49 Contrast Staining Masquerading as Hemorrhage
49.1 Case
Utility of dual-energy CT after endovascular stroke treatment.
49.2 Case Description
49.2.1 Clinical Presentation
A 52-year-old male presented at 23:15 on Sunday night, 60 minutes after being discovered with an altered conscious state. Past medical history was significant for ischemic heart disease with cardiac stents and automatic implantable cardioverter-defibrillator. Examination in the emergency department reveals a Glasgow coma scale (GCS) score of 12 with some motion in all limbs.
49.2.2 Imaging Workup and Investigations
Initial noncontrast CT (NCCT) at 100 minutes post–stroke onset reveals no established ischemic changes with Alberta Stroke Program Early CT (ASPECTS) score of 10.
CTA reveals occlusion of the extracranial and intracranial left internal carotid artery (ICA) and middle cerebral artery (MCA; Fig. 49.1).
No CTP was performed at the time due to excessive motion artefact.
Delayed code stroke was activated as the emergency staffs were attempting to stabilize the patients’ agitated state, and CTP was performed.
CTP at 5 hours post–onset reveals perfusion abnormality in the left MCA territory, with elevated mean transit time and normal cerebral blood volume maps, suggesting the presence of a small core and large penumbra.
Examination by the stroke team revealed a National Institutes of Health Stroke Scale (NIHSS) score of 28. The patient was now outside the intravenous tissue plasminogen activator (IV-tPA) treatment timeframe. Endovascular treatment was contemplated.
Fig. 49.1 CTA coronal maximum intensity projection and 3D reconstruction demonstrate occlusion of the left internal carotid artery, extending to the carotid terminus, proximal left anterior cerebral artery, and into the left middle cerebral artery.
49.2.3 Diagnosis
Left ICA and MCA occlusion with a small core infarct size based on CT findings.
49.2.4 Treatment
Endovascular team was contacted, and repeat CT of the brain was performed at 6.5 hours (05:00) post–symptom onset with minimal core evident—ASPECTS score of 7 (Fig. 49.2).
Endovascular team in the hospital at 05:50 was unable to proceed with conscious sedation due to concerns regarding airway protection. Patient was intubated and moved onto the neuroangiography table at 06:50. Groin puncture occurred at 07:00.
Fig. 49.2 NCCT in a 52-year-old male, performed 6.5 hours after onset. There is loss of gray-white differentiation in the left insular cortex and left lentiform nucleus. Additional left inferior temporal change (not shown) with ASPECTS score of 7.
Endovascular Treatment
Initial digital subtraction angiography (DSA) revealed extensive thrombus burden, extending from cavernous ICA to the terminus and into both A1 and M1 MCA segments.
Multiple passes with Penumbra 054 for thromboaspiration, and Treveo stentriever.
Recanalization of the ICA, anterior cerebral artery (ACA), and MCA was achieved, with final thrombolysis in cerebral infarction (TICI) 2a reperfusion after 120 minutes of procedure time.
Posttreatment Imaging
Postprocedural dual-energy CT imaging at 12 hours post-onset reveals hyperattenuation in the left basal ganglia, left temporal lobe, and in the left sylvian fissure.
Since this could be due to post procedural hemorrhage, CT images with iodine subtraction are reconstructed (Fig. 49.3).
This confirmed that the hyperattenuation was due to contrast staining and not intracranial hemorrhage.
Fig. 49.3 Normal CT (top row) and water (bottom row) maps from dual-energy CT. On the normal CT, there is increased attenuation in the left caudate, lentiform nucleus and temporal lobe, as well as increased attenuation within the sylvian fissure and frontotemporal sulci. No increased attenuation is evident on the water maps (also known as “virtual non contrast”), indicating that the increased attenuation is attributable to contrast staining rather than hemorrhage. Note that these were the corresponding areas of ischemic change evident on the pretreatment CT, since these areas have disruption of the blood–brain barrier.
Outcome
Repeat CT the following day revealed some contrast washout, and delayed CT at 8 weeks revealed final infarct volume similar to initial CT scans (Fig. 49.4).
After 4 months, the patient was able to function independently with slight dysphasia and residual hemiparesis, with a mRS score of 2.
Fig. 49.4 NCCT at 8 weeks revealed final infarct volume similar to initial CT scans. Note that the encephalomalacia is limited to the areas with contrast staining from infarction prior to endovascular treatment.
