CHAPTER 3 Diseases of the lung are treated with a variety of drugs. In this chapter, the mechanisms of action, side‐effect profile, and interactions of the commonly used drugs are discussed. The clinical indications for the use of these drugs are described in more detail in the relevant chapters that follow. Obstructive airways disease is discussed in Chapter 6, diffuse parenchymal lung disease in Chapter 7, respiratory infections in Chapter 8, respiratory failure in Chapter 13, and sleep disorders in Chapter 14. Inhaled therapy has been used for centuries: sulphurs and volatile aromatic substances, such as methyl and eucalyptus, have been used to relieve respiratory symptoms for many years. An inhaler or a nebuliser will deposit the drug directly into the lungs where it is absorbed and works rapidly. Systemic side effects from inhaled therapy are less than with oral or intravenous treatment. All inhaler systems are relatively inefficient, with only 8–15% of the drug reaching the lung, no matter how good the inhaler technique is. Particle distribution within the lungs can be measured by radio‐labelling the drug and using a gamma camera to quantify deposition. The factors that determine particle deposition in the lungs include the size of the particle, the inspiratory flow rate, and the distance the particle needs to travel, which is determined by the method of inhalation. Factors that favour distal particle sedimentation include small size and low flow rate. An aerosol is a suspension of fine particles of varying sizes with a favourable surface‐to‐volume ratio, which allows a small dose to disperse widely over the airways and the alveolar surfaces (Figure 3.1). There is an optimal particle size which favours deposition. The mass median aerodynamic diameter (MMAD) of the aerosol is the diameter about which 50% of the total particle mass resides and this affects where most of the particles that enter the lung are deposited. Large particles of >6 μm in diameter are more likely to be deposited centrally, smaller particles <5 μm in diameter reach the smaller airways and those of 2–3 μm in diameter reach the alveoli. Particles which are even smaller than this may not settle and are expired. Drug deposition is enhanced by turbulent flow which predominates in these central passages, and particularly at airway bifurcations. Figure 3.1 Particle size and drug deposition. A faster inspiratory flow rate results in the particles being deposited more centrally because of inertial impaction. Slow inhalation with breath‐holding results in the particles reaching the peripheral and distal bronchioles. Particles deposited in the conducting airways, which stretch from the larynx to the terminal bronchioles, will become trapped in the mucociliary escalator (MCE). In healthy individuals, the MCE clears the particles within 6–24 hours after deposition, but the clearance will be delayed in conditions such as bronchiectasis, where there is ciliary damage. Small particles in the alveoli are cleared very slowly via alveolar macrophages and lymphatics. The solubility of the drug also affects how quickly the drug is absorbed and cleared from the lungs. The three main types of inhaler devices are pressurised metered dose inhalers (pMDI), dry powder inhalers (DPI), and soft mist inhalers (SMI) (Figure 3.2). Despite the differences in drug delivery to the lung with these various devices, no significant difference in bronchodilator effect has been found. Figure 3.2 Several types of inhaler devices. A pressurised metered dose inhaler (pMDI) can be used alone or with a spacer. It comprises of a canister, which can store up to 200 doses of the drug, and a plastic actuator. The drug in the small canister is either dissolved or suspended as crystals in a liquid propellant mixture of hydro‐fluoroalkane (HFA) which has replaced the chlorofluorocarbon (CFC) which is detrimental to the ozone layer. A low concentration of surfactant prevents aggregation of the small particles and acts as a lubricant. The patient should be instructed to shake the canister thoroughly, remove the cap, place the mouthpiece of the actuator between the lips, breathe out steadily, release the dose while taking a slow, deep breath in, hold the breath for a count to 10 and wait a minute before repeating. The use of the different inhalers and nebuliser is demonstrated in the supplementary video (www.wiley.com/go/ParamothayanEssential_Respiratory_Medicine) The pMDI has several advantages: it is portable, relatively cheap, and small doses of the drug can be given. However, the elderly and young children can find it difficult to use as co‐ordination is needed between actuation and inhalation. This can lead to poor compliance. Poor technique can result