Physiology
Cardiovascular Examination
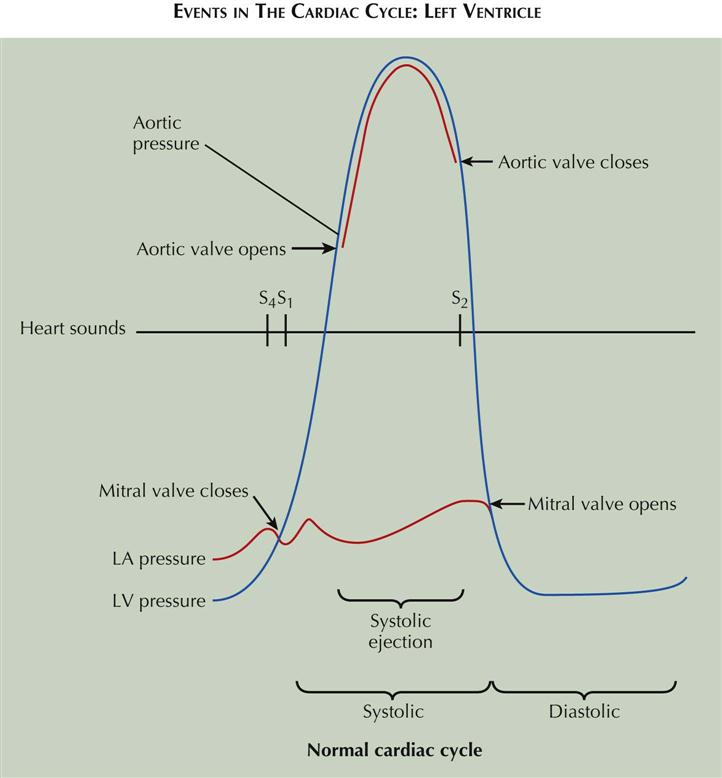
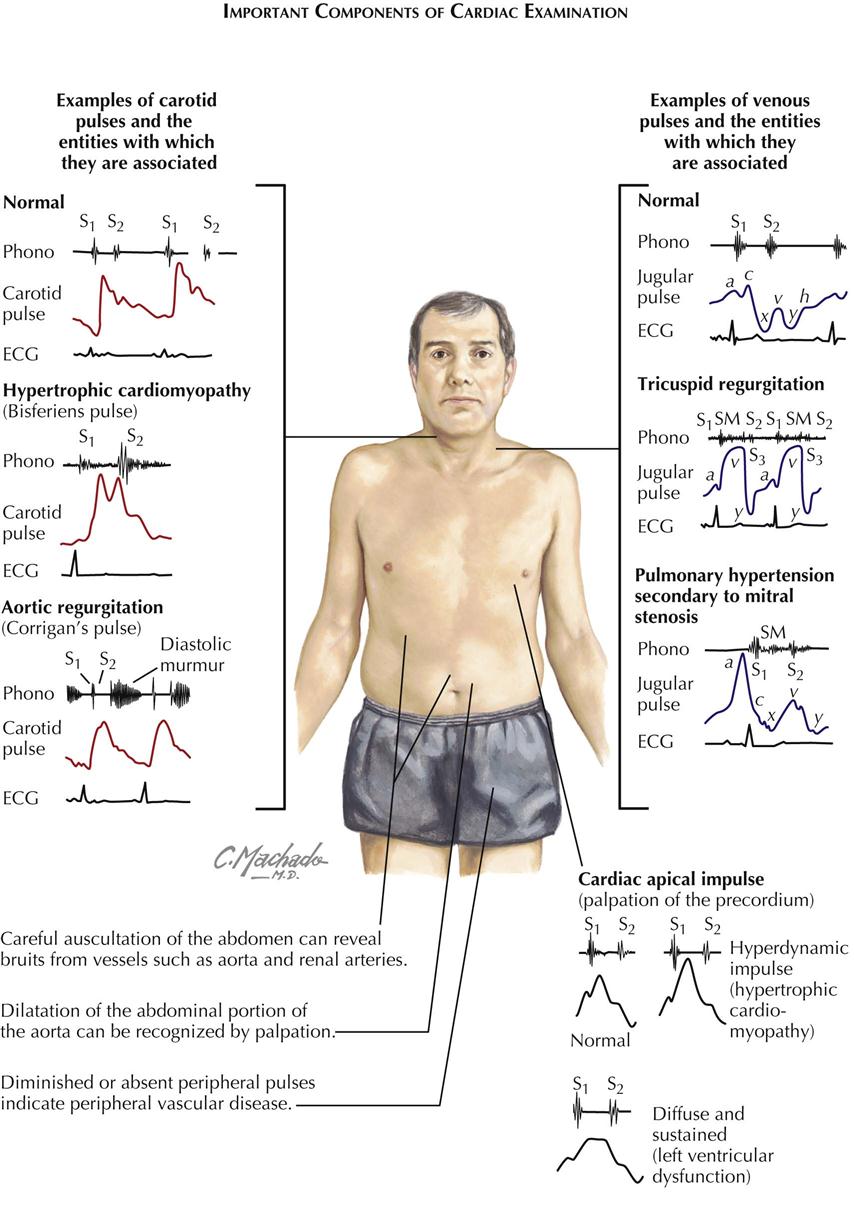
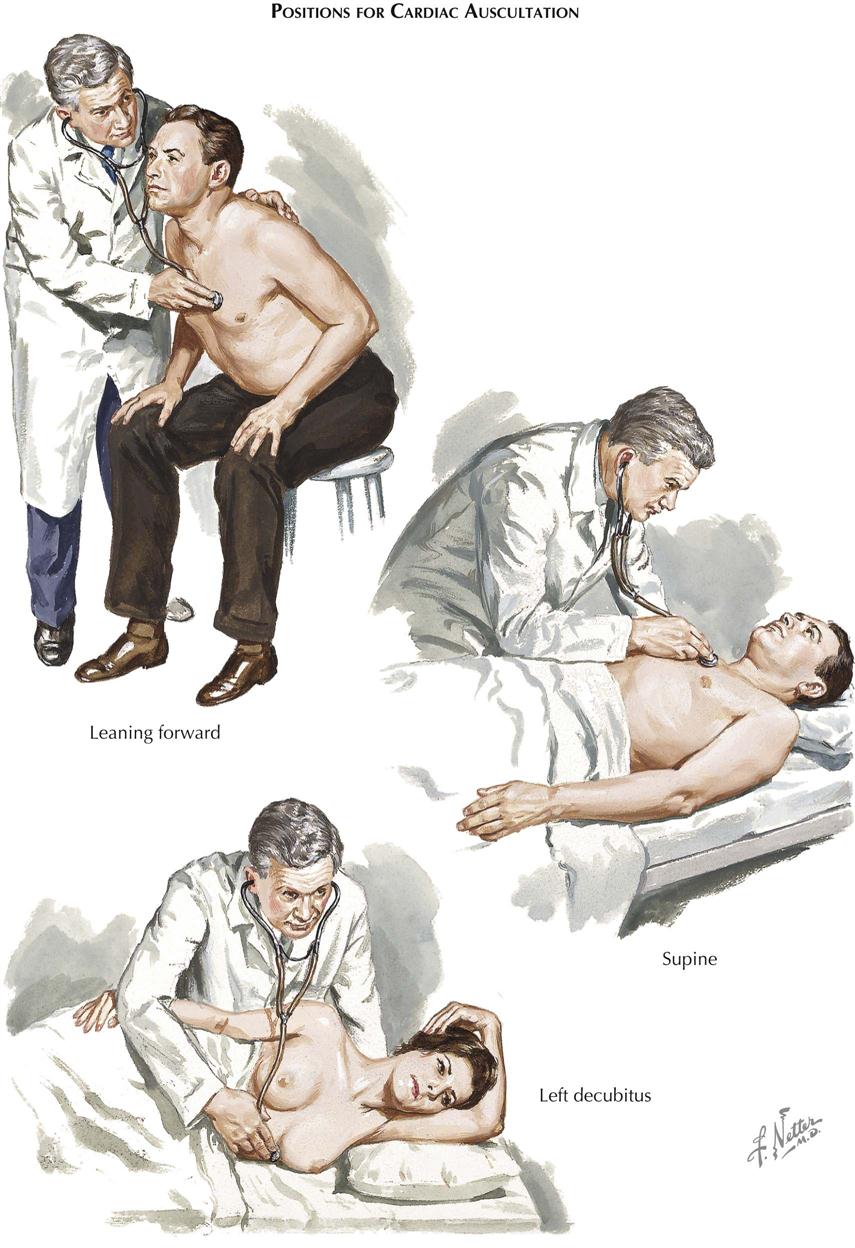
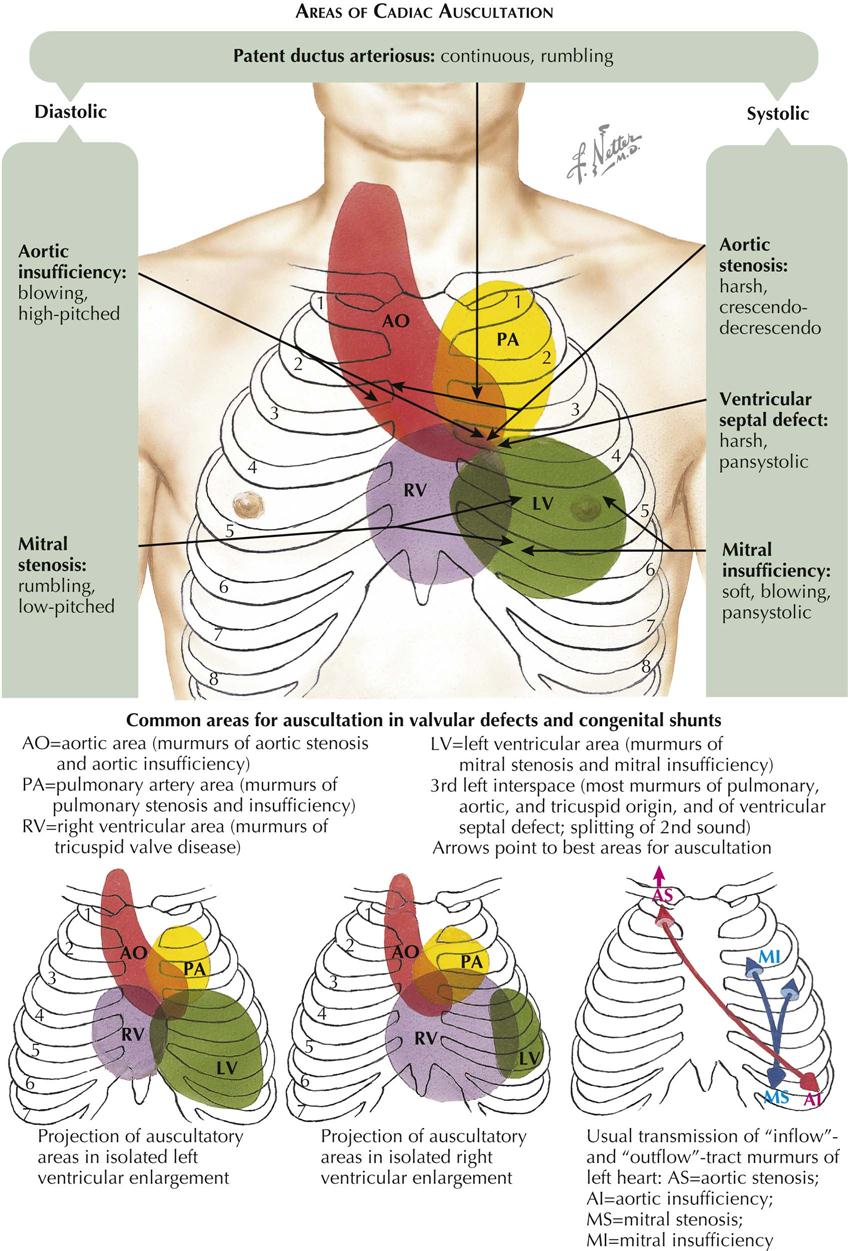
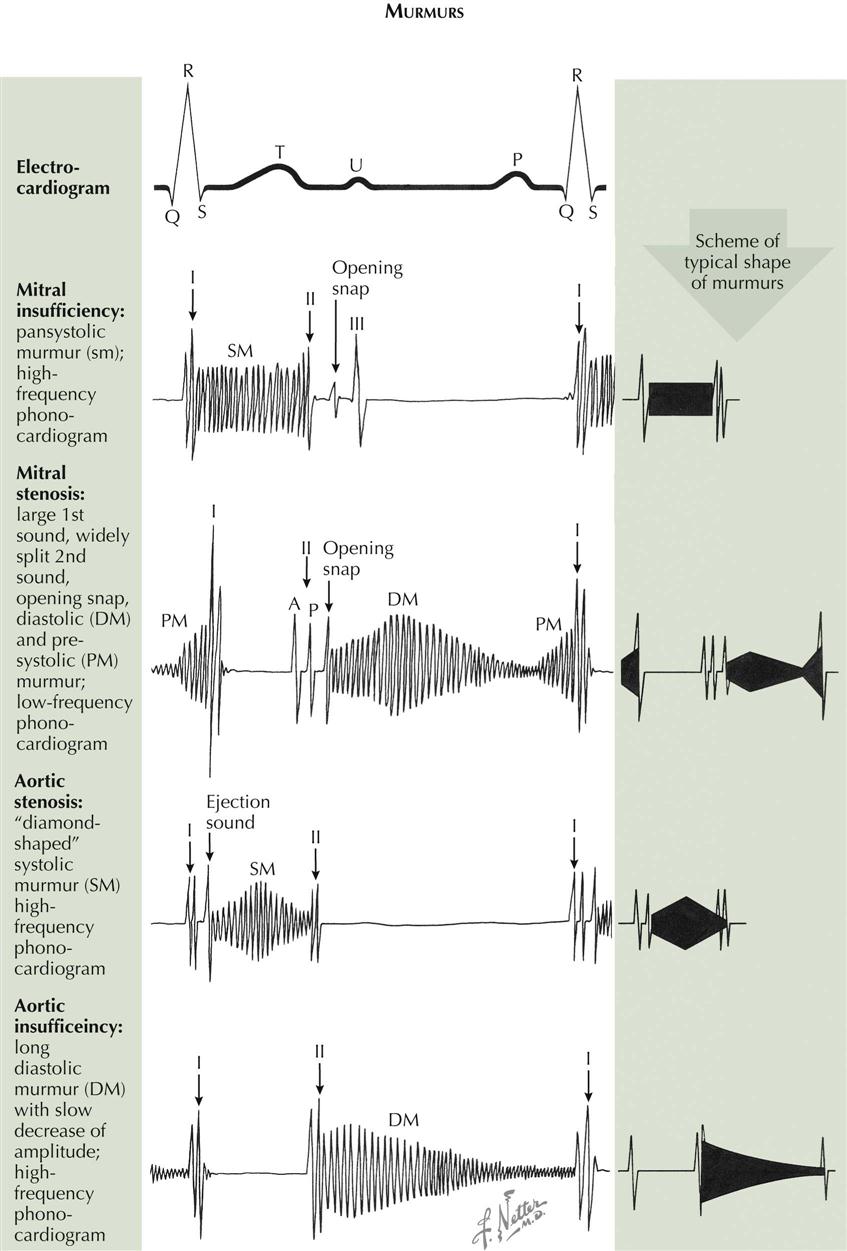
Events in the Cardiac Cycle: Left Ventricle
The events occurring during the cardiac cycle are driven by the left ventricular (LV) pressure. The mitral valve closes and results in the first heart sound (S1) when LV pressure rises above left atrial (LA) pressure (see Plate 2-1). The aortic valve opens when LV pressure rises above aortic diastolic pressure and is the onset of systolic ejection. The aortic valve closes and produces the second heart sound (S2) when LV pressure falls below aortic pressure and terminates systolic ejection. The mitral valve opens when LV pressure falls below LA pressure. The fourth heart sound (S4) occurs in late diastole following atrial contractions.
Cardiac auscultation is not the only way to examine the cardiovascular system. Peripheral vessels reflect what is occurring in the cardiovascular system; for example, carotid and femoral artery examination can reveal peripheral vascular disease. Bruits over these vessels may indicate carotid stenosis or aortofemoral stenosis. An abdominal bruit may indicate renal artery stenosis. A decrease in blood pressure in either arm suggests subclavian artery stenosis. If the ankle-brachial index (ratio of systolic pressure in arm to systolic pressure in ankle using Doppler technique) is less than 0.9, some peripheral artery disease may be present.
Jugular venous pulsations seen when the patient is lying with the upper body elevated to 30 degrees suggests elevated RA pressure of several causes.
Precordial palpation before auscultation of the heart can help the examiner make the proper cardiac diagnosis. The apical impulse can suggest LV hypertrophy (enlargement) if sustained and slow rising. Palpation along the left sternal border may reveal a parasternal lift, which suggests an elevated right ventricular (RV) pressure of about 40 mm Hg. Plate 2-2 illustrates some examples of carotid pulses and venous pulsations and cardiac apical impulses associated with specific cardiac disease states.
The basic instrument of auscultation is the human ear. Although the stethoscope has technical advantages, it often distorts, decreases, or selectively emphasizes certain vibrations. Frequently, the naked ear is superior to the stethoscope in the detection of low-pitched vibrations (S3, S4) because the ear is a larger collector of sound and fuses auditory with palpatory perception.
