal.
Ischemic Injury and Infarction: ECG Localization
In the myocardial region of an occluded coronary artery, ECG changes caused by ischemia, injury, and infarction can be identified (see Plate 6-22 ). ECG abnormalities are highly suggestive but not diagnostic of acute MI. In the patient who has large Q waves, ST-segment elevation, and T-wave inversion in several ECG leads, the diagnosis of an evolving MI is highly suspect; however, not all MI patients have these classic changes. Some patients present with severe chest pain and only T-wave inversion or a significant ST-segment depression, as well as elevation of cardiac markers (NSTEMI). In contrast, other patients have had obvious Q waves develop on the ECG and prominent ST-segment elevation (STEMI). All MIs begin in the subendocardial area and extend transmurally. The development of Q waves and ST-segment elevation probably relates to the transmural degree of wall involvement; the more involvement toward the epicardium, the more likely the appearance of Q waves and ST-segment elevation.
The site of the infarction can be localized in general by the ECG abnormalities. Thus, anterior and anterolateral MIs generally display ECG abnormalities of the precordial leads as well as leads I and aVL. However, all variations of these ECGs can occur, and there may be changes from V1 to V6 (including lead I) and aVL, or changes may occur only in leads I and aVL and the early precordial leads. In contrast, the diaphragmatic or inferior MI generally shows ST elevation in leads 2, 3, and aVF.
Cardiac Markers and Diagnosis of Acute Myocardial Infarction
Cardiac markers are essential for the diagnosis of acute myocardial infarction but do not appear immediately in the bloodstream if an occlusion occurs. Creatine kinase-(CK), CK isoenzymes (e.g., CK-MB), and troponin T are measurably abnormal about 4 hours after occlusion. Troponin T is the most sensitive and specific marker for acute myocardial infarction at the present time and probably is the only cardiac marker necessary to make the diagnosis of acute myocardial infarction; however, renal failure can result in the elevation of all cardiac markers in the absence of an acute myocardial infarction.
