The urinary system, also known as the renal system or urinary tract, consists of the kidneys, ureters, bladder, and the urethra, and its main function in the human body is the production, storage, and eventual removal of urine, the fluid waste excreted by the kidneys. The kidneys are complex organs of the urinary system considered vital in maintaining body physiology. A human being’s survival depends, to a large degree, on the crucial functions and processes performed by the kidneys, which aim at maintaining a stable internal environment (homeostasis) for optimal cell and tissue metabolism. These include: i) the regulation of plasma osmolarity by modulating the amount of water and electrolytes (e.g., sodium, potassium, phosphates) in the blood; ii) the removal of metabolic waste products (e.g., nitrogenous substances) and foreign substances (e.g., drugs) from the body through urine; iii) the regulation of long‐term acid‐base balance by modulating the excretion of hydrogen ions and the reabsorption of bicarbonate; iv) the production of several important hormones, such as erythropoietin, which stimulates the production of red blood cells and renin, which is involved in blood‐pressure regulation; and v) the conversion of vitamin D to its active form, which is crucial for intestinal calcium absorption (Ogobuiro & Tuma, 2020). Kidney disorders can develop suddenly (acute) or over a long period (chronic). Acute kidney injury (AKI) is the sudden loss of kidney function over a period of hours or days. It is usually caused by an event that leads to kidney malfunction, such as severe dehydration, blood loss from major surgery or injury, or improper use of potentially nephrotoxic medication. It is considered reversible given that a prompt workup of the underlying cause is pursued and proper care is implemented (e.g., optimization of volume status and avoidance of nephrotoxic substances) (Moore, Hsu, & Liu, 2018). On the other hand, chronic kidney disease (CKD) is the gradual loss of kidney function over a period of years or even decades, usually caused by a long‐term medical condition and aggravated by risk factors. CKD encompasses a wide spectrum of renal dysfunction that ranges from a mild loss of kidney function to renal failure, also called end‐stage renal disease (ESRD), which represents the most common form of kidney disease. CKD is now recognized as a major cause of morbidity and mortality, and its efficient management remains a challenge for the medical community (Bikbov, Perico, Remuzzi, & on behalf of the, 2018). CKD refers to the progressive and irreversible decline in renal function and is defined as the presence of either chronic impaired renal clearance capacity or chronic kidney damage (National Kidney, 2002; Stevens, Levin, & Kidney Disease: Improving Global Outcomes Chronic Kidney Disease Guideline Development Work Group, 2013). Renal clearance capacity refers to the rate at which kidneys filter blood, while kidney damage refers to either functional abnormalities of the kidneys (such as proteinuria or abnormalities of the urinary sediment, such as dysmorphic red cells) or structural abnormalities of the kidneys as noted on imaging studies (National Kidney, 2002; Stevens et al., 2013). In clinical practice, the ability of the kidneys to clear blood from waste products and to reserve proteins are combined to determine CKD presence, severity, and risk of progression (Vart & Grams, 2016). The clearance capacity of the kidneys is typically expressed through the glomerular filtration rate (GFR), a measure of how much blood (in mL) is “cleaned” by the kidneys per minute. In the absence of methods to directly measure the number of nephrons and single‐nephron GFR, overall GFR is estimated indirectly from the clearance rate of exogenous markers, such as inulin, or endogenous markers, such as creatinine and cystatin C, using appropriate equations that take body size into account, assuming that the average adult body size is 1.73 m2. Estimated GFR is expressed as mL/min/1.73 m2 and low values are indicative of impaired renal clearance efficiency (Vart & Grams, 2016). The protein reservation capacity of kidneys is typically estimated through the degree of proteinuria, a condition in which protein abnormally leaks into urine. Given that albumin is the most abundant high molecular‐weight protein in plasma, the albumin:creatinine ratio (ACR) is the dominant marker of the abnormal leakage of albumin into urine (albuminuria). ACR is expressed in mg/g or mg/mmol, and high values are indicative of a pathologic alteration of the glomerular filtration barrier and an impaired kidney protein reabsorption function (Vart & Grams, 2016). In general, CKD is defined as the presence of decreased kidney function with GFR <60 mL/min/1.73 m2 or kidney damage with an ACR ≥30 mg/g or ≥3 mg/mmol for 3 or more months (National Kidney, 2002; Stevens et al., 2013). The