in deposition of the drug in the oropharynx rather than in the lungs. If inhaled corticosteroids (ICS) are being used, then oropharyngeal deposition can result in candidiasis and dysphonia. pMDI can be less effective in patients with significant airway obstruction as high inspiratory flow rates are required in this situation. It is generally recommended that the MDI is used with a spacer as this reduces oropharyngeal drug deposition and allows better penetration of the drug to the periphery of the lungs (Figure 3.3). Figure 3.3 Individual using an MDI. Dry powder inhalers (DPI) are breath‐actuated devices that contain a desiccant which ensures that the powder is kept dry. Most adults and children prefer these as they require less co‐ordination and are easier to use than a pMDI. The patient needs to be able to generate an inspiratory flow rate of at least 30 l min−1 to ensure adequate drug deposition in the lungs and to reduce oropharyngeal deposition. The turbohaler is the most commonly used DPI (Figure 3.4). It can hold 50–200 doses of the drug and a dose indicator gives a warning when only 20 doses remain. Patients are often concerned because they may not feel any sensation in their oropharynx when they inhale (Figure 3.5). Other DPI devices include the spinhaler, rotahaler, discs, and blisters. These devices are similar in their efficacy. Figure 3.4 Turbohaler. Figure 3.5 Patient using a turbohaler. A spacer device improves drug delivery and is recommended for use with all aerosol inhalers, including the pMDI. A large spacer with a one‐way valve is called a volumatic device (Figure 3.6). This increases the distance from the actuator to the mouth and allows the particles time to evaporate and slow down before inhalation. This results in a larger proportion of the particles being deposited in the lungs and minimises oropharyngeal drug deposition, thus decreasing the incidence of oropharyngeal candidiasis. Patients should inhale from the spacer device as soon as possible after a single actuation because the drug aerosol is very short‐lived. Tidal breathing is as effective as single breaths. The use of a large volume spacer is essential in young children and is an alternative to a nebuliser. The able spacer and aerochamber (Figure 3.7) are smaller volumatic devices which are more portable. Figure 3.6 Volumatic device (spacer). Figure 3.7 Aerochamber. The spacer should be cleaned once a month by washing in mild detergent and allowed to dry in air without rinsing. The mouthpiece should be wiped clean of detergent before use. More frequent cleaning should be avoided as this can affect the electrostatic charge and drug delivery. Spacers should be replaced every 6–12 months. Tube spacers are tube‐like attachments to the pMDI with a much smaller interval volume than the large volume spacers. They too enable the aerosol to slow down before reaching the mouth. Several studies have shown that in acute asthma, multiple doses of a bronchodilator given through a spacer have a similar bronchodilatory effect as if the drug is given through a nebuliser. However, a nebuliser has the advantage in that it can be used when the patient is very breathless and unable to make the inspiratory effort. A nebuliser (Figure 3.8) can deliver a higher dose of drug to the airways than an inhaler. A solution containing the drug, usually 1 mg ml−1, is turned into an aerosol for inhalation. Figure 3.8 Portable nebuliser. Nebulised short‐acting β2‐agonists (SABA) and anticholinergic medication are used to treat patients with exacerbation of asthma or COPD. Nebulised SABA can also be used to assess airway reversibility in patients with asthma and COPD. Nebulised methacholine and histamine can be used to assess bronchial hyper‐reactivity, and nebulised hypertonic saline can be used to induce sputum. Nebulised colomycin is used to treat pseudomonas aeruginosa infection associated with bronchiectasis and cystic fibrosis, and nebulised pentamidine can be used to treat pneumocystis jirovecii infection. Nebulised opiate can be given to relieve intractable breathlessness in the palliative care setting. There is no evidence for the use of nebulised steroids in exacerbations of asthma or chronic obstructive pulmonary disease (COPD). Jet nebulisers are more widely used than ultrasonic nebulisers. The jet nebuliser requires an optimum gas flow rate of 6–8 l min−1, which can be either piped air or oxygen. In patients who present with type 1 respiratory failure and require nebulised drugs, 6 l of oxygen should be used to drive the nebuliser. In patients who are at risk of type 2 respiratory failure, air should be used to drive the nebuliser. Supplemental oxygen can be given at the same time via a nasal cannula to maintain the