Positions for Cardiac Auscultation
In auscultation, various patient positions can be used (see Plate 2-3). The patient may be sitting, supine, lying on the left side, or bent forward to increase the contact of the apex with the chest wall, as preferred for mitral or LV sounds and murmurs. The left side (left decubitus) position accentuates the rumbling murmur of mitral stenosis. The bent-forward position is preferred for aortic diastolic murmurs, whereas the supine position is best for pulmonic and tricuspid murmurs.
Areas of Cardiac Auscultation
The conventional designations for areas of auscultation have been mitral, tricuspid, aortic, and pulmonary. Current understanding divides the thorax into seven areas: left ventricular, right ventricular, left atrial, right atrial, aortic, pulmonary, and descending thoracic aortic (see Plate 2-4).
Left Ventricular Area
The apical area (“mitral” area) is the best location for detecting not only the murmur of mitral stenosis or insufficiency but also the LV or atrial gallops and the aortic component of the second sound (A component of II). The murmurs of aortic stenosis and especially of aortic insufficiency also are often heard well at this location. However, these vibrations are detected over a larger area formed by the entire left ventricle, centering around the apical beat and extending to the fourth and fifth left interspaces medially and to the anterior axillary line laterally. In patients with ventricular enlargement, the sound shifts to either the left or the right.
Right Ventricular Area
The “tricuspid” area is renamed the right ventricular area. In addition to the murmurs of tricuspid stenosis and insufficiency, RV and atrial gallops and the murmurs of pulmonary insufficiency and ventricular septal defect can be well heard here. The RV area includes the lower part of the sternum and the fourth and fifth interspaces, 2 to 4 cm to the left and 2 cm to the right of the sternum. This area may extend also to the point of maximal impulse, in the presence of severe RV enlargement; the “apex” in such patients is formed by the right ventricle.
Aortic Area
The aortic component of S2 and the murmurs of aortic valve defects are often heard well at the third left interspace (Erb’s area). This point is frequently more revealing than the second right interspace, except in patients with dilatation of the ascending aorta, where the manubrium or the second right interspace may be more informative. The aortic area should designate both the aortic root and part of the ascending aorta. The vibrations heard best in this area include the murmurs caused by aortic stenosis, aortic insufficiency, augmented flow across the aorta or dilatation of the ascending aorta, and abnormalities of the neck arteries, as well as the aortic ejection click and aortic component of S2.
Pulmonary Area
The pulmonary area should refer to the pulmonary artery rather than the pulmonary (pulmonic) valve. The murmurs of pulmonary stenosis and insufficiency, the murmur caused by increased flow or dilatation of the pulmonary artery, the pulmonary ejection click, the pulmonary component of the second sound (P component of II), and the murmur of patent ductus arteriosus are heard best here. The pulmonary area is formed by the second left interspace near the sternal edge and extends upward to the clavicle and downward to the third left interspace near the sternal margin. However, it may also extend posteriorly at the level of the fourth and fifth dorsal vertebrae.
Most Significant Auscultatory Findings
Heart Sounds
The first heart sound (S1) is often louder over the LV area (apex and midprecordium), whereas the second heart sound (S2) is frequently louder over the aortic and pulmonary areas (base). The first sound is a long noise of lower tonality, whereas the second sound is shorter and sharper.
In normal adolescents or young people, S1 may be split. The best area for hearing this split sound is at the third left interspace. This splitting is not influenced by respiration. The loudness of S1 is decreased in myocarditis, myocardial infarction (MI), myocardial fibrosis, hypothyroidism, mitral insufficiency (soft), aortic insufficiency, and pericarditis with effusion. S1 is increased in mitral stenosis, systemic hypertension, and hyperthyroidism.
The second sound is frequently split during inspiration and in normal children and young people. The best area for hearing this splitting is the third left interspace, close to the sternum (Erb’s point). S2 has an increased loudness of the aortic component in systemic hypertension, coarctation of the aorta, and aortitis. Decreased loudness of S2 characterizes aortic stenosis. The aortic component may be so delayed as to follow the pulmonary component, a paradoxical splitting. S2 has increased loudness of the pulmonary component in pulmonary hypertension, whereas loudness is decreased in pulmonary stenosis. The pulmonary component not only is smaller but also is delayed, causing a wider splitting. S2 has wider, fixed splitting in conditions presenting a diastolic overload of the right side of the heart and in right bundle branch block, because of a delay in the pulmonary component. Patients with left bundle branch block may present with such a delay in the aortic component as to cause paradoxical splitting.
The third heart sound (S3) may be normal in children, adolescents, and young athletes but may be audible over the LV or RV area in ventricular overload, myocarditis, tachycardia, or heart failure.
The fourth heart sound (S4) is not heard in the normal heart. S4 is audible over the LV area in hypertension, during myocardial ischemia, or when the ventricle is stiff and noncompliant, as in diastolic dysfunction. S4 can also be heard in patients with ventricular volume overload, myocarditis, tachycardia, atrial flutter, and complete or incomplete atrioventricular (A-V) block or obstruction. The fourth sound is then called atrial gallop. A left atrial gallop is frequently heard in aortic stenosis or systemic hypertension. A right atrial gallop is often heard in pulmonary stenosis or pulmonary hypertension. A slightly different type is the summation gallop, caused by the summation of S3 and S4. This is most often seen in patients with tachycardia and grade 1 A-V block.
A systolic ejection click can be heard over either the pulmonary area (pulmonary ejection sound) or the aortic area (aortic ejection sound). These clicks are caused by “doming” of the aortic or pulmonary valve; the aortic valve may be bicuspid. These ejection sounds occur with dilatation of the aorta or pulmonary artery or narrowing of the aortic or pulmonary valve, usually with poststenotic dilatation. The ejection click is a high-frequency sound due to abrupt halting of the valve opening.
A diastolic opening snap can be heard in the fourth left interspace, close to the sternum, over the entire LV area, or even over the entire precordium, in patients with a pliable noncalcified valve. This is the mitral opening snap most often heard in mitral stenosis. Occasionally it can be heard in diastolic overload of the left side of the heart (mitral insufficiency, patent ductus). A tricuspid opening snap is audible over the RV area in patients with tricuspid stenosis and occasionally can be heard in diastolic overload of the right ventricle (tricuspid insufficiency, atrial septal defect).
Murmurs
The regurgitant murmur of A-V valve insufficiency is holosystolic and usually loud (see Plate 2-5). The murmur of mitral insufficiency is maximal over the LV area and easily audible at the left axilla, whereas the murmur of tricuspid insufficiency is maximal over the RV area and is well heard over the right precordium. Inspiration or inspiratory apnea increases the loudness of the tricuspid murmur but decreases the loudness of the mitral murmur.
The murmur of A-V valve stenosis is a typical, low-pitched rumble which acquires higher pitch and greater loudness in presystole (presystolic accentuation) if there is sinus rhythm. It is heard best in mitral stenosis in the fourth left intercostal space, halfway between the apex and the sternal border. In tricuspid stenosis this rumble is heard best over the RV area. This murmur becomes louder in inspiration because of increased flow across the tricuspid valve during inspiration.
The regurgitant murmur of semilunar valve insufficiency is a high-pitched, blowing, occasionally musical decrescendo. In aortic insufficiency the murmur is loudest in the third left interspace (Erb’s area), and can be followed along the left sternal border toward the apex. If the ascending aorta is dilated, the murmur is louder in the second right interspace and can be followed downward along the right sternal border. In pulmonary insufficiency the murmur is loudest over the second left interspace and can be followed downward from the upper left to the lower right part of the sternum. If the patient has pulmonary hypertension, the murmur sounds similar to aortic regurgitation. If the pulmonary artery pressure is normal or low, the murmur may have a rumbling character.
The stenotic murmur of the semilunar valves is the loudest of all murmurs. It is harsh, starts slightly after S1 with aortic valve opening, and is often preceded by an ejection click, especially if the valve is bicuspid and mobile. The murmur often has a crescendo-decrescendo quality and ends before or with S2, depending on the severity of the stenosis. In aortic stenosis the murmur is maximal in the third left or second right interspace. It is readily heard over the suprasternal area and the carotid arteries and can be heard at the apex. In subvalvular aortic stenosis, it is maximal over the LV area. In pulmonary stenosis the murmur is best heard over the pulmonary area. It radiates moderately downward and often can be heard in the back over the lungs.
The murmur caused by a ventricular septal defect is long, harsh, and pansystolic. It is heard best over the RV area.
The murmur caused by patency of the ductus arteriosus is a continuous, machinery-like murmur because aortic systolic and diastolic pressures are higher than pulmonary systolic and diastolic pressures. It is best heard over the first and second intercostal spaces.