Clinically, if the cardiac markers are normal at admission to the emergency department (ED), when the patient is having acute cardiac pain and ST-segment elevation, the MI likely has occurred recently, within 3 or 4 hours. However, if the cardiac marker is elevated, the occlusion and STEMI likely occurred more than 4 hours before ED evaluation. The onset and duration of increase in CK enzymes and troponin T after a coronary occlusion vary, but in the patient with STEMI the occlusion usually occurred recently if initial serum values are normal. If a revascularization procedure is performed early in the course of acute MI, the cardiac markers my increase much more than if a revascularization procedure had not been performed, most likely from washout of the markers from the perfused myocardium.
Recanalization of Occluded Coronary Artery in Acute Myocardial Infarction
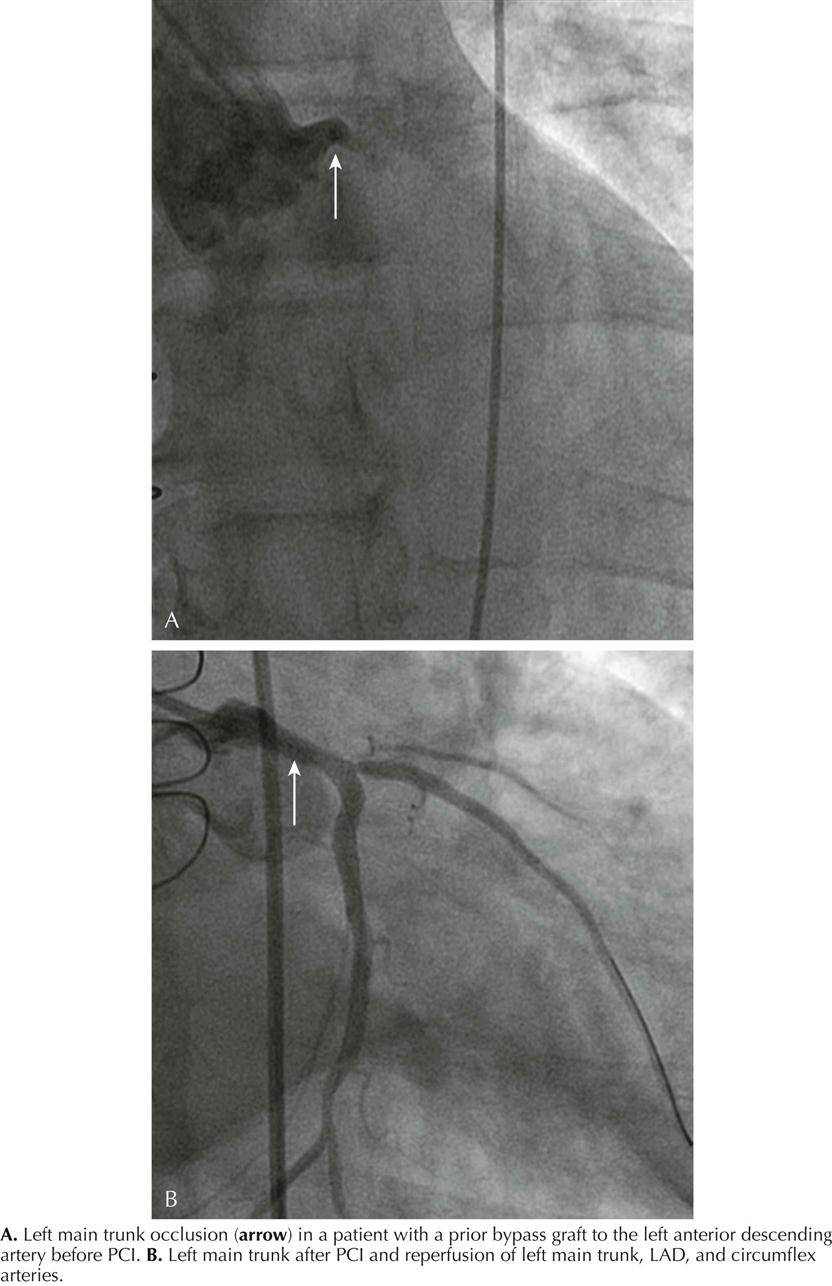
Rapid opening of the infarcted artery is generally accepted as critical to increase patient survival, preferably using PCI. However, thrombolytic therapy remains a viable option in hospitals when PCI capability is not immediately available. Percutaneous recanalization of an occluded coronary artery by balloon angioplasty and stent placement in patients with STEMI is the standard of practice. The appropriate management of acute STEMI currently is door-to-balloon (“D2B”) time—from ED arrival to passage of a guidewire into the infarcted artery. D2B time is easily and accurately measured and documented. D2B time less than 90 minutes is an acceptable goal. The rationale is that short D2B time results in less myocardial necrosis. A favorable outcome correlates well with minimal necrosis and ejection fraction (EF) greater than 40%. An unfavorable outcome correlates with a large amount of necrosis and EF less than 40%.2
Timing of Vessel Occlusion
In canine studies, MI size versus duration of coronary occlusion was measured with the circumflex coronary artery ligated. Thus the actual time of onset of coronary occlusion was identified and the time course of myocardial necrosis measured. Myocardial cells started to die about 20 minutes after onset of ischemia, with cell death complete by 6 hours.
Theoretically in the human, if mortality reduction is plotted against extent of salvage of myocardium from 0 to 3 hours, a steep reduction in percent mortality occurs, and by 3 hours the myocardial salvage is greatly reduced. After 3 hours the percent mortality reduction is similar to that found at 3 hours. Unfortunately, the exact time of vessel occlusion is not known in human MI.
