The main goal of a text on respiratory care equipment is to provide a source of technical information to supplement more general textbooks on respiratory care. One difficulty in creating such a reference is striking the proper balance between too much detail (as would be found in a ventilator operator’s manual) and too little (as would be found in a ventilator specification sheet). Both manuals and spec sheets are readily available for most ventilators. However, as you read these documents, you quickly realize an even bigger problem: there is no standardization in either vocabulary or format, making comparisons among devices difficult. This problem is particularly bothersome when it comes to understanding the modes of ventilation offered by the many different types of mechanical ventilators. An earlier version of this chapter (2016) listed almost 300 unique mode names on several dozen ventilators,13 but they were certainly not 300 unique modes. There are many cases of different names for identical modes (e.g., Pressure-Control Ventilation Plus Adaptive Pressure Ventilation on the Hamilton Galileo is the same as Pressure Regulated Volume Control on the Siemens Servo 300) and a few cases of the same name used for very different modes (e.g., Assist/Control on the Puritan Bennett 840 is a form of volume control whereas Assist/Control on the Bear Cub infant ventilator is a form of pressure control). The purpose of classifying modes of ventilation is the same as the purpose of classifying anything else—to make possible meaningful comparisons. By comparing and contrasting modes, we are better able to match a given patient’s immediate needs to the available technology. This is the same as matching a particular drug to a patient’s disease. What is different is that, until now, there has never been a standard reference for classifying modes of ventilation like there is for drugs. This chapter is, in part, an attempt to fill that need. Despite the lack of a standardized classification system for modes, such a taxonomy has been described previously.14,15 The basic principle of this system has been embraced by authors of ventilator textbooks.16–18 What has been lacking, until now, is a systematic approach for teaching this system. Indeed, a taxonomy can be viewed as the last step in a sequence of theoretical constructs that build the classification system. This section provides a detailed explanation of 10 fundamental constructs of ventilator design and function upon which a practical ventilator mode taxonomy can be based. These concepts build on one another to yield a practical framework for understanding, comparing, and contrasting the features of ventilators. This framework can provide the structural outline for a complete didactic course on mechanical ventilation. The ultimate purpose of these activities is to enable clinicians to select the most appropriate mode for a given clinical situation. The didactic approach described here is informed by the data from an international survey.19 After describing these 10 concepts (stated as maxims, or concise statements of scientific principles), we demonstrate how the resulting taxonomy can be used to guide selection of modes. The most basic function of a ventilator is to deliver a breath. The most basic definition of a breath is one cycle of inspiratory flow followed by a matching expiratory flow (Figure 10-9). These flows are paired by size, meaning approximately equal inspiratory and expiratory volumes. Inspiration is not necessarily followed immediately by the matching expiration. For example, during airway pressure release ventilation, the transition from low pressure to high pressure results in a large mandatory breath inspiration. This is possibly followed by a few small spontaneous breath inspirations and expirations. Finally, the transition from high to low pressure results in the matching mandatory exhalation. It is also possible to have many small mandatory breaths superimposed on larger spontaneous breaths, as during high-frequency oscillatory ventilation. FIGURE 10-9 A breath is defined as one cycle of inspiratory flow followed by a matching expiratory flow, yielding approximately the same volumes. Reproduced with permission from Mandu Press Ltd., Cleveland, OH. The two most basic definitions in reference to a breath are inspiratory time and expiratory time. Inspiratory time is the period from the start of inspiratory flow to the start of