oxygen saturation between 88% and 92%. Management of respiratory failure is discussed in Chapter 13. Many different designs of nebuliser chambers are available which produce aerosols with particles of different sizes, depending on the design of the baffles in the chamber and the gas flow rate. They usually hold 4–6 ml of solution and have a flow rate of 6–8 l min−1. Droplets with a MMAD of 1–5 μm are deposited in the conducting airways and are therefore suitable for treatment of asthma, whereas a particle size of 1–2 μm is needed for the alveolar deposition of pentamidine. Approximately 10% of a nebulised drug reaches the lungs, with most of the aerosol mist being wasted. The ultrasonic nebuliser delivers large particles of 3–10 μm from high frequency (1–2 mHz) sound waves induced by the vibration of a piezoelectric crystal which, when focused on the surface of a liquid, creates a fountain of droplets. It has less clinical use than the jet nebuliser. Long term oxygen therapy (LTOT) is indicated for patients with chronic type 1 respiratory failure with a resting PaO2 < = 7.3 kPa or those with a resting PaO2 < = 8 kPa with evidence of peripheral oedema, polycythaemia (haematocrit >55%) or pulmonary hypertension. LTOT improves survival in patients with respiratory failure by reducing the risk of developing cor pulmonale. Controlled LTOT is indicated for patients with type 2 respiratory failure, but must be prescribed with care and closely monitored as there is a risk of CO2 retention. The indications for oxygen therapy and principles of controlled oxygen are discussed in Chapter 13. LTOT is given through a concentrator for those requiring oxygen for more than 15 h day−1. The concentrator draws in air, filters out the nitrogen and concentrates the oxygen to reach 95% purity. The oxygen can be humidified to make it less drying to the nostrils (Figure 3.9). Other types of devices include oxygen reservoirs containing liquid oxygen or compressed oxygen. The percentage of inspired oxygen(FiO2) required is determined by measuring the arterial blood gas (ABG) on air and then on oxygen. The concentrator can be pre‐set to deliver the exact flow rate required. A back‐up cylinder of oxygen is also supplied for use in an emergency, for example during a power cut, and can supply oxygen for several hours. If a flow rate of more than 5 l min−1 is needed, then more than one concentrator may be required. Figure 3.9 Oxygen cylinder. Source: ABC of COPD, 3rd edition, Figure 11.7. Portable oxygen can be given for ambulant patients as bottled liquid oxygen which evaporates into the gas. Modern oxygen concentrators are light and portable and can be wheeled on a trolley. They have sufficient oxygen to last several hours and contain battery packs and electrical connections to charge them. Oxygen can be prescribed for patients with intractable dyspnoea in the palliative care setting. There is little evidence that short‐burst oxygen therapy (SBOT) is effective. Oxygen is flammable, so the patient and their family must be warned against the risks of smoking while on oxygen. Oxygen concentrators should be kept in a well‐ventilated area, away from gas stoves and flames. Inhaled drugs are primarily used to treat obstructive airways diseases, such as asthma, COPD, and bronchiectasis. The evidence and indications for the use of these drugs are discussed in Chapter 6. β2 ‐adrenoceptor agonists: the smooth muscle of the airways from the trachea to the terminal bronchioles has β2‐adrenoceptors. Direct stimulation of these receptors results in activation of adenylate cyclase and an increase in cyclic adenosine 3, 5 monophosphate (cAMP). The cAMP activates protein kinase A, which then phosphorylates several target proteins within the cell, resulting in the lowering of intracellular calcium concentration by the active removal of calcium from the cell into intracellular stores. Protein kinase A also inhibits phosphoinositide hydrolysis and myosin light chain kinase, resulting in the opening of the large‐conductance calcium‐activated potassium channels that repolarise the smooth muscle cell and stimulate the sequestration of calcium into intracellular stores. The overall effect is relaxation of the airway smooth muscle and bronchodilatation. Short‐acting β2 ‐agonists (SABAs) bind to the β2‐adrenoceptors and are effective bronchodilators with minimal side effects. β2‐agonists also have some anti‐inflammatory properties: they inhibit mediator release from mast cells, thus reducing the development of bronchial mucosal oedema after exposure to mediators such as histamine and leukotrienes. SABAs also inhibit the release of inflammatory peptides, such as substance P, from sensory nerves which contributes to bronchodilatation. They