Intra-Aortic Balloon Counterpulsation
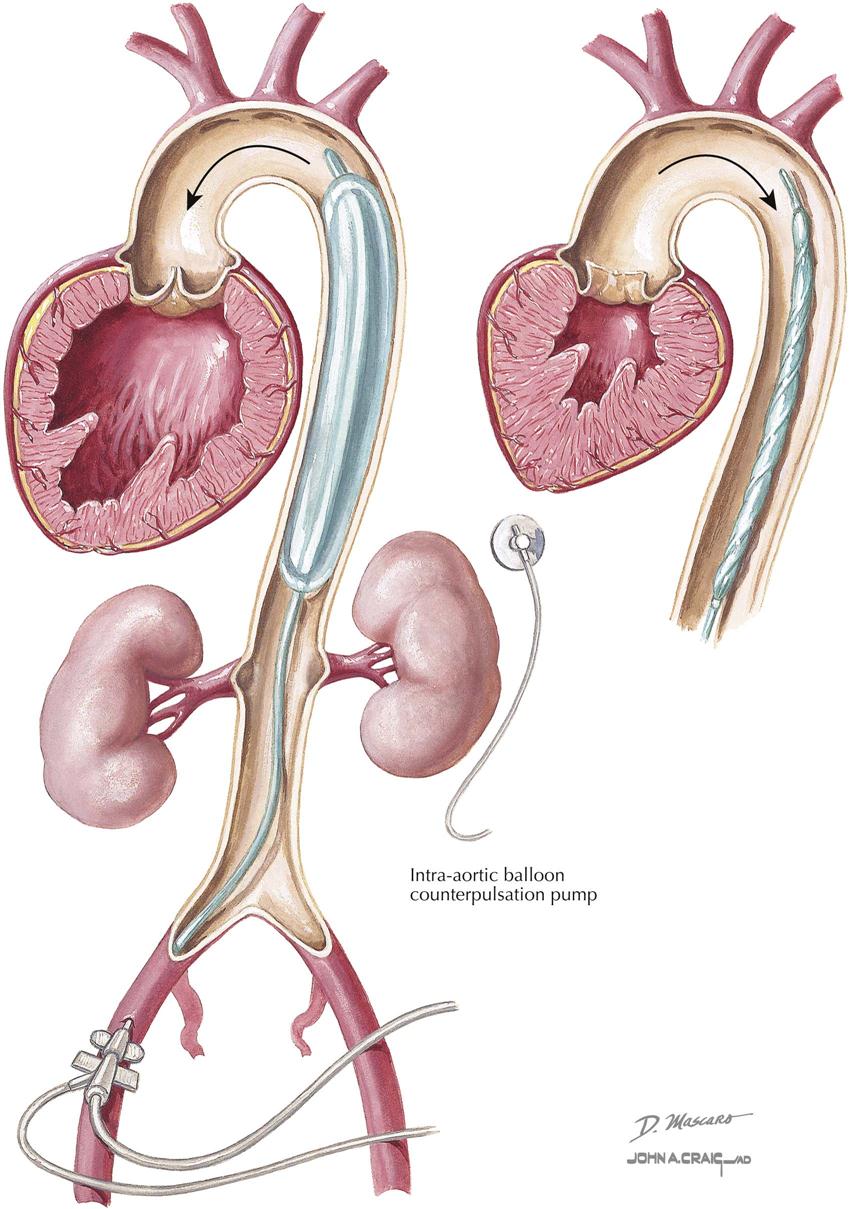
Counterpulsation is the only technique that will lower aortic systolic pressure and increase aortic diastolic pressure. To accomplish intra-aortic balloon counterpulsation (IABCP), a catheter with a cylindrical polyethylene balloon (~40 mL in adult device) is introduced into the aorta, deflated, and extended to just below the left subclavian artery and above the renal arteries, so as not to obstruct either vessel. When visualized on chest radiography or fluoroscopy, the tip of the catheter is placed at the level of the bifurcation of the right and left main bronchi, again so as not to occlude subclavian and renal arteries. Balloon expansion with helium occurs actively during diastole, and collapse of the balloon occurs actively during systole. Intra-aortic pressure is measured at the catheter tip.
Timing of balloon expansion and collapse is controlled by either ECG or pressure waveform during the cardiac cycle. When the balloon is expanded in diastole, aortic diastolic pressure increases, as does coronary perfusion pressure, which theoretically increases myocardial blood flow. When the balloon is collapsed during systole, LV afterload reduction occurs, probably a suction effect that unloads the left ventricle and increases cardiac output. When counterpulsation is working correctly, the elevated diastolic aortic pressure likely is interpreted as high blood pressure, and the baroreceptor reflexes tend to lower systolic pressure, thus decreasing myocardial oxygen demand.
The clinical indications for IABCP are cardiogenic shock of any etiology, acute mitral regurgitation that does not respond to nitroprusside, acute ventricular septal defect, and refractory acute coronary syndromes (ischemia or arrhythmias). Less clear indications relate to preprocedural use in patients considered “high risk” for a revascularization procedure such as PCI or CABG (e.g., high-grade left main coronary artery stenosis). IABCP should not be used in patients with aortic insufficiency; the increase in diastolic pressure will increase the degree of aortic insufficiency. IABCP cannot be used in patients whose aorta cannot be accessed because of severe peripheral vascular disease. Complications with IABCP are fortunately rare and include leg ischemia, aortic disruption, renal artery obstruction when the balloon is not properly placed, and bleeding.