49.3 Discussion
Dual-energy CT has ever increasing clinical application throughout the various imaging disciplines, including neuroradiology. The principle of dual-energy CT is the acquisition of two datasets for the same anatomical location with different kVp, allowing decomposition of the imaged object based on the different attenuation coefficients obtained at each kVp. There are three main techniques to facilitate dual-energy acquisition. First, two X-ray tubes may be positioned at right angles within the CT gantry, allowing simultaneous acquisition via two separate detector arrays, each X-ray tube operating at a different kVp. This technique enables the high-temporal resolution required for some clinical applications, but can limit the field of view. Second, a single tube may be utilized with a dual-layer detector array, with each layer optimized to absorb photons at higher or lower energy levels, and each subsequently reconstructed independently. Finally, a single X-ray tube may rapidly switch between the two desired kVp levels, paired with a single detector array.
Neuroradiology applications of dual-energy CT include generating “virtual” noncontrast images from data obtained during a contrast enhanced study; automatic “bone removal” during post processing; reduced beam hardening artefact; improved detection of underlying enhancing mass lesions in the setting of parenchymal hematoma; postprocessing removal of calcified carotid artery plaque for CTA; and differentiating between iodinated contrast media and parenchymal blood. 1 , 2 , 3 , 4 , 5 , 6 , 7 , 8 The last two applications are of particular interest to neurovascular and interventional neuroradiologists.
When referenced against DSA, dual-energy CTA with automated post processing “plaque removal” has good quantification of stenoses and superior accuracy for detection of relevant stenosis compared to standard CTA reconstructions. The “plaque removal” technique does have the potential to overestimate high-grade stenosis as occlusion, and is thus complementary to standard reconstructions rather than a replacement. 2 , 6
Use of dual-energy CT to differentiate between blood and iodinated contrast media may be useful in different clinical settings. Dual-energy CT allows differentiating between iodine and blood based on the differences in attenuation of each that occur at the two selected kVP levels. Postprocessed virtual noncontrast map (water map) and iodine maps may be viewed as standalone images, or fused onto source images. 9 , 10 , 11 , 12
Parenchymal hyperattenuation is frequently observed following intra-arterial therapy for acute ischemic stroke, representing blood, contrast staining, or a combination of both. 13 , 14 , 15 , 16 , 17 Early studies reported that the presence of parenchymal hyperattenuation after intra-arterial thrombolysis was a risk factor contributing to secondary hemorrhage and a poor prognostic factor. 14 , 15 More recently, the significance of parenchymal hyperattenuation after mechanical clot extraction has been evaluated with varied findings. In a series of 48 patients, parenchymal and/or subarachnoid hyperattenuation carried no risk of symptomatic hemorrhage or negative prognosis. 17 In a larger series of 101 patients, parenchymal hyperattenuation was associated with a four-fold risk of hemorrhagic transformation, but had no negative prognostic implication. 16 In a study of 50 patients, the presence of “blood–brain barrier disruption” was associated with a poorer outcome. 18 “Blood–brain barrier disruption” was defined as “spontaneous deposition of iodinated contrast medium in the parenchyma of the ischemic core,” but was “differentiated from contrast extravasation secondary to endovascular therapy complications.” Detail with regard to distinguishing between hemorrhage and contrast staining is lacking in this study, and the significance of the findings is uncertain with regard to the prognostic implication of parenchymal hyperattenuation. Regardless of prognosis, identifying hemorrhage and distinguishing it from contrast staining may have treatment implications by influencing the decision to anticoagulate, and identifying patients who may need early or closer imaging surveillance.
Distinguishing between parenchymal hyperattenuation and hemorrhage may be achieved with serial CT imaging. Contrast staining typically “washes out” within 24 to 48 hours, with hemorrhage persisting as increased parenchymal attenuation. Alternatively, MRI with blood sensitive sequences may be utilized. In the acute setting, if there is dense contrast staining, the attenuation of the contrast may exceed that of blood, allowing the presence of contrast staining to be established on conventional CT; however, an associated hemorrhage cannot be excluded. Less dense or heterogeneous contrast staining may appear identical to blood, and thus be indistinguishable on conventional CT. Dual-energy CT is emerging as a valuable tool to acutely distinguish between blood and contrast staining in the setting of intra-arterial therapy for acute stroke. Generating iodine and water maps allows contrast staining to be distinguished from hemorrhage, with each appearing as high attenuation on their relative maps, as well as in the assessment of relative amounts of each if they coexist. 4 , 5 A major advantage of dual-energy CT is that there is no need to delay or repeat imaging to determine if hemorrhage is present, and thus treatment decisions, particularly with regards to anticoagulation, can be instituted immediately. In addition, there is no increase in radiation dose over conventional CT. One pitfall with this technique is calcification, as this appears as increased attenuation on both water and iodine maps. In the current case, the iodine and water maps revealed that the increased parenchymal attenuation was due solely to contrast staining, with no hemorrhage present at the time of the posttreatment CT examination.
