CHAPTER 17 Acute lung injury (ALI) can occur due to direct insult to the lungs or secondary to a systemic inflammatory response. ALI, which can develop over hours to days, causes a diffuse, inflammatory lung injury. ALI can progress to acute respiratory distress syndrome (ARDS) over a period of days. ALI progressing to ARDS has significant morbidity and mortality. Box 17.1 lists common causes of ALI and ARDS. Patients with ALI and ARDS develop acute respiratory failure refractory to supplemental oxygen. They present with severe breathlessness, fever, cyanosis, tachycardia, and hypotension. As the condition worsens, patients display symptoms and signs of multi‐organ failure, which includes delirium, crackles in the lungs, haemodynamic compromise, and renal failure. Serial arterial blood gas sampling will demonstrate worsening hypoxaemia and a metabolic acidosis. A chest‐X‐ray (CXR) typically shows bilateral pulmonary infiltrates. Intubated patients with ARDS develop high peak and plateau airway pressures and increased amounts of frothy, exudative fluid from the lungs with a high neutrophil count. As this is not due to left ventricular failure (LVF), the pulmonary capillary wedge pressure will not be increased. The differential diagnoses of ARDS includes respiratory infection, pulmonary oedema, pulmonary haemorrhage, acute exacerbation of interstitial lung disease, cryptogenic organising pneumonia (COP), acute eosinophilic pneumonia, acute interstitial pneumonia (Hamman‐Rich syndrome), and disseminated malignancy. The diagnosis of ARDS is, therefore, made after excluding the above conditions. Microbiological analysis of bronchoalveolar lavage fluid can point to an infective aetiology and a differential cell count can be helpful in determining the underlying cause. For example, a raised eosinophil count in the fluid may suggest acute eosinophilic pneumonia, while in COP there is a mixed pattern of increased lymphocytes, neutrophils, and eosinophils with a reduction in the number of macrophages. Patients who develop acute respiratory failure secondary to pulmonary haemorrhage will produce blood‐stained secretions, with haemosiderin‐laden macrophages in the lavage fluid, and may drop their haemoglobin (HB) if the haemorrhage is severe. Plasma brain natriuretic peptide level (BNP) < 100 pg ml−1, a normal echocardiogram and a pulmonary capillary wedge pressure of <18 mmHg will exclude pulmonary oedema secondary to cardiac dysfunction. If the aetiology of ARDS is not clear, then a surgical lung biopsy is recommended. This has a complication rate of 39%, but a relatively low mortality rate, and a specific diagnosis resulting in a change in management is made in 60% of cases. Box 17.2 lists the internationally agreed criteria for the diagnosis of ARDS. The severity of ARDS depends on the level of hypoxaemia and is measured in a ventilated patient with a positive end‐expiratory pressure (PEEP) of greater than 5 cm H2O. To measure the PaO2/FiO2 ratio, the PaO2 is measured in mmHg and the FiO2 is expressed as a decimal between 0.21 and 1. The incidence of ARDS is 2–8 cases/100 000 population per year. ALI is more common but is often not recognised. The overall mortality for ARDS is about 50%, but depends on the underlying cause and is greater in patients over the age of 60. For example, mortality may be 35% for ARDS secondary to trauma, 50% when sepsis is the cause and 80% for aspiration pneumonia. Death is usually secondary to multi‐organ failure caused by tissue hypoxia. Survivors often develop chronic pulmonary fibrosis and impaired lung function, although those with ALI who are managed optimally can recover completely, with normal lung function. Insult to the lungs from a direct or indirect aetiology results in an acute inflammatory process. The initial acute inflammatory phase, which causes diffuse alveolar damage, lasts for 3–10 days, and causes widespread endothelial damage and the release of inflammatory cytokines, including IL‐1, IL‐6, and TNF‐α. These cytokines activate neutrophils and monocytes which adhere to the endothelium and the alveolar epithelium and release proteolytic enzymes. These enzymes damage the alveolar‐capillary membrane, resulting in loss of surfactant, increased alveolar‐capillary permeability, alveolar collapse, and pulmonary oedema. The reduction in functioning alveoli causes ventilation/perfusion mismatch, worsening hypoxaemia, and respiratory failure. The alveolar arterial (A‐a) gradient is widened. After the acute inflammatory phase, as ALI progresses to ARDS, there is pulmonary fibrosis with hyaline membrane formation. The lungs become stiffer, with reduced compliance and increased dead space. Microthrombi