diagnosis, staging, and progression risk assessment of CKD based on the categorization of GFR and ACR values is summarized in Table 20.1 (Levey et al., 2011). Table 20.1 Diagnosis and prognosis of CKD based on the criteria of kidney disease improving global outcomes (KDIGO). ACR = albumin:creatitine ratio; GFR = glomerular filtration rate. Source: (Levey et al., 2011). CKD is a global health burden with a high economic cost to health systems. According to the available epidemiological studies, the mean global CKD prevalence is estimated as high as 13.4% (11.7–15.1%), of which 3.5% (2.8–4.2%) corresponds to stage 1 (GFR > 90 mL/min/1.73 m2 + ACR > 30 mg/g), 3.9% (2.7–5.3%) corresponds to stage 2 (GFR 60–89 mL/min/1.73 m2 + ACR > 30 mg/g), 7.6% (6.4–8.9%) corresponds to stage 3 (GFR 30–59 mL/min/1.73 m2), 0.4% (0.3–0.5%) corresponds to stage 4 (GFR 29‐15 mL/min/1.73 m2), and 0.1% (0.1–0.1%) corresponds to stage 5 CKD (GFR < 15 mL/min/1.73 m2) (Hill et al., 2016). In 2016, the global number of individuals with CKD reached 752.7 million, of which 417.0 million were women and 335.7 million were men, while the most prevalent form of CKD in both genders is albuminuria (ACR > 30 mg/g) with preserved GFR (Bikbov et al., 2018). The most common causes of CKD are diabetes mellitus and hypertension, which are responsible for up to two‐thirds of the cases (Drawz & Rahman, 2015). Other risk factors for CKD include autoimmune diseases, systemic infections, urinary tract infections, nephrolithiasis, lower urinary tract obstruction, hyperuricemia, AKI, and a family history of CKD as well as sociodemographic and lifestyle factors, such as smoking, heavy alcohol consumption, being an older adult, Black, and/or overweight/obese as well as the use of nonsteroidal anti‐inflammatory drugs (Drawz & Rahman, 2015). Diabetes mellitus is a group of metabolic diseases characterized by hyperglycemia resulting from defects in insulin production, insulin action, or both (“2. Classification and Diagnosis of Diabetes: Standards of Medical Care in Diabetes‐2019,” 2019). It is a well‐established risk factor for kidney injury that can eventually lead to the development of diabetic kidney disease (DKD) through mechanisms that include hyperaminoacidemia, a promoter of glomerular hyperfiltration, and hyperperfusion as well as hyperglycemia, which induces chronic inflammation and oxidative stress through an increased production of advanced glycosylation end products, reactive oxygen species, and inflammatory cytokines (Alicic, Rooney, & Tuttle, 2017). DKD develops in approximately 40% of patients who are diabetic and is the leading cause of CKD worldwide. If poorly treated, DKD can eventually lead to ESRD, however, the majority of DKD patients die from cardiovascular diseases and infections related to diabetes before reaching ESRD. Therefore, optimal glycemic control is crucial for all DKD patients to reduce the progression of CKD, the incidence of ESRD, and mortality risk (Alicic et al., 2017). Hypertension (systolic/diastolic pressure ≥140/90 mm Hg) and CKD are closely interlinked, given that sustained hypertension can lead to the deterioration of kidney function and that a decline in kidney function can conversely lead to poor blood‐pressure control (Hamrahian, 2017; Ku, Lee, Wei, & Weir, 2019). It is well established that hypertension is the second leading cause of CKD worldwide after diabetes mellitus and accounts for approximately 30% of all ESRD patients. The leading theory explaining this relationship is that systemic hypertension is transmitted to intraglomerular capillary pressure, which leads to glomerulosclerosis and gradual loss of kidney function. However, the pathophysiology of hypertension in CKD is complex and is a sequela of multiple factors, including reduced nephron mass, increased sodium retention and extracellular volume expansion, sympathetic nervous system overactivity, activation of the renin‐angiotensin‐aldosterone system, and endothelial dysfunction. Regardless of the cause‐effect relationship of the two entities, hypertension is highly prevalent in CKD patients and its efficient management is of high importance, as it can help toward both slowing CKD progression and reducing cardiovascular risk (Hamrahian, 2017; Ku et al., 2019). Mild CKD (estimated GFR >60 mL/min/1.73 m2) is usually an asymptomatic disorder with no physical findings specific to decreased kidney function. On the contrary, advanced CKD is associated with serious complications, including cardiovascular disorders, cachexia, cognitive dysfunction, and metabolic abnormalities such as anemia, dyslipidemia, renal osteodystrophy, secondary hyperparathyroidism, and electrolyte disturbances (Thomas, Kanso, & Sedor, 2008). Most importantly, advanced CKD is recognized as a major cause of mortality. As shown in Figure 20.1, according to a 2010 collaborative meta‐analysis of 14 general population