increase the mucus secretion from the submucosal glands and ion transport across the airway epithelium, thus enhancing mucociliary clearance. However, these short‐acting β2‐agonists do not have a significant inhibitory effect on the chronic inflammation of asthmatic airways. Salbutamol and terbutaline are the safest and most effective SABAs used for treating asthma with rapid improvement in breathlessness and wheezing (see Chapter 6). Salbutamol can be given at a dose of 100 μg/metered inhalation via a pMDI alone or with a volumatic device and through a nebuliser, 2.5 or 5 mg as required. In severe acute asthma, intravenous salbutamol could be considered, although careful cardiac monitoring would be required. Oral preparations of salbutamol may be used by patients who cannot manage the inhaled route, for example, children and the elderly. Terbutaline, also a SABA, is usually given via a turbohaler or nebulised at a dose of 5–10 mg, up to four times a day. It can also be given subcutaneously at a dose of 250–500 μg four times a day, or intravenously at a dose of 3–5 μg ml−1, which equates to 90–300 μg h−1 for 8–10 hours. Bambuterol, a long‐acting oral preparation and pro‐drug of terbutaline, may be of value in nocturnal asthma, but is rarely used. The onset of bronchodilatation occurs within minutes after inhalation of a SABA and the effect is sustained for 4–6 hours. Patients with asthma and COPD are advised to carry SABAs to be used when they become breathless. Their use can protect against various challenges such as exercise, cold air, and allergens. β2‐agonists are more effective in relieving breathlessness in asthma than in COPD as there is more reversibility in asthma. Patients with asthma who are only on SABA and are using it many times a day for symptom control should receive additional treatment, as monotherapy in asthma is associated with an increased risk of death. The main side effects of SABA, which are dose‐related, occur due to stimulation of the β‐adrenoceptors in the cardiac muscle and skeletal muscle, resulting in tachycardia (presenting with palpitations) and fine tremor, mainly of the hands. The selective β2‐agonists are associated with fewer side effects. Hypokalaemia can occur when β2‐agonists are given rapidly through a nebuliser, for example, in an acute exacerbation of asthma, because of the stimulation of potassium entry into skeletal muscle. The risk of hypokalaemia is increased when the patient is also being treated with theophylline, corticosteroids, and diuretics. β2‐agonists can also cause muscle cramps, headaches, paradoxical bronchospasm, urticarial angioedema, hypotension, and collapse. Tolerance can occur when the drug is given continuously due to down‐regulation of the receptor. Theophylline, which can be used in acute asthma and COPD, can also cause tachycardia, so patients who are receiving both drugs should be carefully monitored. Long‐acting β2 ‐agonists (LABAs) have a slightly slower onset of action than SABAs but the bronchodilator effect is sustained for 12 hours; therefore, the drug should be taken twice a day. Salmeterol is a partial agonist which is given at a dose of 6 or 12 μg and acts within 20 minutes. Formoterol has a more rapid onset of action and is licensed for short‐term symptom relief and for the prevention of exercise‐induced bronchospasm. LABAs should not be used for the relief of an asthma attack. It is recommended that formoterol and salmeterol are given in combination with ICS in asthma and COPD as these drugs act synergistically to improve symptoms, reduce exacerbations, reduce hospitalisation, and improve compliance. Preparations are available with different doses of each component so that patients can step the dose up or down as required. LABAs can rarely cause QT‐interval prolongation, taste disturbance, nausea, dizziness, rash, and pruritus. Short‐acting anticholinergic (SAA) drugs are specific antagonists of muscarinic receptors and inhibit cholinergic nerve‐induced bronchoconstriction, resulting in bronchodilatation. Normal airways have a resting vagal bronchomotor tone caused by tonic cholinergic nerve impulses which release acetylcholine (Ach) near the airway smooth muscle. Cholinergic reflex bronchoconstriction may be initiated by irritants, such as cold air and stress. This effect may be exaggerated in patients with COPD because of the fixed narrowing of the bronchi. Anticholinergic drugs, therefore, have a greater bronchodilator effect in COPD than in normal airways. SAAs protect against the acute effects of irritants, such as sulphur dioxide, inert dusts, and cold air by blocking cholinergic bronchoconstriction. Anticholinergics are ineffective against antigen‐induced or exercise‐induced bronchoconstriction