Friction Rubs
Friction rubs can be heard over various areas and resemble the sound made by rubbing new leather. Friction rubs can be heard in atrial systole, ventricular systole, and ventricular diastole (i.e., three-component rub).
Neural and Humoral Regulation of Cardiac Function
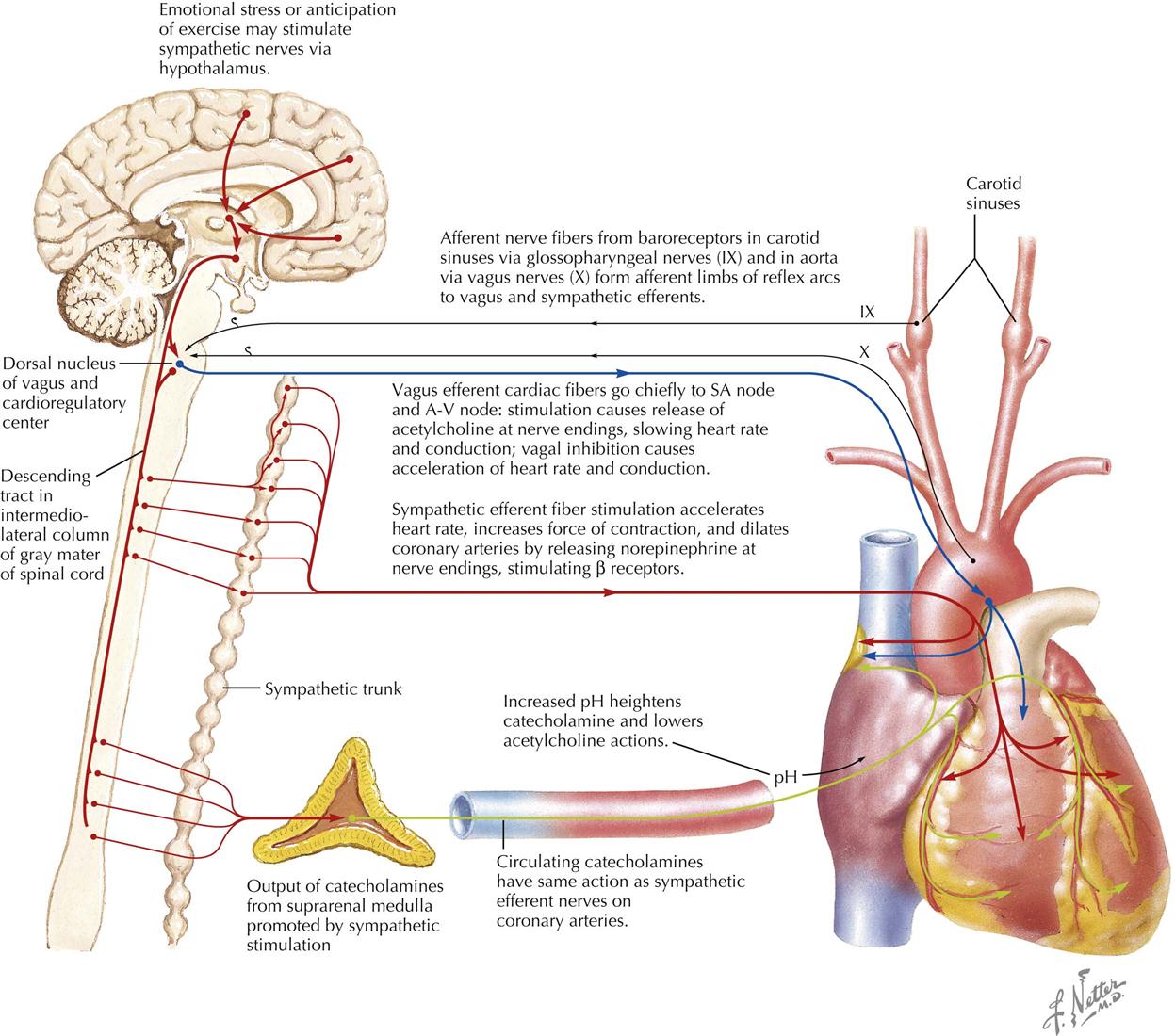
The efferent innervation of the heart is controlled by both the sympathetic nervous system and the parasympathetic nervous system. Afferent fibers accompany the efferents of both systems. The sympathetic fibers have positive chronotropic (rate-increasing) effects and positive inotropic (force-increasing) effects. The parasympathetic fibers have a negative chronotropic effect and may be somewhat negatively inotropic (but small and masked) in the intact circulatory system by the increased filling that occurs when diastolic filling time is increased.
The heart is normally under the restraint of vagal inhibition, and thus bilateral vagotomy increases the heart rate. Vagal stimulation not only slows the heart but also slows conduction across the A-V node. Sectioning of the cardiac sympathetics does not lower heart rate under normal circumstances.
The totally denervated heart loses some (but surprisingly little) of its capacity to respond to changes in its load. The denervated heart still responds to humoral influences, more slowly and less fully, but it is remarkable how well the secondary mechanisms, such as the suprarenal medullary output of catecholamines, can substitute for the primary mechanism that controls heart rate in exercise.
The nervous mechanisms controlling heart rate include the baroreceptor reflexes, with afferent arms from the carotid sinus, the arch of the aorta, and other pressoreceptor zones operating as negative feedback mechanisms to regulate pressure in the arteries. These reflexes affect not only heart activity but also the caliber of the resistance vessels in the vascular system.
The heart is also affected reflexively by afferent impulses via the autonomic nervous system. The response may be tachycardia or bradycardia, depending on whether the sympathetic or parasympathetic system is activated more strongly in the individual patient. Tachycardia is the common response in excitement.
Physiologic Changes During Pregnancy
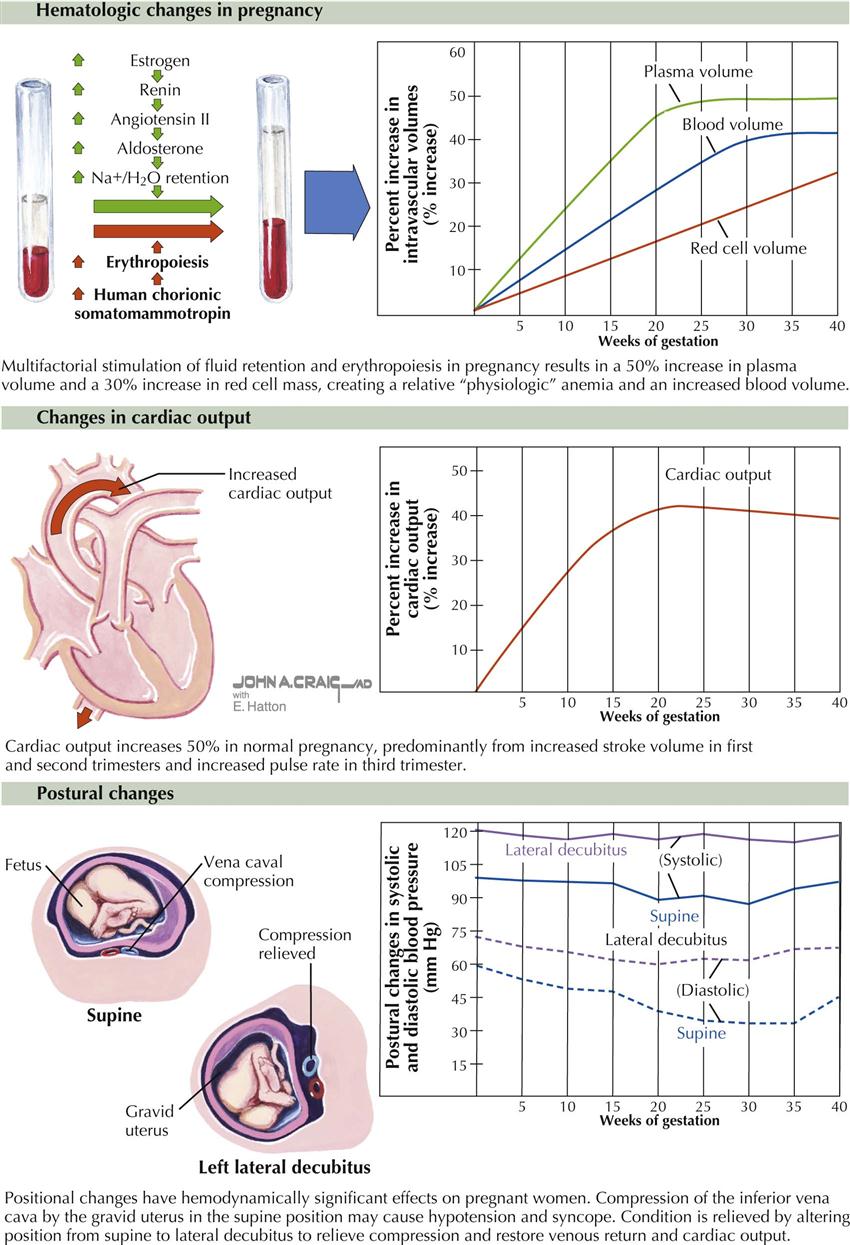
During a normal pregnancy, a woman undergoes multiple cardiovascular physiologic changes to support the increased metabolic demands of the woman and the unborn child. The changes are fairly typical in a healthy pregnant woman but may vary in magnitude from patient to patient. Early in the pregnancy, the first trimester, the changes are usually mild or may not be evident. The classic changes include the following;
Anatomic factors related to the enlarged uterus can also influence physiologic changes, particularly in the third trimester. Inferior vena cava compression can increase femoral venous pressure and can elevate the diaphragm, which in turn can shift the axis of the heart and alter the axis of the electrocardiogram toward the left.