cohorts with 105,872 participants (Matsushita et al., 2010), a GFR <60 ml/min/1.73 m2 and ACR ≥10 mg/g were highlighted as independent predictors of mortality risk in the general population. Notably, this increased risk for all‐cause mortality is largely driven by cardiovascular deaths, which constitute approximately 60% of the deaths in CKD patients (Tonelli et al., 2006). Cardiovascular disorders in CKD include: (a) atherosclerosis, which predisposes the patient to ischemic cardiac events (myocardial infarction/angina), cerebrovascular events (strokes, transient ischemic attacks), and peripheral vascular events (gangrene, amputation); (b) arteriosclerosis, which predisposes the patient to left ventricular hypertrophy and ischemic heart disease; (c) cardiomyopathy is associated with disorders of cardiac structure (concentric and eccentric hypertrophy) and function (diastolic and systolic dysfunction), which predispose the patient to pulmonary edema; (d) arrhythmogenic disorders resulting in atrial fibrillation and sudden cardiac death; and (e) cardiac valvular disorders, particularly affecting the aortic and mitral valves (Parfrey & Barrett, 2015). The close relationship between CKD and cardiovascular diseases can be explained by both traditional and non‐traditional cardiovascular risk factors that are present in most CKD patients. Traditional risk factors include smoking, sedentariness, obesity, hypertension, dyslipidemia, and diabetes mellitus, while non‐traditional risk factors are mainly related to uremia, i.e., high levels of urea in the blood due to insufficient excretion in urine and include low levels of hemoglobin, albuminuria, and related malnutrition (loss of lean body mass) as well as abnormal bone and mineral metabolism (Alani, Tamimi, & Tamimi, 2014). Interestingly, it has been shown that the heart and the kidneys are closely linked, with the disease of one organ causing dysfunction of the other, and ultimately leading to the failure of both, a condition known as cardio‐renal syndrome. Cardiovascular involvement in CKD is known as type‐4 cardio‐renal syndrome or chronic reno‐cardiac syndrome, a condition characterized by the hemodynamic cross‐talk between failing kidneys and the heart as well as by significant alterations in neurohormonal markers and inflammatory molecular signatures that derive from the pathophysiology of CKD and result in a highly increased cardiovascular risk (Figure 20.2) (Ronco, Haapio, House, Anavekar, & Bellomo, 2008). FIGURE 20.1 All‐cause and cardiovascular mortality according to eGFR and ACR adjusted for each other, age, gender, race, cardiovascular history, systolic blood pressure, diabetes, smoking, and total cholesterol levels. The reference categories are GFR 95 ml/min/1.73 m2 and ACR 5 mg/g (0.6 mg/mmol). Dots and triangles represent statistically significant and non‐significant differences compared to reference categories, respectively. ACR = albumin:creatinine ratio; CVD = cardiovascular disease; eGFR = estimated glomerular filtration rate. Source: (Matsushita et al., 2010). Given the complex nature and complications of CKD, optimal disease management is best accomplished through a collaboration between different medical specialties in the context of a multidisciplinary team. In the early stages of CKD, the main goals of treatment include slowing the decline in kidney function and the likelihood of reaching ESRD, preventing the establishment of cardiovascular diseases, and treating CKD metabolic complications. To accomplish these goals, dietary protein intake should be <1.0g/kg/d, blood pressure should be closely monitored and kept within normal range in patients with hypertension, and glycemia should be controlled in patients with diabetes mellitus using available therapies (Drawz & Rahman, 2015). In ESRD, treatment should facilitate a transition to renal replacement therapy, usually advised to start once the estimated GFR is <15 mL/min/1.73 m2 and the patient is symptomatic. Common indications to initiate renal replacement therapy are volume overload unresponsive to diuretics, pericarditis, uremic encephalopathy, major bleeding secondary to uremic platelets, and hypertension that does not respond to treatment. Hyperkalemia and metabolic acidosis that cannot be managed medically and progressive “uremic” symptoms, such as fatigue, nausea and vomiting, loss of appetite, and evidence of malnutrition, are also indications for renal replacement therapy. Renal replacement therapy options include dialysis, i.e., the process of removing excess water, solutes, and toxins from the blood in the form of hemodialysis, peritoneal dialysis, hemofiltration, hemodiafiltration, or intestinal dialysis as well as renal transplantation (Hakim & Lazarus, 1995). Nutritional therapy in CKD is of high importance. As already mentioned, CKD is progressive, irreversible, and affects various metabolic