expiratory flow. Inspiratory time equals inspiratory flow time plus inspiratory hold time (pause time). Inspiratory hold time, or pause time, is the period from the cessation of inspiratory flow (into the airway opening) to the start of expiratory flow during mechanical ventilation. On some ventilators, inspiratory hold time is set directly. On others, hold time is the difference between the preset inspiratory time and the inspiratory flow time due to the preset tidal volume at the preset inspiratory flow (i.e., Flow time = Tidal volume/Inspiratory flow). Inspiratory hold time is often used to increase mean airway pressure and improve oxygenation and to create a static airway pressure (called plateau pressure) that may be used to calculate respiratory system resistance and compliance. Expiratory time is the period from the start of expiratory flow to the start of inspiratory flow. Figure 10-9 is the basis for several mathematical equations relevant to ventilator settings (Table 10-1). TABLE 10-1 Equations for Breath Timing Based on Figure 10-9 Parameter Symbol Volume (L) V Flow (L/min) Time (s) T Frequency (breaths/min) f Parameter Symbol Equation Inspiratory time (s) TI (60 / f ) – Te or (60 × I ) / [f × (I + E)] Expiratory time (s) TE (60 / f ) – TI or (60 × E) / [f × (I + E)] Total cycle time or period (s) Ttot TI + TE or 60/f Inspiratory to expiratory time ratio I:E TI : TE or D/(100% – D) Duty cycle (%) D (TI / Ttot ) × 100% or 100% × I / (I + E) Mean inspiratory flow Tidal volume VT Reproduced with permission from Mandu Press Ltd., Cleveland, OH. The main purpose of a ventilator is to assist with the patient’s work of breathing. Work is a function of pressure necessary to expand the volume of the respiratory system. Pressure is generated by either the patient’s inspiratory muscles (Pmus) or the ventilator (Pvent). Either way, there is an increase in the pressure difference across the respiratory system (which we will refer to simply as inspiratory pressure; a detailed discussion of the subject is provided in a recent textbook20) so that its volume increases during inspiration. An assisted breath can be recognized on a ventilator graphic display by examining the pressure waveform during inspiration (i.e., positive flow). If airway pressure rises above baseline (end-expiratory pressure) during inspiration (as defined in maxim 1 as a positive change in flow), then the breath is assisted. If airway pressure falls below baseline pressure during inspiration, the patient is doing some work on the ventilator, and we say the breath is “loaded,” rather than assisted. Some loading is unavoidable because ventilators cannot control airway pressure perfectly; some pressure drop is necessary for pressure triggering a breath, and there are electrical/mechanical delays between sensing a patient effort and the start of inspiratory flow.21 A ventilator provides assistance by maintaining a desired waveform for either inspiratory pressure (called pressure control (PC)) or inspiratory volume/flow (called volume control (VC)). The theoretical foundation for this assertion is a mathematical model of patient–ventilator interaction, known as the equation of motion for the respiratory system:22 where Pvent(t) is inspiratory pressure generated by the ventilator as a function of time (see maxim 2), E is the elastance of the respiratory system (lungs and chest wall), V(t) is volume as a function of time, R is respiratory-system resistance, and In this equation, the term EV(t) has the units of pressure and is called the elastic load. The term RV. (t) also has the units of pressure and is called the resistive load. Hence, the term breath unloading means that the ventilator supplies some portion of the work to deliver volume and flow against these loads. A plot of Pvent(t) versus time gives the airway pressure waveform seen on ventilator displays. If the shape of this waveform is predetermined by the ventilator settings, we say that the ventilator is providing pressure control. One very confusing issue with pressure control is that sometimes the operator sets the magnitude of the pressure waveform relative to atmospheric pressure (called peak inspiratory pressure) and other times the magnitude is set relative to PEEP (in this case, simply called inspiratory pressure).23 A plot of the V(t) or Volume control (VC) means that both volume and flow are preset before inspiration. Setting tidal volume is a necessary