Rheumatic Fever in Sydenham’s Chorea
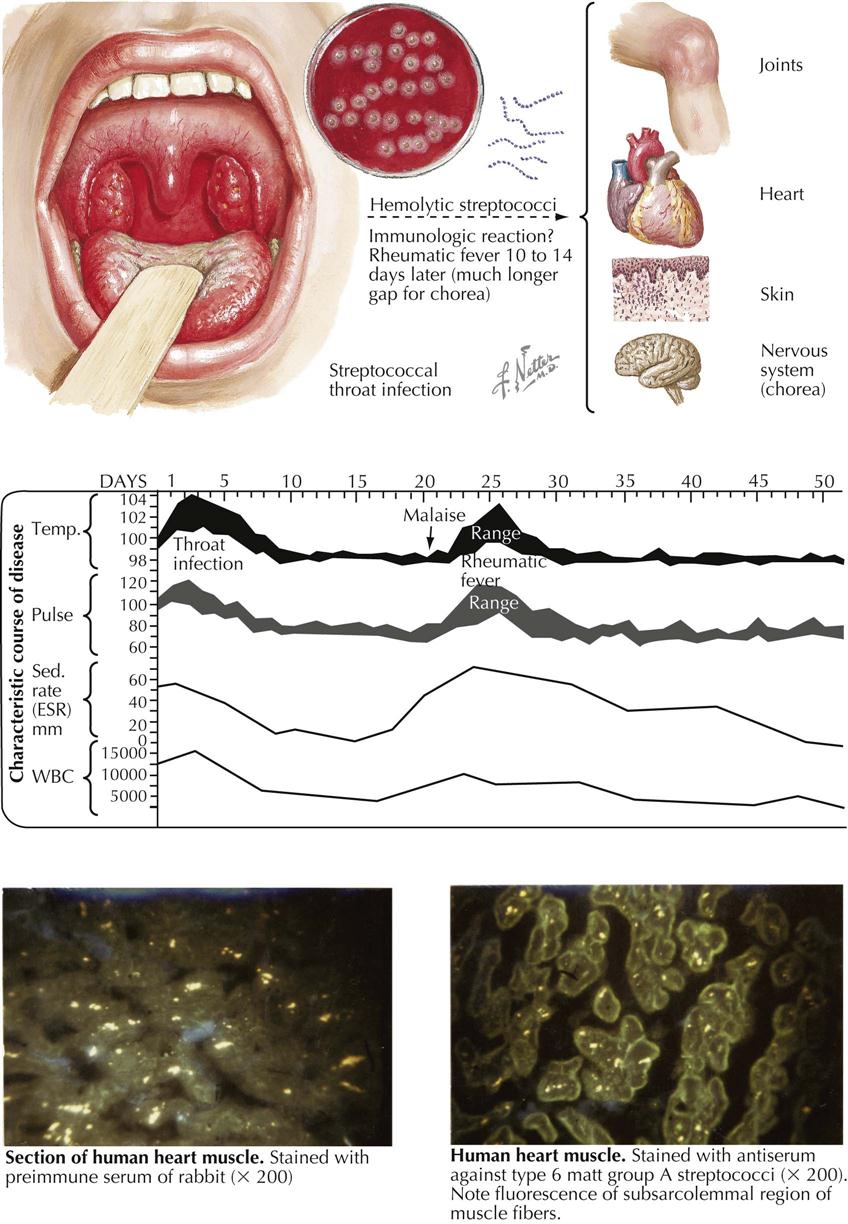
Rheumatic Fever
Although uncommon in the United States and other developed countries, rheumatic fever is common in developing countries and can affect people of all ages, except in the first years of life. The febrile onset is accompanied by severe but temporary arthritis and often by carditis affecting all three cardiac layers. The etiologic relationship of rheumatic fever to β-hemolytic streptococci is based on the following evidence:
The frequency with which rheumatic fever follows a streptococcal infection varies from 3% in epidemics to about 0.3% in sporadic infections. This discrepancy is largely caused by the greater severity of epidemic types of infection, as revealed by clinical features and the antibody titers induced. When streptococcal infections of comparable severity are studied, the incidence of subsequent rheumatic fever, even in sporadic cases, is also about 3%.
The lesions of rheumatic fever do not result from direct invasion by streptococci. When adequate precautions are taken against contamination, organisms cannot be isolated from the joints or the heart. The events relating the primary infection to these lesions are still unclear, but circulating toxins released from the organisms are unlikely to be responsible, because then the clinical manifestations of rheumatic fever would coincide with the sore throat (as do the toxic symptoms of diphtheria). Furthermore, none of the many known streptococcal products produces comparable lesions in animals. The relationship between streptococcal infection and rheumatic fever is therefore most widely regarded as immunologic, with the lesions resulting from an antigen-antibody reaction in the affected tissues. This concept was supported by observations that lesions superficially resembling those of rheumatic carditis could be produced in rabbits by the injection of massive doses of foreign serum.
A comparable massive exposure to antigen is unlikely to occur in humans. Any immunologic reaction underlying the pathogenesis of rheumatic fever is more likely to be more specific, directly involving one or more of the antigens native to the affected tissues. Many strains of β-hemolytic streptococci contain an antigen in their cell walls that cross-reacts with an antigenic component of mammalian hearts, including the human. Furthermore, animals injected with such strains of streptococci produce antibodies that react with cardiac antigens. Similar antibodies have been found in a high proportion of patients with active rheumatic fever.