pathways. Altered energy homeostasis, abnormal protein catabolism, and acid‐base derangements are typical manifestations of the disease. As CKD progresses, nutritional status deteriorates and protein‐energy wasting occurs, a condition that requires dietary adjustments. Reasons for the establishment of protein‐energy wasting in the CKD population include: the accumulation of nitrogen‐containing products from dietary and intrinsic protein catabolism in the body that may distort taste and smell and blunt patients’ appetite, which results in insufficient energy intake; coexisting medical conditions, and frailty, particularly in elderly patients who account for a large proportion of those who are affected by CKD; abnormal gastrointestinal nutrient absorption due to uremia, which affects the microbiome and disrupts intestinal epithelia. FIGURE 20.2 Pathophysiological interactions between heart and kidney in type 4 cardio‐renal syndrome or “chronic renocardiac syndrome”. BMI = body‐mass index; CKD = chronic kidney disease; EPO = erythropoietin; LDL = low‐density lipoprotein. Source: (Ronco et al., 2008). However, beyond dietary adjustments to prevent or manage malnutrition, nutritional therapy may also help manage uremia and other CKD complications, such as electrolyte imbalances, water and salt retention, and bone disorders. Given the high prevalence of CKD and considering the exceptionally high costs and burden of maintenance dialysis therapy and kidney transplantation, dietary interventions are increasingly chosen as a conservative management strategy for the disease. Nutritional management of CKD is generally focused on dietary protein, sodium, potassium, and phosphorus and aims at both managing CKD complications and achieving an optimal nutritional status. A synopsis of the dietary guidelines for the management of CKD is presented in Table 20.2 (Kalantar‐Zadeh & Fouque, 2017). Table 20.2 Dietary guidelines for the management of CKD. BMI = body‐mass index; CKD = chronic kidney disease; EAA = essential amino acids; GFR = glomerular filtration rate; HBV = high biologic value; KA = ketoacids; LPD = low‐protein diet; PEW = protein‐energy wasting. Source: (Kalantar‐Zadeh & Fouque, 2017). The optimal protein intake for patients with CKD is probably one of the most debated and challenging issues in disease management, given that the quantity and quality of ingested protein has been proposed as a risk factor for CKD. On the one hand, experimental evidence suggests that long‐term dietary protein intake exceeding 1.5 g/kg/d may cause glomerular hyperfiltration and proinflammatory gene expression (Hostetter, Meyer, Rennke, & Brenner, 1986; Tovar‐Palacio et al., 2011), which are known risk factors for CKD. On the other hand, a very low‐protein intake can aggravate muscle wasting and contribute to both uremia, due to increased intrinsic protein catabolism, and severe malnutrition, given that CKD patients often suffer from cachexia (Wright, Southcott, MacLaughlin, & Wineberg, 2019). Altogether, the current evidence suggests that the ideal protein intake for CKD patients can vary from 0.6 to 0.8 g/kg/d for adults with moderate‐to‐advanced CKD (GFR <45 ml/min/1.73 m2) and those with substantial proteinuria (urinary protein excretion > 0.3 g/d) to 1.2 to 1.4 g/kg/d for CKD patients on dialysis or with malnutrition aiming at preventing or correcting protein‐energy wasting. In either case, about half of the protein ingested should be of “high biologic value” (e.g., from dairy products and lean meat) and adequate energy (30–35 kcal/kg/d) along with ongoing nutritional education should be provided to preserve muscle mass (Kalantar‐Zadeh & Fouque, 2017). Dietary sodium restriction is also an integral part of CKD nutritional management to control fluid retention, hypertension, and to improve patients’ cardiovascular risk profile. Observations in the general population suggest a J‐shaped association between sodium intake and cardiovascular risk, with an intake of >5 g/d or <3 g/d being associated with an increased risk of cardiovascular disease and death (Stolarz‐Skrzypek et al., 2011). Although a daily dietary allowance <2.3 g of sodium (<100 mmol) is often recommended for patients with cardiovascular disease, there is no evidence that patients with CKD will benefit from this strict sodium restriction, given the risk of hyponatremia, worsening of nutritional status due to an unpalatable diet, and adverse cardiovascular outcomes. Therefore, a daily dietary sodium intake of <4 g (<174 mmol) is recommended for the overall management of CKD and its associated risks, and a sodium intake <3 g (<131 mmol) is recommended for CKD patients with symptomatic fluid retention, severe hypertension, or proteinuria (Kalantar‐Zadeh & Fouque, 2017). A sodium restriction <3‐4 g/d is translated into a diet characterized by a minimum consumption of packaged products (e.g., salty snacks