but not sufficient criterion for declaring volume control because some modes of pressure control allow the operator to set a target tidal volume but allow the ventilator to determine the flow (see adaptive targeting schemes later in this chapter). Similarly, setting flow is also a necessary but not sufficient criterion; some pressure control modes allow the operator to set the maximum inspiratory flow, but the tidal volume depends on the inspiratory pressure target and respiratory system mechanics. Pressure control (PC) means that inspiratory pressure as a function of time is predetermined. In practice, this currently means presetting a particular pressure waveform (e.g., P(t) = constant), or inspiratory pressure is set to be proportional to patient inspiratory effort, measured by various means. For example, P(t) = NAVA level × EAdi(t), where NAVA stands for neurally adjusted ventilatory assist and EAdi stands for electrical activity of the diaphragm (see servo targeting scheme later in this chapter). In a passive patient, after setting the form of the pressure function (i.e., the waveform), volume and flow depend on E and R.24 In rare cases, no parameters of the pressure, volume, or flow waveforms are preset Examples of this are high-frequency oscillatory ventilation (3100 ventilator) and volumetric diffusive respiration (Percussionaire). In the earlier version of this chapter (2016),13 we called this “time control,” which we have come to see is incorrect. There is, in fact, no control variable in the sense we have described because these devices use open-loop control, rather than closed-loop, or feedback, control (see the information on targeting schemes below). Instead, we will classify these particular high-frequency devices as pressure control with open loop (PCOL). The algorithm for determining the control variable for a given mode of ventilation is shown in Figure 10-10. Common pressure, volume, and flow waveforms produced by ventilators are shown in Figure 10-3. Figures 10-11 and 10-12 show the relationships among factors that determine minute ventilation for volume control and pressure control. FIGURE 10-10 Algorithm for determining the control variable of a mode. Paw = airway pressure, VT = tidal volume. Reproduced with permission from Mandu Press Ltd., Cleveland, OH.; Chatburn RL, Volsko TA, Hazy J, Harris LN, Sanders S. Determining the basis for a taxonomy of mechanical ventilation. Respir Care 2012;57(4):514-524. FIGURE 10-11 Influence diagram for volume control. Reproduced with permission from Mandu Press Ltd., Cleveland, OH. FIGURE 10-12 Influence diagram for pressure control. Reproduced with permission from Mandu Press Ltd., Cleveland, OH. For unassisted breathing, the brain generates signals to start and stop inspiration. When a patient is connected to a ventilator, the ventilator requires a signal to start and stop the inspiratory flow. Starting inspiration is called triggering. Common trigger signals include time, airway pressure, volume, flow, and the electrical signal from the diaphragm, but other signals are possible. Stopping inspiration is called cycling. Common cycle signals are the same as for triggering. The term sensitivity refers to the amount that the trigger or cycle signal must change before inspiration starts or stops. As it turns out, trigger and cycle are key definitions in the development of a classification system for modes of ventilation. Some authors (including those who write standards and ventilator operator’s manuals) tend to restrict the definition of trigger to only the act of the patient initiating inspiration, leaving machine starting of flow undefined. As a result, they have no convenient method of distinguishing machine trigger and cycle events from patient trigger and cycle events (see maxim 5) and no practical way to define mandatory and spontaneous breaths (see maxim 6). Without definitions for mandatory and spontaneous breaths, we cannot define ventilatory patterns (maxim 7), and there is no basis for the taxonomy built on all of the maxims (see maxim 10). The point is that each of the maxims is an important component of the theoretical foundation for this system of classifying modes. There may, of course, be other ways of developing a mode taxonomy; however, none has yet been described that is as practical and consistent with current clinical and educational paradigms as the one described here. We normally want to keep the delivery