However, despite the presence of firmly bound gamma globulin (presumably antibody) in the heart of many patients with rheumatic fever, circulating antibody correlates poorly with clinical severity. Cardiac damage in experimentally immunized animals is unconvincing; repeated infection in rabbits by different β-hemolytic streptococci most closely resembles human rheumatic carditis. Although the mechanism is still unclear, immunologic cross-reactivity with myocardial and other antigens is presently the most likely cause.
The clinical features of an acute attack may range from the trivial to the fulminating—from easily overlooked pallor and fatigue to an exquisitely painful arthritis with high fever, rash, and carditis sufficiently severe to lead to congestive heart failure. The temperature chart is characterized by a nonremittent type of fever with a pulse rate raised disproportionately. The arthritis is migratory, rarely lasting in any one joint for more than 1 or 2 days. Swelling is slight and accompanied by some flushing of the overlying skin. Large joints are more frequently affected than small joints, although these can become involved as well, and the clinical picture may then resemble rheumatoid arthritis.
Rheumatic Fever in Sydenham’s Chorea: Noncardiac Manifestations
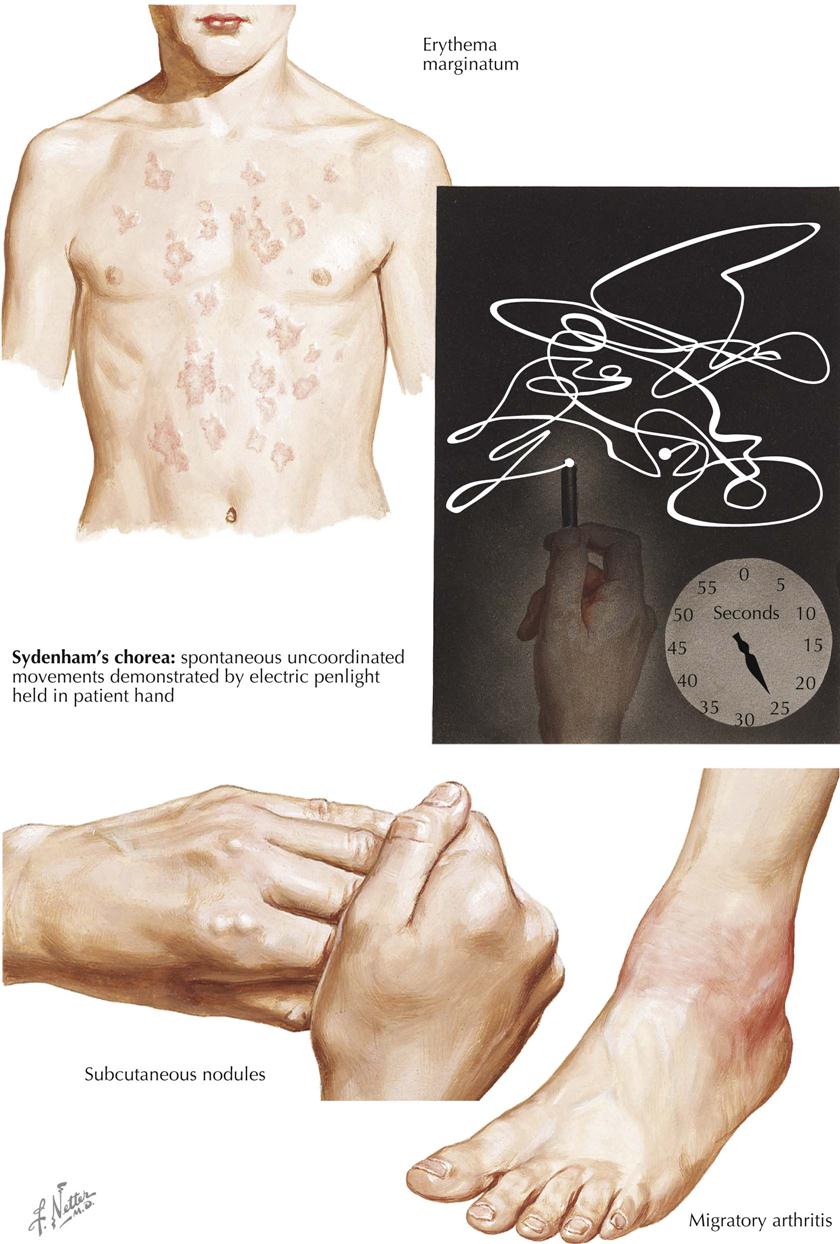
Despite the many features common to rheumatic fever and rheumatoid arthritis, notably polyarthritis and subcutaneous nodules, these are distinct nosologic entities. If differentiation is difficult in the early stages, the difference in clinical evolution rapidly distinguishes rheumatic fever from arthritis, and even in the early stages, histology of the synovium or biopsied nodule can be helpful. The lesion in rheumatic fever is capsular rather than synovial, with areas of fibrinoid necrosis accompanied by focal infiltrations of histiocytes and lymphocytes.