and pastries), processed foods (e.g., cold cuts), or pre‐cooked meals, a modest consumption of dairy products, grains, and meat, and allows for the addition of approximately 1 teaspoon of salt during cooking daily. Dietary potassium is also an important target of the nutritional management of CKD, given that hyperkaliemia frequently occurs in patients with advanced disease and can be lethal. A modest dietary potassium restriction is therefore beneficial for most CKD patients. However, a very low dietary potassium intake can expose patients to unintended side‐effects, such as an unhealthy atherogenic diet, given that many potassium‐rich foods (e.g. fresh fruits and vegetables) are high in dietary fibers and vitamins and generally considered an integral part of a cardio‐protective diet, and constipation, which may actually result in higher gut potassium absorption (Khoueiry et al., 2011; St‐Jules, Goldfarb, & Sevick, 2016). Given the aforementioned, patients with mild‐to‐moderate CKD should aim for a potassium intake similar to that of the general population (4.7 g/d), while in patients with a tendency toward hyperkalemia (>5.5 mmol/L), a dietary potassium intake of <3 g/d (<77 mmol/d) is recommended, with the condition that a balanced intake of fresh fruits and vegetables with high fiber is not compromised (Kalantar‐Zadeh & Fouque, 2017). To achieve this goal, CKD patients must be educated on the dietary sources of potassium, techniques to lower the natural potassium content of foods (e.g., peeling, cutting in smaller pieces, and changing water during cooking), and how to balance high‐ and low‐potassium foods in their meals when they design their daily dietary plan. Regarding phosphorus, hyperphosphatemia is also common in kidney failure, however a modest protein intake (0.8 g/kg/d) is generally equivalent to a diet with a modest phosphorus content, given that most high‐protein foods, such as dairy and meat products, are also rich in phosphorus. Restricting dietary phosphorus intake to <800 mg/d (26 mmol/d) is recommended for patients with moderate‐to‐advanced CKD, this requires a modest fish consumption (no more than 1 serving/week) and the limitation of processed foods, in which food additives include readily absorbable inorganic phosphorus that can lead to a high phosphorus burden (Kalantar‐Zadeh & Fouque, 2017). It should be noted that in dialysis, for patients who are at increased risk for protein‐energy malnutrition, a strict reduction of protein intake to control hyperphosphatemia may be associated with poor outcomes and is therefore contraindicated (Lynch, Lynch, Curhan, & Brunelli, 2011). In this case, an individualized approach that incorporates the optimal use of phosphorus binders with meals that contain phosphorus (usually main meals, i.e., breakfast, lunch, and dinner), and the avoidance of phosphorus‐rich snacks throughout the day is crucial for the optimal management of CKD (Tonelli, Pannu, & Manns, 2010). Other important aspects of the nutritional management of CKD include an appropriate calcium intake, which in advanced CKD, is lower than that recommended for the general population (increased calcium release from bones in hyperactive renal bone disease is can cause a positive calcium balance that may worsen vascular calcification), an adequate vitamin D intake to manage renal osteodystrophy, an adequate intake of unrefined carbohydrates from plant‐based foods to prevent constipation and promote a more favorable microbiome, a healthy dietary lipid profile with emphasis on mono‐ and poly‐unsaturated fatty acids over saturated fatty acids, a reduced protein intake with a greater proportion of diet from plant‐based foods to correct acidosis as well as the assessment and individualized correction of micronutrient deficiencies (e.g., iron, zinc, copper, folate, vitamin K, and vitamin D) through supplementation (Kalantar‐Zadeh & Fouque, 2017). CKD patients are characterized by low levels of physical activity and markedly poor exercise capacity. Decreased physical activity and fitness in CKD patients represent the beginning of a vicious cycle of declining physical function and disability, which, if left uncontrolled, continues to escalate progressively and negatively affects all aspects of the disease, including kidney failure, cardiovascular risk, and mortality (Figure 20.3) (P. Painter & Roshanravan, 2013). Regarding physical activity, CKD patients can be characterized as a highly sedentary population. Most available data refer to dialysis patients, who have been shown to fail to meet physical activity guidelines and norms of the general population (Avesani et al., 2012; Johansen et al., 2010) and to report a significantly lower level of physical activity, assessed through accelerometers or pedometers, compared with gender‐ and age‐matched healthy controls (Baria et al., 2011; Johansen et al., 2000; Zamojska, Szklarek, Niewodniczy, & Nowicki, 