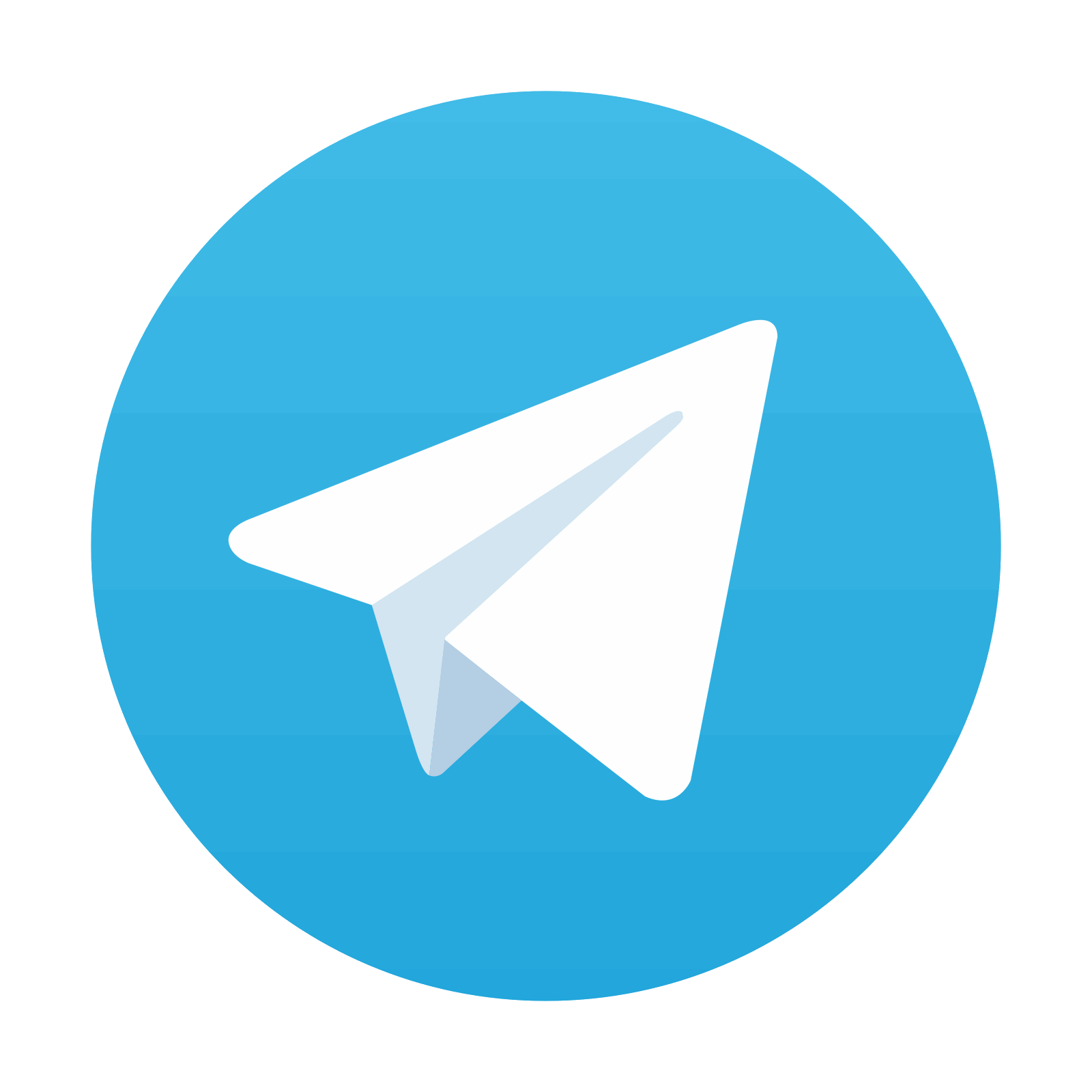
Stay updated, free articles. Join our Telegram channel

Full access? Get Clinical Tree
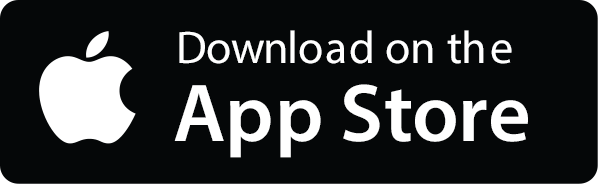
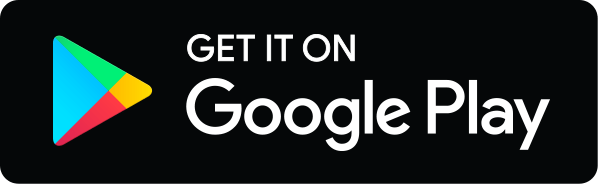