can form in the pulmonary capillaries and progress to DIC, causing pulmonary vasoconstriction and pulmonary hypertension. Reduced tissue perfusion results in multi‐organ failure. Secondary respiratory and systemic infections can occur. Early recognition of patients at risk of developing ALI is essential. Careful clinical monitoring on the high dependency unit (HDU) or intensive care unit (ICU) is recommended to pick up signs of deterioration, which may be subtle in the early stages. Serial arterial blood gas measurements through an arterial line, the measurement of central venous pressure and left atrial pressure will distinguish between pulmonary oedema secondary to left ventricular failure and ARDS. Careful assessment for signs of sepsis, including blood and urine cultures, as well as bronchial lavage, may be indicated. Chest X‐ray and high‐resolution CT thorax (HRCT) initially show widespread diffuse or patchy infiltrates with evidence of airspace consolidation, particularly in the dependent areas of the lung (Figure 17.1, Figure 17.2). Once ARDS is established, coarse, reticular changes, consistent with pulmonary fibrosis, develop. Figure 17.1 Chest X‐ray showing ARDS. Figure 17.2 High resolution CT scan showing ARDS. Patients with ALI and ARDS are extremely unwell and should be managed in the ICU. Management consists of treating the underlying cause, treating secondary sepsis aggressively, appropriate fluid resuscitation, and oxygenation to reduce the risk of multi‐organ failure. It is essential to avoid excessive intravenous fluid administration and to aim for a central venous pressure (CVP) of <4 mmHg. A combination of diuretics, systemic vasodilators, inotropes, and vasoconstrictors may be required to obtain adequate cardiac output and perfusion pressures at low left‐atrial filling pressures. General supportive care includes optimal nursing care, daily chest physiotherapy to clear secretions, nutritional support with total parenteral nutrition (TPN) to reduce hypoalbuminaemia, avoidance of pressure ulcers, prophylaxis against the development of venous thromboembolism, proton pump inhibitor to prevent gastrointestinal stress ulcers, and correction of anaemia. There is some evidence that medication to reduce body temperature will reduce the catabolic state which can worsen tissue hypoxia and contribute to multi‐organ failure. Deep sedation and neuromuscular blockade are used to treat agitated delirium. This can help to optimise mechanical ventilation by reducing asynchrony with the ventilator and may improve survival in patients with severe ARDS. Nosocomial infection in patients who are intubated and ventilated is a common cause of morbidity and mortality. This can be difficult to distinguish from ARDS, so if any doubt exists, patients should receive broad spectrum intravenous antibiotics. In the initial stages of ALI, high inspired oxygen concentrations can be delivered using CPAP. However, as the condition deteriorates, patients will require mechanical ventilation. As lung compliance decreases secondary to pulmonary fibrosis and consolidation, high peak inspiratory pressures (PIP) and high positive end‐expiratory pressures (PEEP) of >10 cm H20 may be required to achieve normal tidal volumes. The high pressures used in mechanical ventilation can result in ventilator‐induced barotrauma to the normal alveoli, which are easier to inflate. Repeated alveolar collapse and re‐expansion result in atelectasis and damage to the normal alveoli, exacerbating the ventilation‐perfusion (V‐Q) mismatch. Complications of mechanical ventilation include pneumothoraces and the formation of lung cysts. High oxygen concentrations can result in oxygen toxicity. Ventilator‐induced barotrauma is associated with a poor outcome in ARDS. Ventilating at low tidal volumes and keeping the plateau airway pressure to less than 30 cm H2O reduces alveolar over‐distension, recruits collapsed alveoli, is associated with fewer complications, and improves mortality in patients with ARDS. Patients with moderately severe or severe ARDS (PaO2/FiO2 < 200 mmHg) (26.6 kPA) may require a high PEEP. Permissive hypercapnia, resulting from low tidal volume ventilation, is well tolerated. Nursing the patient in a prone position ensures that blood flow is greatest in areas of the dependent lung and improves the VQ mismatch. Extra‐corporeal membrane oxygenation (ECMO) in a specialist centre may be necessary if the usual ventilatory methods fail, although improved survival has not been proven in adults. Inhaled nitric oxide (NO) increases capillary blood flow to the ventilated alveoli and reduces the VQ mismatch, but has not been shown to reduce mortality. Inhaled prostacyclin