Cardiac Catheterization
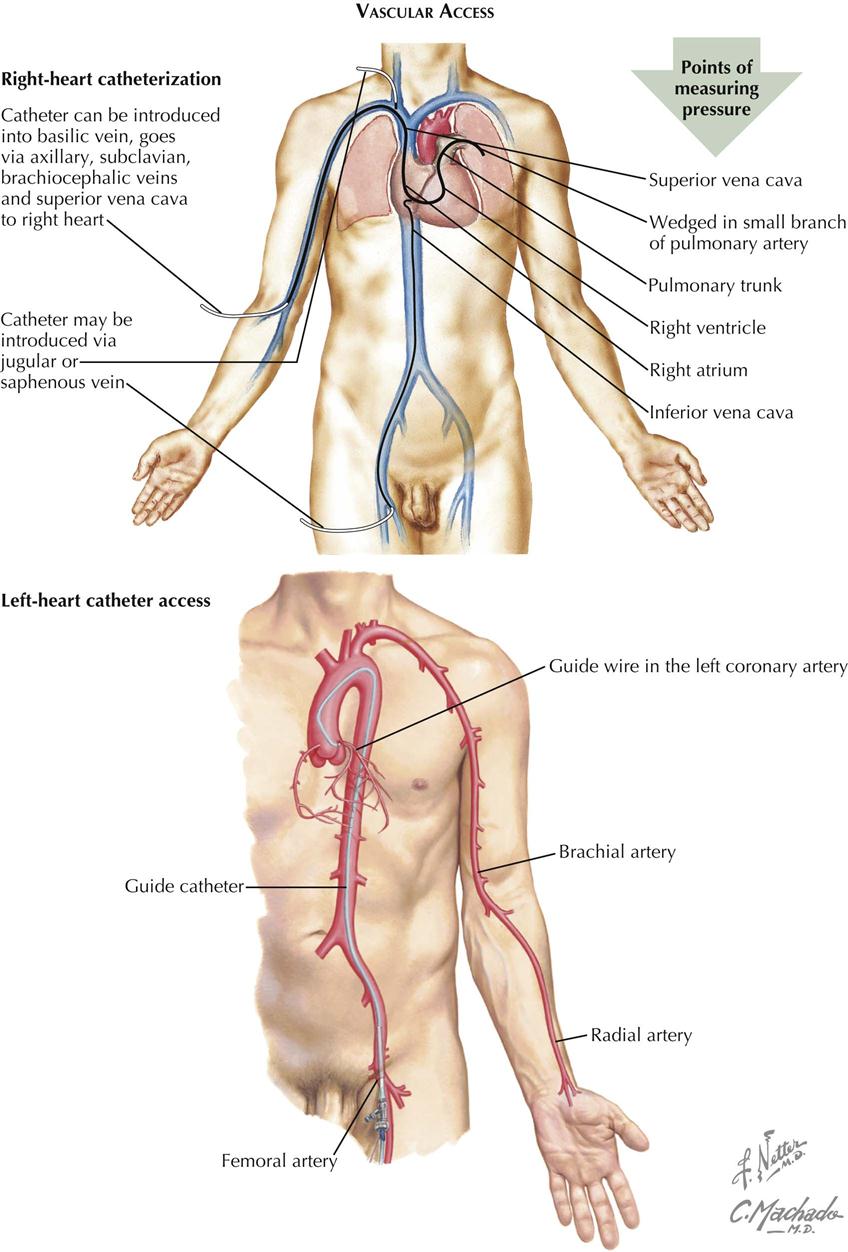
Vascular Access and Right-Sided Heart Catheterization
Cardiac catheterization, first attempted by Forssmann on himself in 1928, was developed by Cournand, Richards, and their colleagues and is now a common procedure in both clinical and research laboratories.
Technique
The primary goal of right-sided heart catheterization is to access the conditions existing in the chambers and great vessels of the right side of the heart. In these procedures a radiopaque flexible catheter of various designs, including balloon tipped, is introduced into a vein, usually the femoral or jugular, percutaneously using local anesthesia. After introduction into the vein, the catheter is manipulated under fluoroscopic control and constant electrocardiographic monitoring downstream through the venous system to the right atrium, and eventually into the right ventricle and pulmonary artery. The catheter is often wedged and is advanced into the most peripheral branch of the pulmonary artery that will accept the catheter tip or occlusion by a balloon-tipped catheter.. A pressure recorded from the wedge position has essentially the same mean pressure as the left atrium and the same but delayed phasic features. If there is no mitral stenosis, the pulmonary capillary wedge pressure (PCWP) reflects the left ventricular end-diastolic pressure (LVEDP).
Diagnostic Procedures
The position of the catheter in the fluoroscopic image may indicate some departure from the intracardiac course normally taken by a catheter. Examples include passage into a persistent left superior vena cava through the coronary sinus from the right atrium, passage through a patent ductus arteriosus, and traversal of an interatrial or interventricular septal defect.
Blood can be sampled for oxygen or other analysis, and pressures can be measured through the catheter from any point reached. Oxygen samples can be used to determine the site of entry into the right side of the heart and the size of a left-to-right intracardiac shunt at atrial, ventricular, or pulmonary artery levels in patients with congenital heart disease. Oxygen values from the pulmonary artery are used with other data to calculate the pulmonary blood flow with thermodilution and balloon-tipped catheters. Measurement of pressures through the catheter using external pressure transducers allows determination of the phasic form of the pressure in any location. Pressures recorded as a catheter traverses a valve permit an evaluation of the site and degree of valvular stenosis.
Special sensors at the tip of a catheter have been designed for the detection and recording of intracardiac ECGs and pressures.
Complications
Brief arrhythmias, vasovagal episodes, and minor phlebitis may be observed in patients undergoing catheterization. More serious complications are rare.
Left-Sided Heart Catheterization
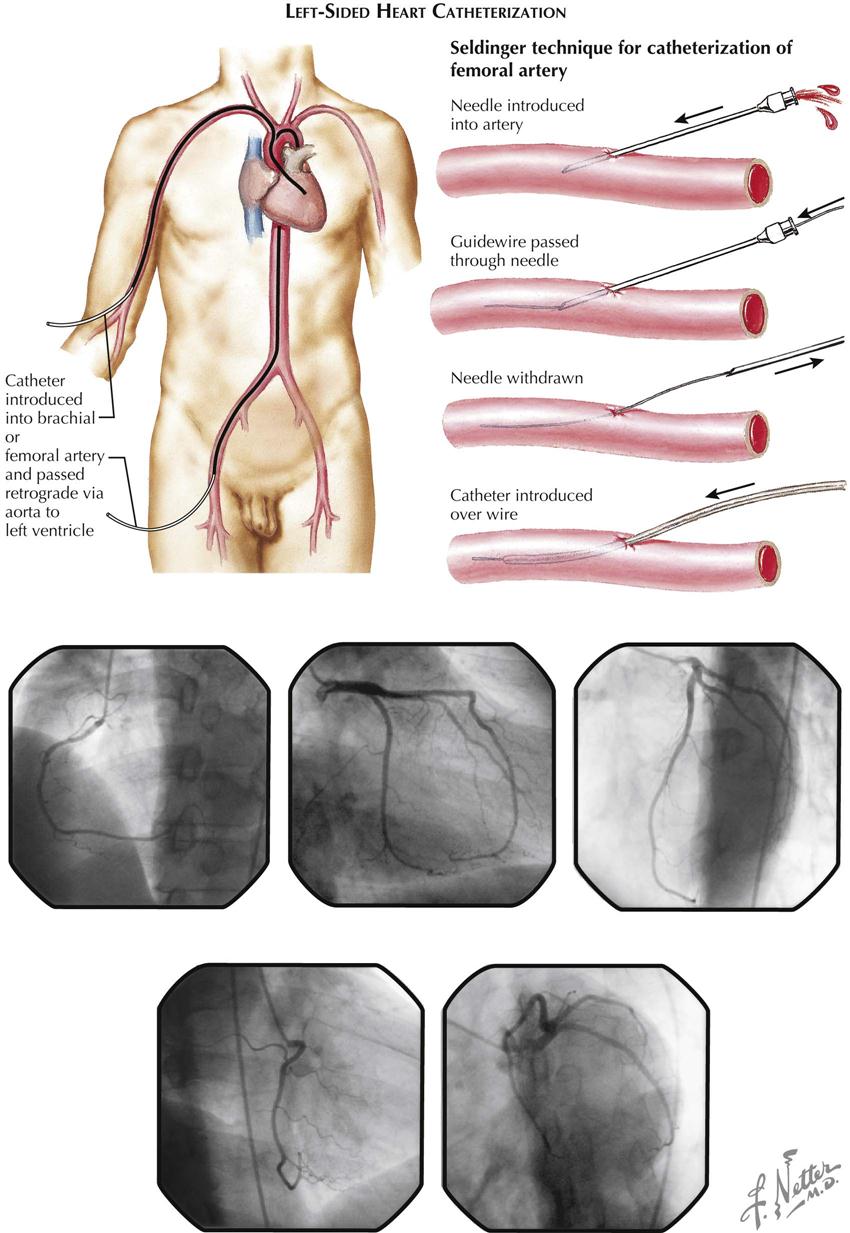
Technique
The aim of left-sided heart catheterization is the study of conditions in the chambers and vessels of the left side of the heart. In congenital heart disease the catheter may reach the left side of the heart from a right-sided heart chamber, passing through an atrial septal defect or a patent ductus arteriosus.