The joint lesions, even when severe, are entirely reversible, and permanent damage does not result. This contrasts with the cardiac lesions, which can occasionally progress to mitral stenosis and its sequelae, even when initial damage is mild. Skin rashes are uncommon but can be characteristic, especially erythema annulare, a fleeting, ring-shaped, usually flat eruption typically found on the trunk. Each lesion spreads centrifugally, leaving a slight staining in the center. Another typical feature of rheumatic fever, seen especially in severe cases, is subcutaneous nodules, mainly confined to the tissues over bony prominences, where friction combined with pressure is apparently responsible for nodule development. The nodules are most conspicuous after 2 to 3 weeks, although their impending development is often preceded by a more diffuse, boggy thickening. Histologically, a typical nodule shows an area of fibrinoid composed of parallel or interlacing bands sparsely infiltrated with histiocytes and fibroblasts. The adjacent tissue is edematous and contains groups of small vessels surrounded by similar cells. Fibrous tissue is inconspicuous.
Permanent cardiac damage correlates closely with persistent or recurrent activity of rheumatic disease, so the detection of such activity is important. The most helpful test is the erythrocyte sedimentation rate (ESR), which is almost never normal in the presence of active disease, except in patients with congestive heart failure. The converse, however—a persistently raised ESR—does not necessarily imply continued activity, especially in girls. Therefore, estimations of C-reactive protein may more accurately reflect the state of the disease activity. The greatest danger to the patient with a history of rheumatic fever is developing a new infection with β-hemolytic streptococci, because the risk of relapse is much greater than after an initial attack. The success of chemoprophylaxis has confirmed both the importance of such reinfection in the development of a relapse and the role of streptococci in rheumatic fever pathogenesis.
Environmental and genetic factors have been studied widely in an attempt to account for the considerable geographic variation in the incidence of rheumatic disease. The most impressive data are provided by Mills’ map, showing the progressive decrease in mortality from rheumatic fever as one passes from the northern to the southern latitudes of the United States. Evidence of genetic factors besides female predominance is less precise, but the increased incidence of nonsecretors of the blood group substances in patients with rheumatic fever indicates modest genetic influence. The dominant factor is environmental and related to the incidence and virulence of the local streptococci.
Sydenham’s Chorea
The rheumatic nature of Sydenham’s chorea (St. Vitus dance), first recognized just over 100 years ago, is now established by evidence similar to that for rheumatic fever, as a poststreptococcal state. This is confirmed by the frequent subsequent development of rheumatic heart disease, in the absence of any clinical evidence of rheumatic fever. The outstanding clinical features are ataxia, incoordination, and weakness, accompanied by spontaneous movements that can be most clearly depicted by the photographic record of a penlight held in the patient’s hand.