2006). It is worth mentioning that although data are insufficient for pre‐dialysis patients, Agarwal et al. (Agarwal & Light, 2011) showed that actimetry‐assessed physical activity level decreases as CKD progresses, with dialysis patients being the most sedentary, followed by pre‐dialysis patients, and healthy controls. Although the etiology of inadequate physical activity in CKD has not been fully elucidated, possible explanations include: (a) the metabolic and nutritional consequences of the disease, such as fluid and electrolyte imbalances, uremia, anemia, bone disease, and malnutrition that combined lead to impaired muscle function and fatigue; (b) the presence of co‐morbidities, such as obesity, hypertension, diabetes mellitus, and cardiovascular diseases that can contribute to reduced exercise capacity; (c) renal replacement therapy for ESRD patients, possibly due to the period of inactivity for the dialysis procedure itself (typically includes three 4‐hour sessions per week), and to the post‐dialysis fatigue syndrome often observed in ESRD patients; and (d) fear of injury or aggravating the disease, lack of guidance from healthcare professionals, and lack of local exercise facilities, which are reported by patients as barriers to exercise (Clarke et al., 2015; Roshanravan, Gamboa, & Wilund, 2017). Regarding physical fitness, several cross‐sectional studies clearly demonstrate that peak oxygen consumption (VO2max) is significantly lower in CKD patients compared with population norms or with healthy control groups, averaging 50% to 80% of healthy levels (Johansen & Painter, 2012). This low VO2max level suggests that most patients with CKD do not have the exercise capacity to sustain even simple lifestyle activities, such as housework and shopping, and that most of them meet criteria for cardiovascular disability. Besides VO2max, performance in several other fitness tests, including hand‐grip strength, leg extension strength, symptom‐limited test, sit‐to‐stand test, timed up‐and‐go test, 400‐m walk, and comfortable and maximal 6‐min‐walking test, has been found significantly reduced in CKD patients compared to population norms, indicating that the CKD population is characterized by low muscle mass and strength and poor physical functioning (Brodin, Ljungman, & Sunnerhagen, 2008; Clyne, Jogestrand, Lins, & Pehrsson, 1994; Odden et al., 2006; Padilla et al., 2008). In addition, the few available prospective studies provide evidence of a gradual decrease in fitness indices, such as VO2max, gait speed, and muscle area and density as CKD severity advances (Leikis et al., 2006; Liu et al., 2014; Roshanravan et al., 2015). Several CKD‐related factors, such as uremic toxins, vitamin D deficiency, hyperparathyroidism, metabolic acidosis, and anemia have been proposed as causes of muscle wasting, altered neuromuscular function, and poor fitness in the CKD population, however, advanced age, the presence of co‐morbidities, and low physical inactivity level are also possible mediators (Johansen & Painter, 2012). FIGURE 20.3 The impact of CKD on the components of functioning as described in the International Classification of Function Framework. The health condition and co‐morbidities associated with CKD, such as sarcopenia, vascular dysfunction, inflammation, and malnutrition affect the three components of functioning: body functions and structures (at the level of body or specific organs and functioning of those systems); activity (the individual level—ability to ambulate, perform physical tasks); and participation (the whole person in their environment—participation in physical activity, ability to participate in life activities). Contextual factors of environment and personal factors affect both activity and participation components. ADL = activities of daily living; CKD = chronic kidney disease; IADL = instrumental activities of daily living; SES = socio‐economic status. Source: (P. Painter & Roshanravan, 2013). Although the protective role of physical activity against other chronic diseases has been described extensively, little is known about its association with the development and progression of CKD. The findings of the available large‐scale cross‐sectional epidemiological studies suggest that a high physical activity level is positively associated with kidney function, i.e., higher values of GFR, and inversely associated with the prevalence of CKD both in the general population (Finkelstein, Joshi, & Hise, 2006; Hallan et al., 2006; Hawkins et al., 2011) and in diabetic patients (Kriska, LaPorte, Patrick, Kuller, & Orchard, 1991; Waden et al., 2008). However, given the cross‐sectional design of these studies, it is not possible to distinguish whether low physical activity predisposes the patient to the development of renal dysfunction, reflects the poor exercise capacity of individuals with established CKD, or both.