because they have no effect on mast cells and have no anti‐inflammatory properties; they do not block the release of inflammatory mediators, such as histamine and leukotrienes. Anticholinergics are less effective bronchodilators than β2‐agonists in acute asthma and offer less effective protection against various bronchial challenges, although their duration of action is significantly longer. Anticholinergics are slower in onset than β2‐agonists, reaching a peak only 1 hour after inhalation, with effects persisting for more than 6 hours. They may be more effective in older patients with asthma who may have an element of fixed airway obstruction. In the treatment of acute and chronic asthma, anticholinergic drugs, when combined with β2‐agonists, may have an additive effect. Ipratropium bromide (atrovent) is a quaternary compound of atropine and a non‐selective anticholinergic that blocks the muscarinic M3 receptors in the smooth muscle of the airways. Ipratropium bromide can be given by pMDI at a dose of 20–40 μg three or four times a day in patients with COPD where it has some bronchodilator effect as well as reducing the amount of mucus production, thereby improving chronic cough. It can also be given in the nebulised form at a dose of 250–500 μg four times a day for acute asthma or acute exacerbation of COPD. It is topically active and not significantly absorbed from the respiratory tract, so systemic side effects are minimal. The side effects, which are secondary to the muscarinic, anticholinergic actions, include dry mouth, blurred vision, and urinary retention. Oxitropium bromide has a similar action to ipratropium bromide but is available in higher doses by inhalation. Its effects may be more prolonged so can be useful in some patients with nocturnal asthma. Long‐acting muscarinic agonist (LAMA) drugs cause bronchodilation, reduce bronchospasm and mucus production, and have a prolonged duration of action caused by slow dissociation from muscarinic receptors. They are licensed for use in COPD as first‐line agents and have the advantage that they only need to be taken once a day. They are also indicated for patients with chronic asthma. Tiotropium is given at a dose of 18 μg daily with a duration of action of 18–24 hours. Aclidinium bromide is also approved for use in COPD and is available as a dry powder. In trials, LAMAs have been shown to improve quality of life, reduce exacerbations and hospital admissions but with no evidence of a reduction in mortality. LAMAs can cause a dry mouth, blurred vision, closed‐angle glaucoma, urinary retention, cardiac arrhythmias, taste disturbance, dizziness, and epistaxis, but systemic side effects are rare because little systemic absorption occurs. Combinations of LABA, inhaled corticosteroid (ICS), and LAMA, improve compliance, maximise bronchodilation, improve symptoms, improve exercise capacity, improve quality of life, and reduce exacerbations in patients with COPD. Corticosteroids (CS) are the most effective and most commonly used drugs for the treatment of lung disease apart from antibiotics. They are potent anti‐inflammatory drugs which have a variety of different systemic effects. Glucocorticosteroid (GCS) receptors are found in most cells in the body. This receptor is bound to two molecules of heat shock protein 90 (HSP‐90) and 1 molecule of immunophilin. Binding of GCS to the receptor dissociates the receptor from the HSP‐90 and results in conformational changes of the receptor complex. The GCS‐receptor complex (Figure 3.10) binds to the promoter‐enhancer regions of target genes and up‐regulates or down‐regulates the gene and thereby the gene product through various pathways. Figure 3.10 Glucocorticoid receptor complex and mechanism of action of corticosteroid. Oral corticosteroids (OCS) have a high oral bioavailability and are rapidly absorbed across the epithelial lining of the gastrointestinal tract by diffusion. OCS are used in the treatment of exacerbation of asthma, COPD, and diffuse parenchymal lung diseases (DPLD), usually at a dose of 0.5–1 mg kg−1 day−1. OCS are also indicated for a variety of other conditions, such as sarcoidosis, allergic bronchopulmonary aspergillosis (ABPA), and vasculitis. Intravenous corticosteroids, such as methylprednisolone, are used to treat severe lung disease or when oral therapy is not possible, for example, when the patient cannot swallow or is vomiting. Cortisone and prednisone are pro‐drugs which require hydroxylation in the liver to the active compounds hydrocortisone and prednisolone. Prednisolone is more stable than cortisone, with twice the half‐life and a much higher affinity for the glucocorticosteroid receptor. Dexamathasone is 25 × times more potent than hydrocortisone (Box 3.1).