More often the left side of the heart is approached by retrograde passage of the catheter from its point of insertion into a peripheral artery, most commonly by percutaneous technic. This technique was designed by Seldinger (see Plate 2-9). The catheter is manipulated under fluoroscopic control in a retrograde direction using the Seldinger technique through the artery to the aorta and frequently across the aortic valve into the left ventricle. Entry into the left atrium retrograde through the mitral valve is possible but not typically used. Approach to the left atrium can also be accomplished using a transseptal technique by passage of the transseptal catheter and transseptal needle from a right femoral vein to the right atrium and across the atrial septum at the level of the fossa ovalis. The catheter can then be advanced into the left ventricle. Direct percutaneous needle puncture of the LV apex may be done to reach the left ventricle in special circumstances, such as LV pressure measurement in patients with mechanical aortic and mitral valves.
Diagnostic Procedures
Sampling and pressure measurements for left-sided heart catheterization do not differ from right-sided procedures. Valvular abnormalities can be estimated using simultaneous pressure measurements on both sides of the valve.
Complications
Arrhythmias, the most common complication of left-sided catheterization, usually respond to simple catheter withdrawal, although rarely may require therapy. Other complications include arterial spasm and the rare dissection or occlusion of the artery. Perforations of the walls of an artery or the aorta, a heart chamber, or a coronary artery also can occur rarely. Fluid should never be forced through a catheter from which blood cannot be withdrawn, particularly if the catheter is in the ascending aorta; a clot can be expressed and embolize peripherally to the brain.
Cardiac Output: Thermodilution Technique
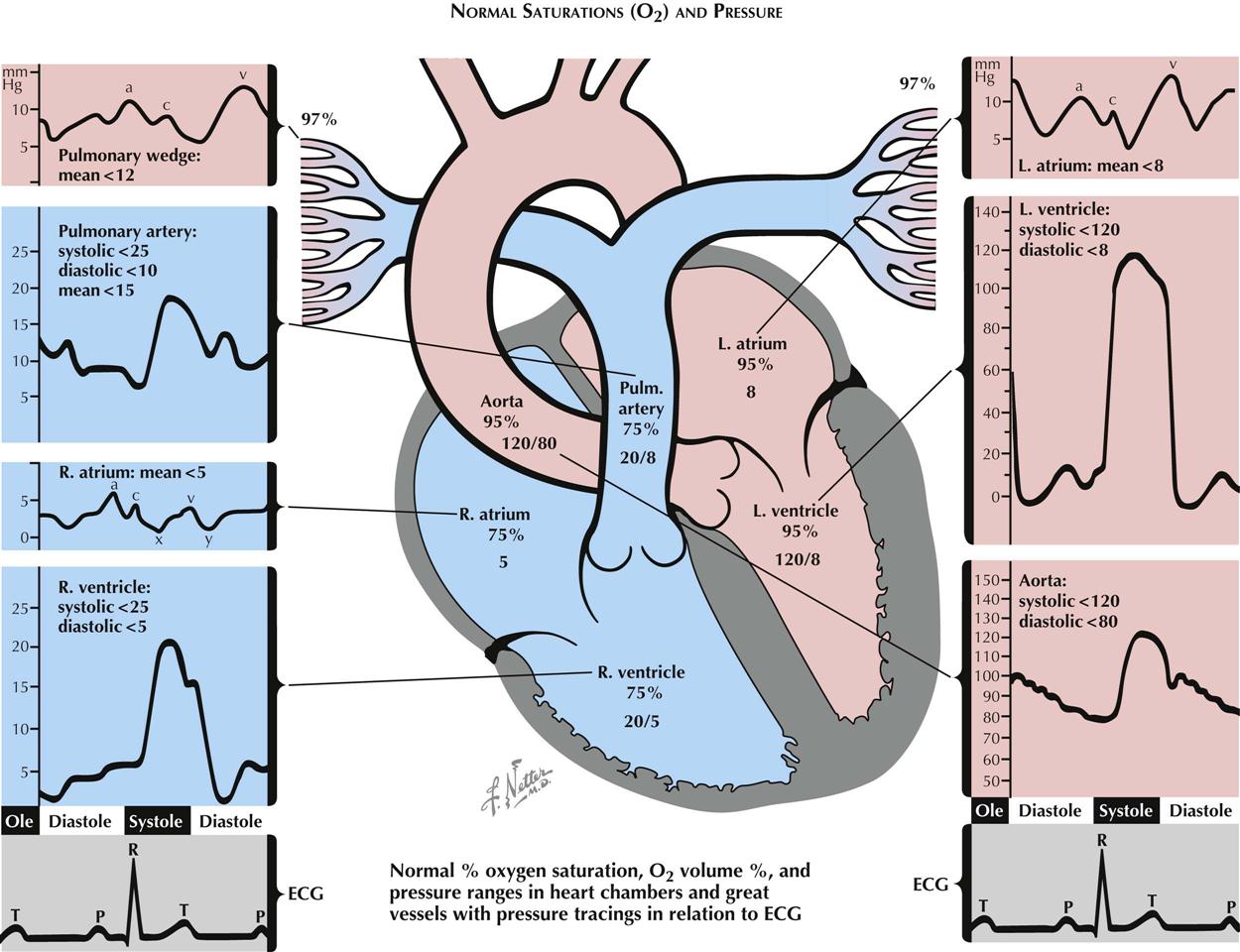
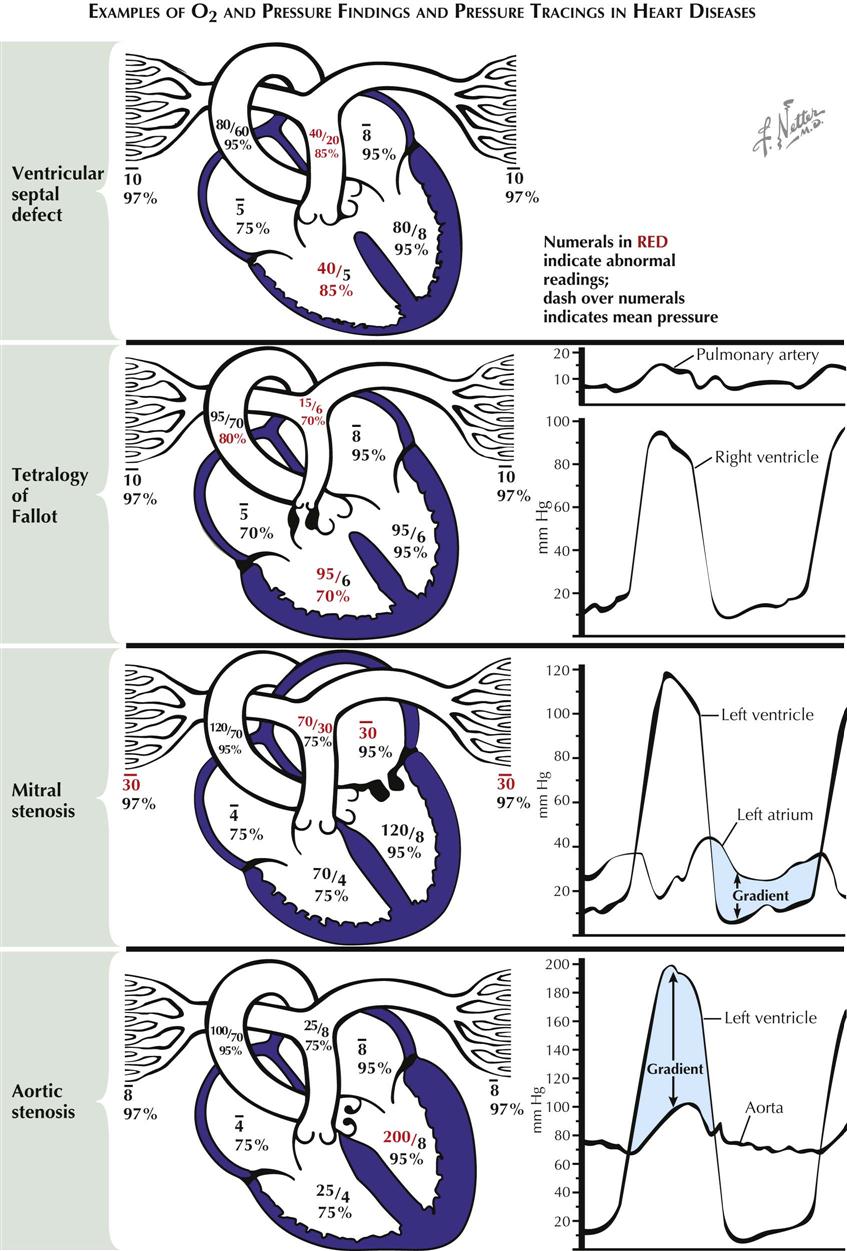
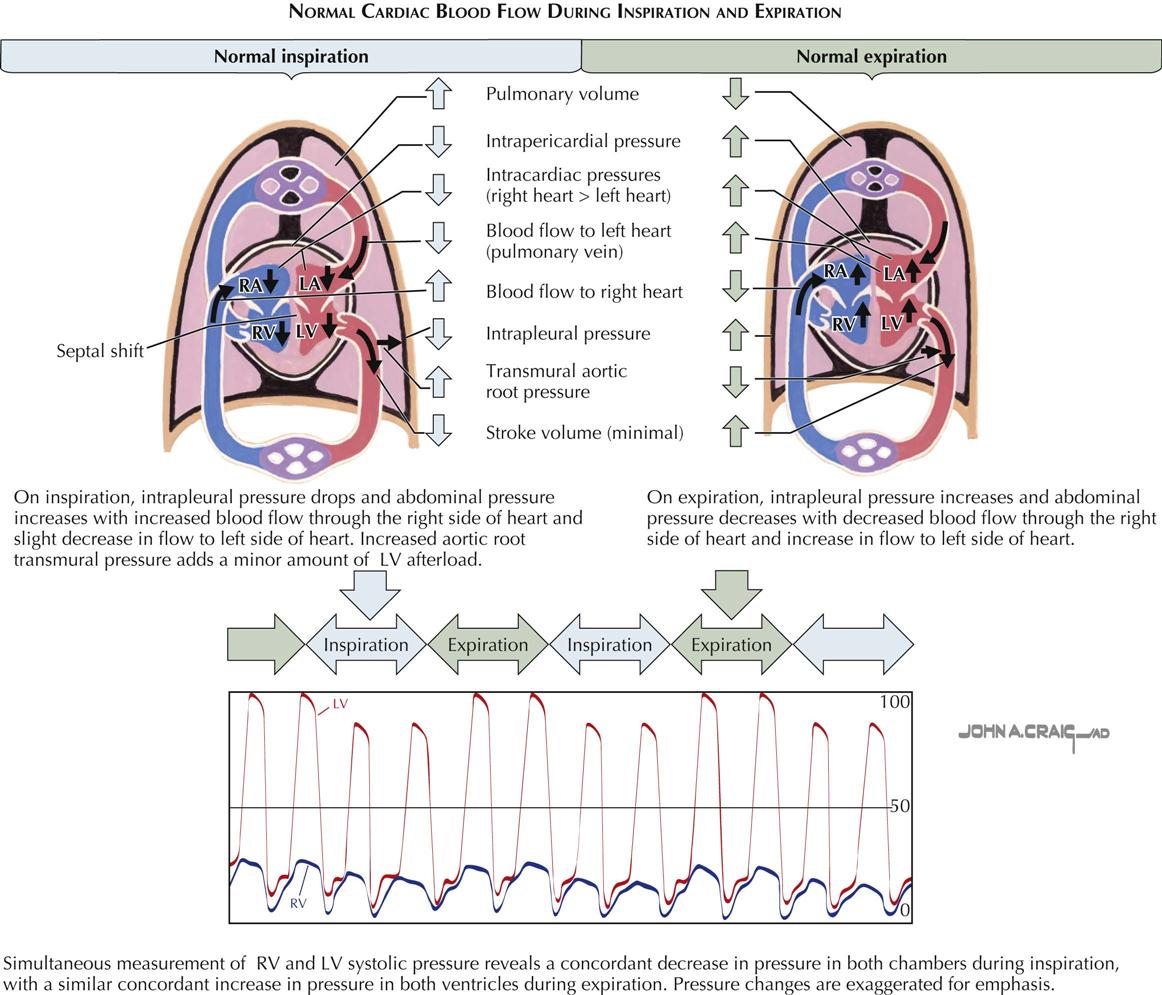
A balloon-tipped pulmonary artery catheter (Swan-Ganz catheter) with a thermistor at the tip (introduced in 1970) floats into the pulmonary artery from the right ventricle as an embolus when the balloon is inflated. The balloon occludes the distal pulmonary branches, and a pressure similar to the PCWP can be measured. When deflated, the catheter measures pulmonary artery pressure, and the thermistor measures a thermodilution curve after injection of 10 mL of cold saline or glucose into the right atrium. The cardiac output can be calculated from the measured thermodilution curve. When the cardiac output is low, the temperature change from right to distal pulmonary artery changes little. When the cardiac output is high, the temperature change is large. Thus the degree of change in temperature is directly proportional to the cardiac output.
Normal Oxygen Saturations and Pressure