Rheumatic Heart Disease: Acute Pericarditis and Myocarditis
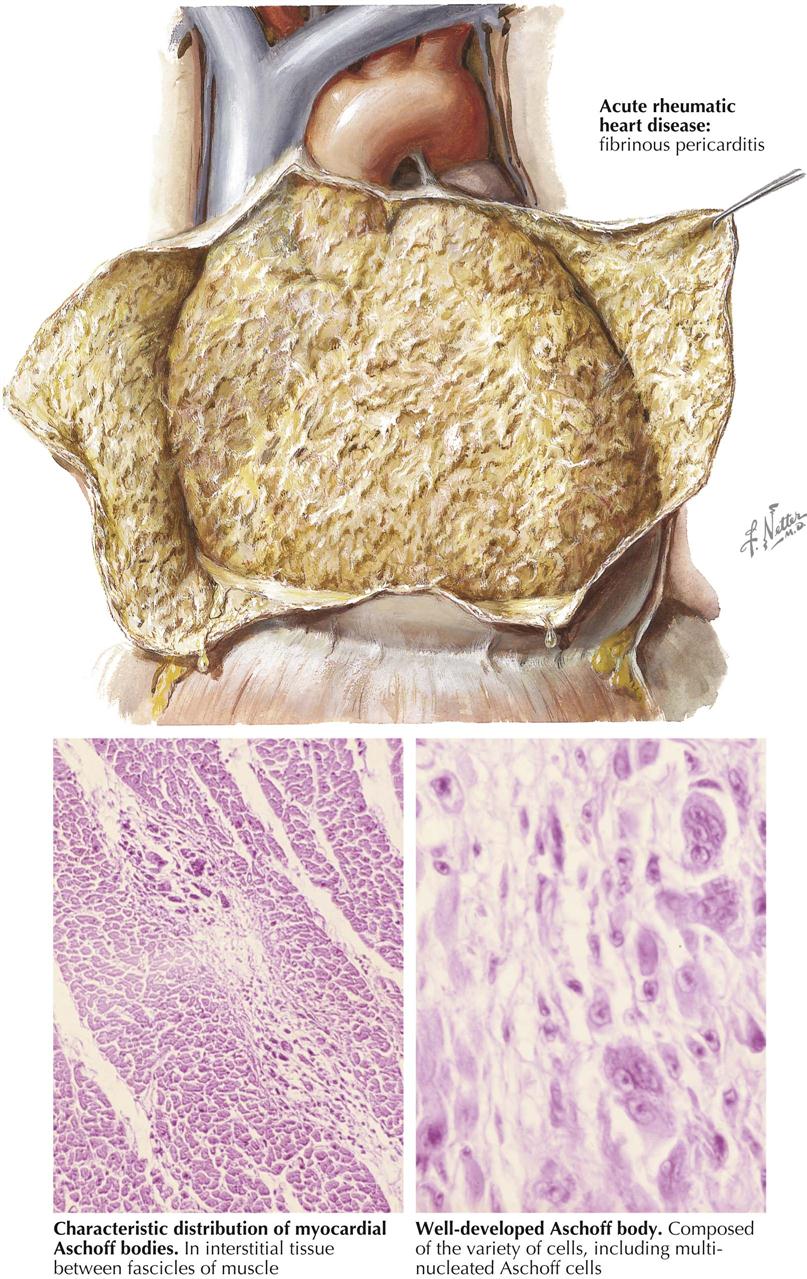
Rheumatic heart disease is a complication of recurrent upper-respiratory infection with group A β-hemolytic streptococci. Streptococcal products circulating from this source through the body causes a reaction in connective tissues called rheumatic inflammation. Predisposed sites include the heart, skin, and synovial membranes. In the latter two areas, inflammation usually heals by resolution, leaving no residual effects, but in the heart these recurrent respiratory infections may lead to severe deformities, usually of the valves.
Chronic rheumatic heart disease requires recurrent infections. Each attack of rheumatic inflammation goes through an active stage followed by healing. In the joints, active rheumatic involvement is characterized by migratory arthritis. In the skin, transitory subcutaneous nodules may appear. In the heart, each of the major anatomic components—the pericardium, myocardium, endocardium, and particularly the valves—may be involved.
Acute Rheumatic Pericarditis
Acute rheumatic pericarditis is characterized by variable exudation of serum and fibrin into the pericardial cavity. Large effusions of fluid may result in radiographic features of uniform cardiac enlargement and physical signs of pericardial effusion. Impairment of the inflow of blood to the heart, with resultant increased systemic venous pressure, may be evident through distention of the cervical veins. The fibrinous element of acute rheumatic pericarditis is manifested by particles of fibrin floating in the associated effusion and by the concentration of a fibrinous deposit on the visceral (epicardial) and parietal layers. The shaggy fibrin, evident when the two layers of the pericardium are separated, has given rise to the name “bread-and-butter heart” of rheumatic pericarditis. This gross feature is not specific, however, since it applies to any condition in which fibrinous pericarditis occurs. Histologically, during the active stage of rheumatic pericarditis, fibrin is deposited on the pericardial surfaces. Beneath this, capillaries and fibroblasts are mobilized and gradually enter the fibrin as granulation tissue. The pericardium shows edema and mild leukocytic infiltration. Specific Aschoff bodies, which characterize acute rheumatic myocarditis, are not usually seen in the pericardium.
Acute Rheumatic Myocarditis
The specific histologic lesion of acute rheumatic carditis is usually restricted to the myocardium and takes the form of the Aschoff body. Aschoff bodies are reactive nodules within the connective tissue and therefore are predominantly found around blood vessels of the myocardium and in other bundles of connective tissue that separate myocardial fascicles. The primary lesion appears to be an alteration of collagen, which shows a coagulation-like change with eosinophilia, a process called fibrinoid necrosis.