of inspiratory flow in synchrony with the patient, assuming the patient is capable of generating trigger and cycle signals. Of course, if the patient is heavily sedated or paralyzed, we must rely on the ventilator to trigger and cycle on its own. In general, we don’t usually specify whether the patient or the machine may trigger or cycle by using particular settings on the ventilator. Rather, these capabilities are built into the mode of ventilation (a mode will be defined in maxim 10). For now, we need to understand how we determine whether the patient or the machine has triggered or cycled an inspiration (or has the potential to do so). For this, we again invoke the equation of motion described previously. Time (t) in the equation is a variable independent of the patient. Suppose inspiration starts after a preset time interval (e.g., because of a preset breathing frequency) or ends after a preset inspiratory time or a preset tidal volume (the integral of flow with respect to time). Clearly, inspiration has started or stopped regardless of any inspiratory or expiratory efforts made by the patient (i.e., changes in Pmus). Thus, we say that inspiration is machine triggered or cycled. Triggering due to a preset frequency is time triggering. Another signal the ventilator can use to trigger inspiration is if the minute ventilation (tidal volume divided by the time for one breath cycle) drops below a preset threshold. Cycling due to a preset tidal volume is called volume cycling; cycling due to a preset inspiratory time (or inspiratory pause time) is time cycling. Conversely, patient triggering, or patient cycling, implies that inspiration starts or stops independent of any preset trigger or cycle signals generated by the ventilator. In the equation of motion, Pmus, elastance, and resistance are all patient determined. Thus, if inspiration starts or stops because of one or more of them, we say that inspiration is patient triggered or cycled. For example, it is easy to understand that, if the patient makes an inspiratory effort (positive change in Pmus), then the ventilator may detect this by a change in airway pressure (pressure triggering), volume (volume triggering), flow (flow triggering), or perhaps even electrical signals derived from the movement of the diaphragm (e.g., neurally adjusted ventilatory assist) or expansion of the chest wall (e.g., electrical impedance tomography). On detecting the signal, inspiration is triggered on. Similarly, if the patient makes an expiratory effort (negative change in Pmus), then inspiration may be cycled off. Thus, the patient can actively pressure, volume, or flow trigger and cycle inspiration. The distinction between patient and machine triggering
Section 2: Understanding Ventilator Technology
1. A Breath Is Defined in Terms of the Flow-Time Curve
2. A Breath Is Assisted If the Ventilator Provides Some or All of the Work of Breathing
3. A Ventilator Provides Assistance by Controlling Either the Pressure or Volume Waveform Based on the Equation of Motion for the Respiratory System
(t) is flow as a function of time. Note that all these variables are measured relative to their end-expiratory values. Under static (i.e., no flow) conditions, these variables are P vent = end-expiratory pressure, usually set PEEP; V = end-expiratory lung volume (functional residual capacity if PEEP = 0); and
= 0, assuming no auto-PEEP.
(t) yields the volume and flow waveforms. If the shape of these waveforms is predetermined by the ventilator settings, we say the ventilator is providing volume control. Note that direct control of the flow waveform (e.g., with a flow control valve) implies indirect control of volume because volume is the integral of flow with respect to time. It is also true that direct control of the volume waveform (e.g., with a piston) implies indirect control of flow because flow is the derivative of volume with respect to time. We say “volume control” rather than “flow control” simply for historical reasons. We don’t use both because that would lead to unnecessary complications when it comes to classifying modes.
4. Breaths Are Classified According to the Criteria That Trigger (Start) and Cycle (Stop) Inspiration
5. Trigger or Cycle Events Can Be Either Patient or Ventilator Initiated
Stay updated, free articles. Join our Telegram channel

Full access? Get Clinical Tree
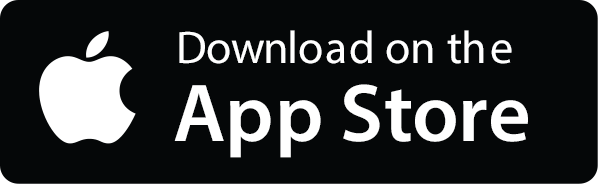
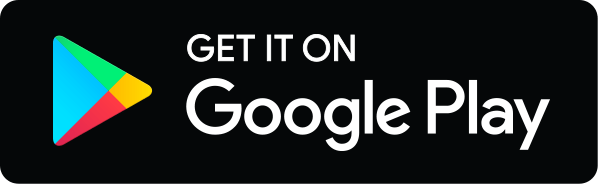