In the venae cavae, right atrium, right ventricle, and pulmonary arteries, oxygen saturation (SO2) is normally close to 75% (see Plate 2-10). Small, phasic variations in SO2 of blood sampled from the right-sided heart chambers can be measured. The variation is maximal in the right atrium, where contributions of blood from the renal veins (with a relatively high SO2), from the hepatic veins (with relatively low SO2), from the coronary sinus (with very low SO2), and from the lower inferior vena cava and superior vena cava (with intermediate SO2), meet and start mixing. The mixing is probably complete by the time the blood reaches the pulmonary artery. In the pulmonary wedge position, 97% to 99% saturated blood can be withdrawn through the wedged catheter, approximating the values of pulmonary venous blood. Blood leaving the pulmonary capillary bed is at least 97% saturated. Blood entering the left atrium is slightly less saturated because of its admixture with blood passing through pulmonary arteriovenous and other small shunts.
Normal Intracardiac Pressures
Atrial and Wedge Pressures
The phasic pressures in the right atrium, the left atrium, and the pulmonary artery wedge position (essentially a slightly delayed left atrial pressure) share the same characteristics, with small differences in the amplitude and timing of the phasic features. In normal sinus rhythm the pressure pulse in these chambers is characterized by an a wave produced by the atrial contraction that begins with completion of the atrial P wave in the electrocardiogram (ECG). After a brief delay, the P wave is followed by the QRS signaling the depolarization of the ventricular myocardium. Immediately after depolarization, ventricular contraction begins. The A-V valves close, and the c waves in the atrial pressure curves are produced by changes in the dimensions of the atria and by bulging of the valves into the atria secondary to ventricular contraction. After the c wave, pressure decreases to a low value (the x descent) in response to further atrial volume changes during continued ventricular contraction. During the remainder of systole, continuous venous inflow produces an increase in pressure, the v wave). The peaks of the v waves coincide with the opening of the mitral and tricuspid valves. A pressure decrease in the atria (the y descent) accompanies the transfer of blood from the atria into the ventricles.
Ventricular Pressures
Except for the peak systolic pressure in the left ventricle being approximately five times greater than that in the right, the phasic pressures in the left and right ventricles are similar in contour. The ventricles begin to contract approximately 60 milliseconds after the QRS in the ECG, with the right preceding the left. This action is associated with closure of the A-V valves, resulting in elevated ventricular pressures. During the subsequent period of sequential myocardial contraction, lasting 10 msec and 40 msec for the right and left ventricles, respectively, there are no volume changes, the period of isovolumic contraction.
When the ventricular pressures exceed the end-diastolic pressures in the pulmonary artery and aorta, the semilunar valves open and ejection begins. During the ejection period, the right ventricle and pulmonary artery and the left ventricle and aorta have the same phasic pressures until, systole being completed, the semilunar valves close and the pressures begin to drop in the ventricles. This is followed by the brief period of isovolumic relaxation.