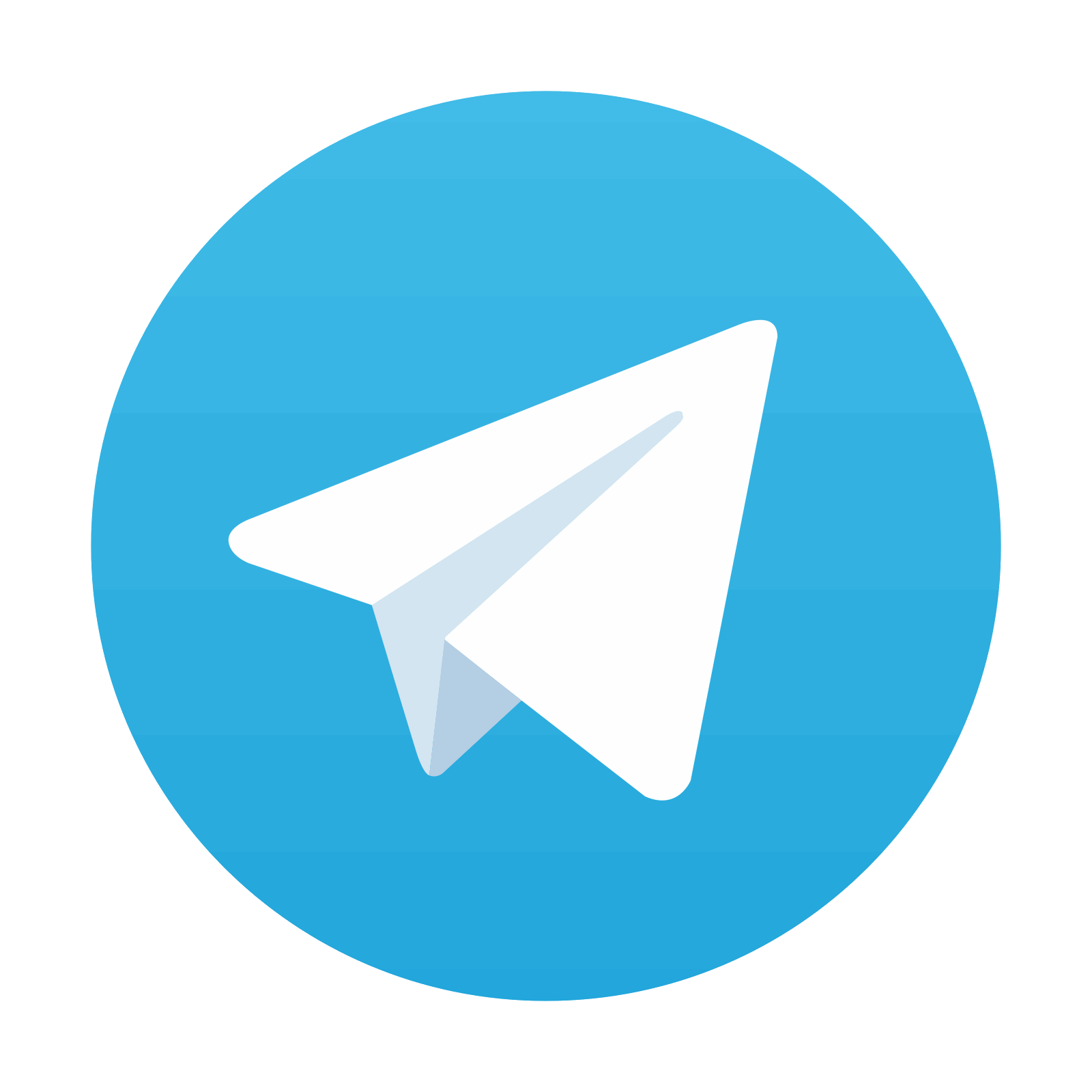
Stay updated, free articles. Join our Telegram channel

Full access? Get Clinical Tree
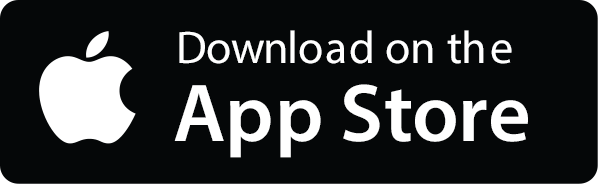
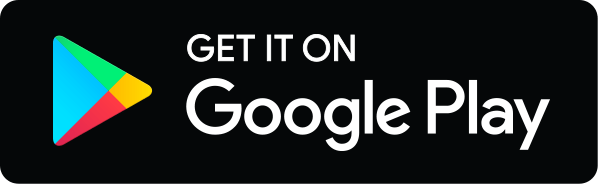