Pharmacology of the lung
Abbreviations
Drugs and the lung
Principles of drug deposition in lungs
Inhaler devices
Oxygen
Inhaled drugs
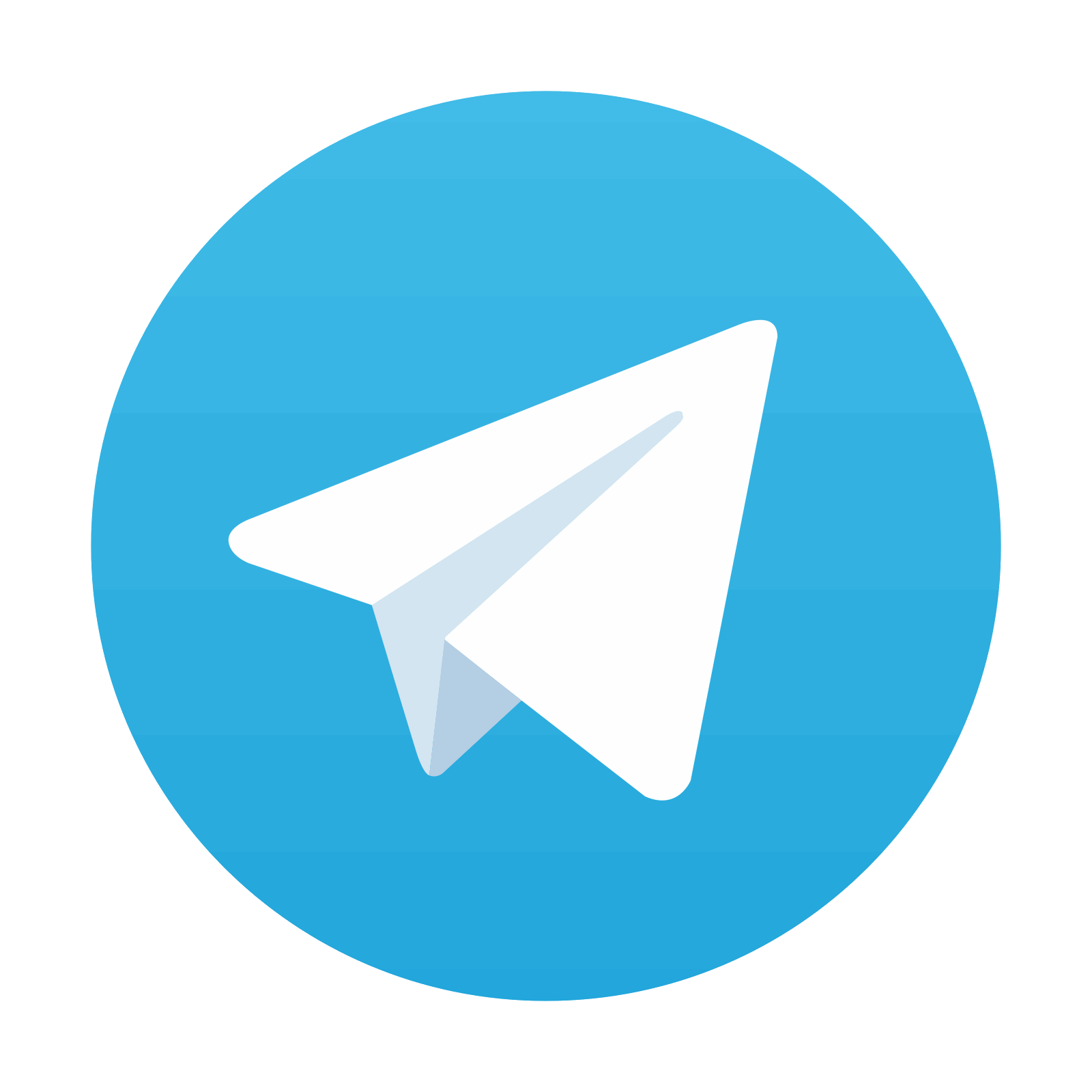
Stay updated, free articles. Join our Telegram channel

Full access? Get Clinical Tree
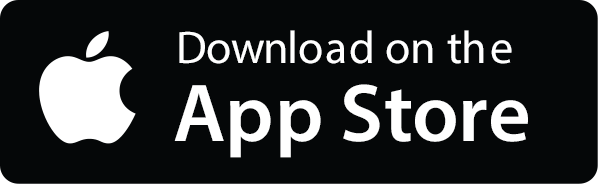
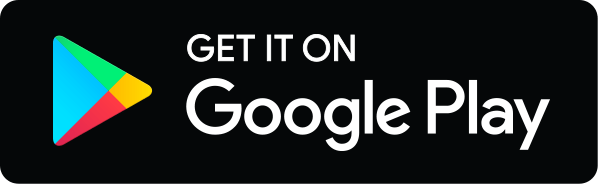