As soon as the ventricular pressures fall below the pressures in the atria, the A-V valves open; and diastole starts and proceeds with venous filling of the common ventricular and atrial chambers, leading to superposable pressures in the atria and ventricles.
Aortic and Pulmonary Artery Pressures
During ejection the ventricular pressures and the pressures in the aorta or pulmonary artery are identical and are characterized by a smooth rise to a peak, then a steady fall to the dicrotic notch, signaling the closure of the aortic and pulmonary valves. This is followed by a steady decrease in pressure as a “runoff” of blood from the arterial system into the venous system occurs through the capillary beds. This is abruptly terminated by the next ejection.
Abnormal Oxygen and Pressure Findings
Ventricular Septal Defect
In ventricular septal defect a shunt of 95% saturated blood is ejected during systole by the left ventricle through the defect into the right ventricle, under the influence of the normally occurring pressure difference between the two ventricles (see Plate 2-11). There the shunted blood contaminates the less-saturated mixed-venous blood. Thus an increased volume of blood with a greater-than-normal SO2 (85%) flows into the pulmonary artery. In the majority of cases the volume of blood shunted depends on the systolic pressure difference between the two ventricles and on size of the defect. The increased SO2 of the blood in the pulmonary artery is in direct proportion to the volume of the shunt. The pressures in the pulmonary artery and right ventricle are usually elevated because of the increased pulmonary vascular resistance, which is secondary to the failure of neonatal involution to take place in the normal prenatal medial hypertrophy of the small arteries. The pressures may be greatly elevated by subsequent intimal and other pathologic changes. Eventually, after development of very high RV pressures, the shunt may be reversed, and desaturated blood may flow from the right to the left ventricle and the systemic arteries.
Tetralogy of Fallot
The basic abnormalities in the tetralogy of Fallot are pulmonary stenosis (valvular or infundibular) interventricular septal defect, disproportion in the diameter between and usually some displacement of the aorta and pulmonary artery, with secondary RV hypertrophy (see Plate 2-11). Because of the pulmonary stenosis, which significantly increases normal outflow resistance, RV hypertension may reach systemic levels. This results in a shunt of unsaturated blood through the defect, with a mild reduction in SO2 in the left ventricle and a greater reduction in the aorta and systemic arteries. The latter causes the cyanosis characteristic of these patients. The greatly reduced pulmonary blood flow reaches full saturation in the lungs. Systolic pressure in the right ventricle reaches the level of the aortic pressure. Distal to the pulmonary stenosis, however, the pressures are lower than normal, and the pressure contour is often distorted.
Mitral Stenosis
The resistance to diastolic flow from left atrium to left ventricle after narrowing of the mitral valve increases left atrial (LA) pressures and eventually reduces LV flow (see Plate 2-11). A pressure gradient across the mitral valve throughout diastole can be demonstrated by simultaneous PCWP measurements or direct LA and LV pressure measurements. This gradient is inversely proportional to the square of the cross-sectional area of the valve orifice and is directly proportional to the square of the volume flow. The gradient is greater with increases in the degree of stenosis and during exercise. The LA hypertension is accompanied by pulmonary venous hypertension, which results in pulmonary hypertension and RV hypertension, increased RV work, and hypertrophy. Diastolic pressures in the pulmonary artery and left atrium are identical until pulmonary vascular resistance is increased because of pathologic changes in the vascular bed, resulting in a gradient between the two pressures. Acute bouts of LA hypertension lead to pulmonary edema, whereas chronic pulmonary artery hypertension may eventually cause RV failure.
Aortic Stenosis
In aortic stenosis, obstruction to the ejection of blood from the ventricle into the aorta, caused by subvalvular, valvular, or supravalvular stenosis, results in abnormally high pressure in the left ventricle and abnormally low pressure in the aorta and thus a systolic pressure gradient across the valve. Progressive obstruction to LV outflow magnifies these effects and leads to LV hypertrophy and eventually acute or chronic LV systolic and diastolic failure (see Plate 2-11).
Effects of Inspiration and Expiration on Intracardiac Pressures and Flow
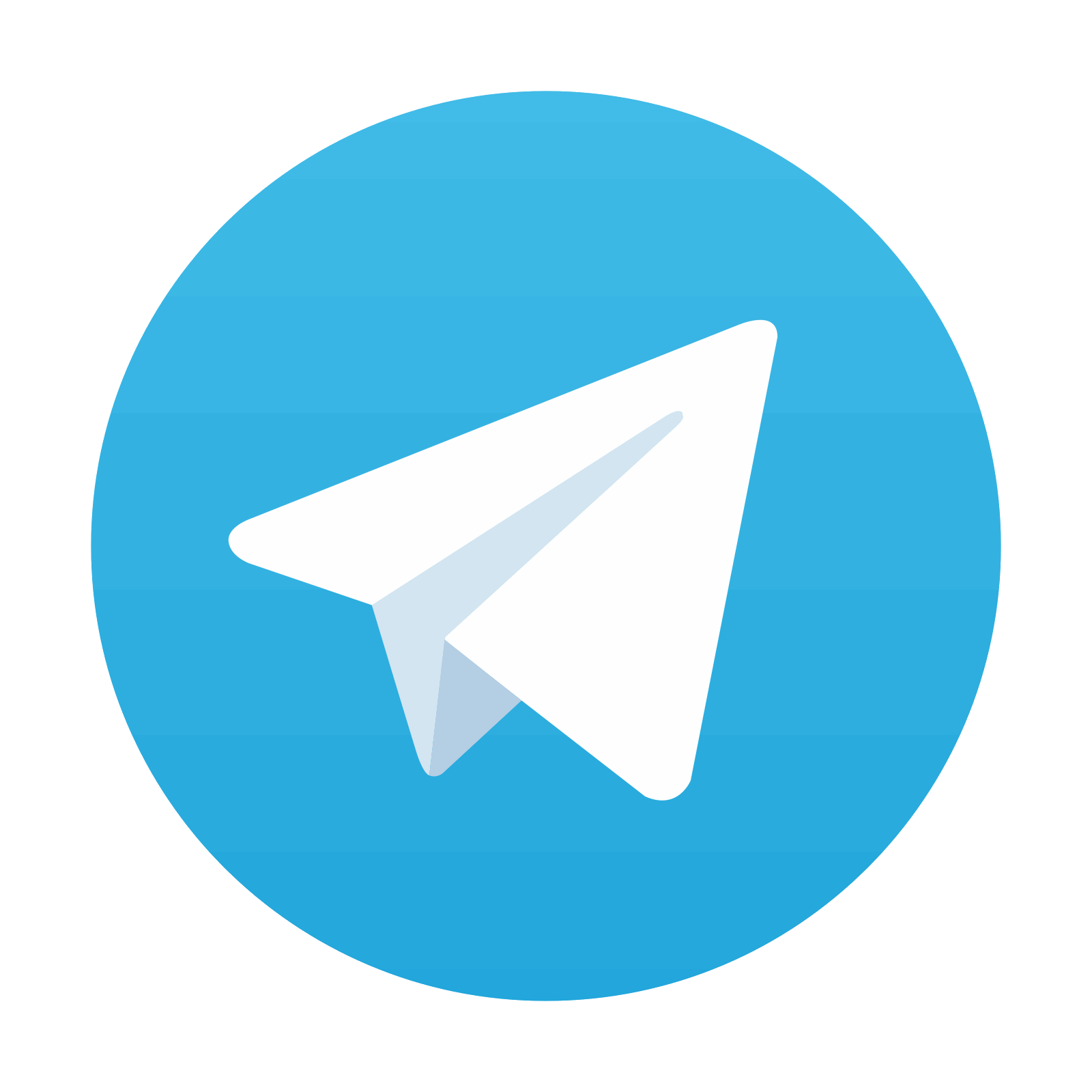
Stay updated, free articles. Join our Telegram channel

Full access? Get Clinical Tree
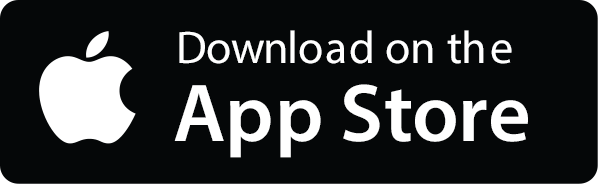
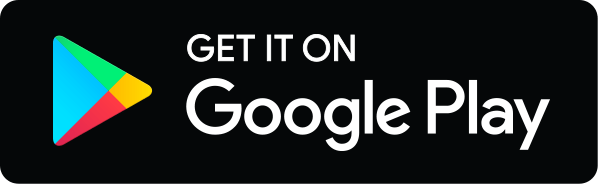