CHAPTER 20
Chronic Kidney Disease
INTRODUCTION
DEFINITION
ACR (mg/g or mg/mmol)
A1
A2
A3
normal to mildly increased
moderately increased
severely increased
<30 or <3
30–299 or 3–30
≥300 or ≥30
GRF (mL/min/1.73 m2)
G1
normal or high
>90
G2
mildly decreased
60–89
G3a
mildly to moderately decreased
45–59
G3b
moderately to severely decreased
30–44
G4
severely decreased
15–29
G5
kidney failure
<15
Absence of CKD. Low disease progression risk.
Mild CKD. Moderately increased disease progression risk.
Moderate CKD. High disease progression risk.
Advanced CKD. Very high disease progression risk.
PREVALENCE
ETIOLOGY
COMPLICATIONS
MANAGEMENT
NUTRITION IN CHRONIC KIDNEY DISEASE
Dietary constituent
Normal kidney function with increased CKD risk
Mild‐to‐moderate CKD
Advanced CKD
Ongoing dialysis or any stage with PEW
Energy (kcal/kg/d)
30–35; adjust to target weight reduction if BMI>30 kg/m2
30–35; increase proportion with LPD
30–35; increase proportion with LPD
30–35; target higher intake if PEW present or imminent
Protein (g/kg/d)
<1.0; increase proportion of plant‐based proteins
<1.0 (consider 0.6–0.8 if GFR<45 ml/min/1.73 m2 or rapid progression)
0.6–0.8, including 50% HBV protein, or <0.6 with addition of EAA or KA
1.2–1.4; may require >1.5 if hypercatabolic state develops
Sodium (g/d)
<4 (<3 in patients with hypertension)
<4; avoid intake of <1.5 if hyponatremia likely
<3; avoid intake of <1.5 if hyponatremia likely
<3
Potassium (g/d)
4.7 (same as recommended for general population)
4.7 unless frequent or severe hyperkalemia excursions likely
<3 if hyperkalemia occurs frequently during high‐fiber intake
<3; target high‐fiber intake
Phosphorus (mg/d)
<1000; minimize added inorganic phosphorus in preservatives and processed foods
<800; minimize added inorganic phosphorus and encourage consumption of more plant‐based foods
<800; minimize added inorganic phosphorus and encourage consumption of more plant‐based foods
<800; minimize added inorganic phosphorus; add phosphorus binder as needed
Calcium (mg/d)
1000–1300 (adjusted for age)
800–1000
800–1000
<800
Dietary fibers (g/d)
25–30; target higher proportion (>50%) of plant‐based foods
25–30 or more; higher proportion (>50%) of plant‐based foods
25–30 or more; consider >70% plant‐based foods
25–30 or more; suggest avoiding strict vegan diet
PHYSICAL ACTIVITY, FITNESS, AND CHRONIC KIDNEY DISEASE
PHYSICAL ACTIVITY AND FITNESS STATUS OF CHRONIC KIDNEY DISEASE PATIENTS
PHYSICAL ACTIVITY, FITNESS, AND RISK OF CHRONIC KIDNEY DISEASE
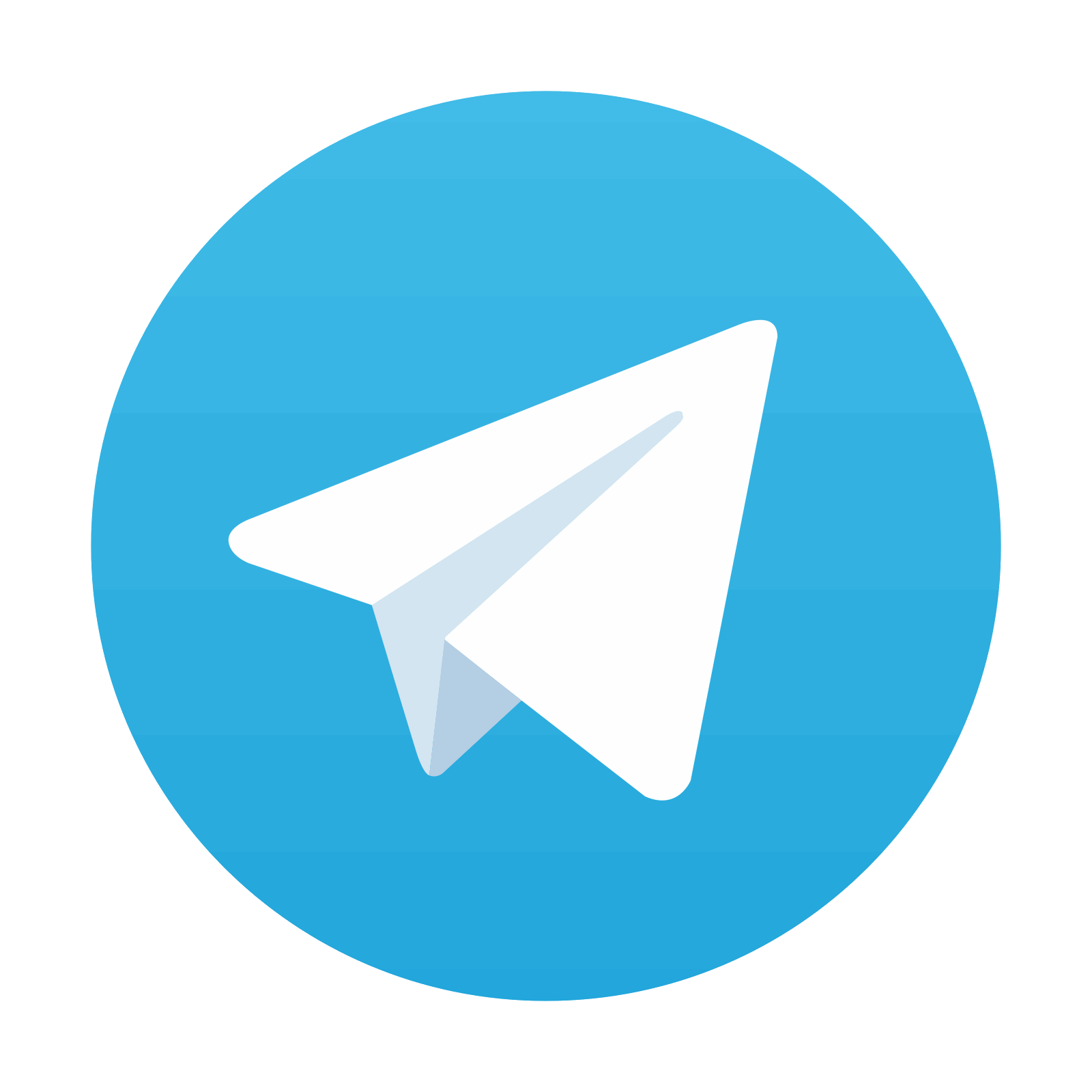
Stay updated, free articles. Join our Telegram channel

Full access? Get Clinical Tree
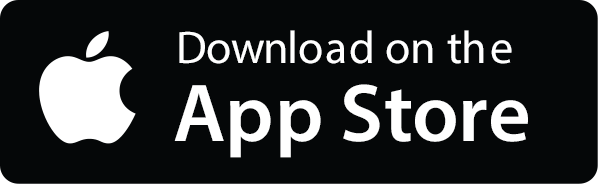
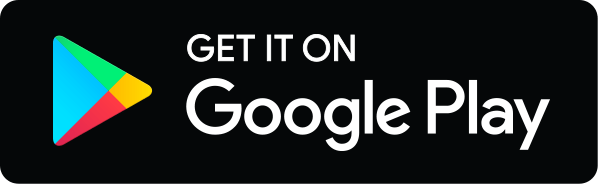