and prostaglandin E1 have improved physiological parameters in trials but have not been shown to improve survival. There is some debate as to whether corticosteroids given in the initial 7–10‐day period reduce the risk of developing pulmonary fibrosis and influence the long term prognosis of patients with ARDS. Corticosteroids are contra‐indicated in the latter stages of the condition. There is currently limited evidence for the use of exogenous surfactant or antioxidant therapy. β‐2 agonists, N‐acetylcysteine, and ibuprofen have not been shown to be beneficial. Trials looking at human mesenchymal stem cells and recombinant human activated protein C are currently underway. Patients with ARDS die from the underlying cause of the ARDS and secondary complications, particularly nosocomial infection, rather than from respiratory failure. Survivors can take several months to improve. Many are left with persistent cognitive impairment resulting from hypoxic brain injury, myopathy, renal impairment, pulmonary fibrosis, and pulmonary hypertension. Transfusion‐related acute lung injury (TRALI), an important cause of transfusion‐related death, can occur within a few hours of the transfusion of any blood product, although most cases are related to red blood cell transfusion. TRALI occurs when the donor plasma has antibodies to human leukocyte and human neutrophil antigens, and this is more likely to occur when plasma or blood products from a multiparous female donor are used. The recipient neutrophils are sensitised by the underlying clinical condition and are then activated by the anti‐leukocyte antibodies. The true incidence of TRALI is unknown, but is estimated to occur in 0.04–0.1% of transfused patients and in up to 8% of critically ill patients, which equates to 1:5000 transfused blood components. TRALI is more likely to occur in patients who have a high APACHE 11 score, and particularly prevalent in patients who have had surgery, those with sepsis, those who have received a large transfusion, and in alcoholics. Patients developing TRALI become breathless and severely hypoxic during or immediately after transfusion of a blood product. When TRALI is suspected, the transfusion should be stopped immediately and the transfusion service should be notified. Supportive treatment includes oxygenation and appropriate ventilatory support using lung protective strategies. Mortality depends on the patient’s underlying illness and can be up to 60%. Patients who survive usually make a complete recovery. The incidence of TRALI has decreased with the use of plasma products primarily from male donors, female donors without prior pregnancy or those who test negative for HLA antibodies. Acute chest syndrome (ACS) developing during a sickle cell crisis is the most common cause of death in adults with homozygous sickle cell disease (HBSS). Approximately 50% of patients with HBSS will have an episode of ACS, the majority associated with a vaso‐occlusive pain crisis. Patients with other phenotypes of sickle cell disease are at a lower risk of ACS. Most episodes of ACS are secondary to bone marrow ischaemia and necrosis, causing fat emboli and bone marrow to enter into the venous circulation, which mainly affects the lungs and the central nervous system. The fat emboli mechanically obstruct the blood vessels, but also release free fatty acids which cause inflammation and tissue injury. Additionally, there is accumulation of microthrombi in the pulmonary vessels. A diagnosis of fat emboli can be difficult to make. Clinical signs include a petechial rash and lipaemia retinalis. Induced sputum or bronchial lavage will show fat‐laden alveolar macrophages. Bone marrow necrosis and fat emboli can also result in liver and kidney failure. ACS presents with high fever, severe chest pain, dyspnoea, hypoxia, and new radiological changes on chest X‐ray. The differential diagnoses includes pulmonary embolus and pneumonia. Supportive management includes supplemental oxygen, analgesia, intravenous fluids, thromboprophylaxis, antibiotics, bronchodilators, and incentive spirometry to reduce pulmonary atelectasis. Exchange blood transfusion is usually carried out to achieve a haemoglobin S% of 30%. Patients who have more than two episodes of ACS are usually commenced on hydroxyurea and a chronic transfusion programme to keep the HB S level < 50%. Haematopoietic cell transplantation could also be considered for those with recurrent episodes of ACS. There is some evidence that repeated episodes of ACS predispose to the development of interstitial pulmonary disease, historically known as chronic sickle cell lung disease. This can progress to pulmonary hypertension and respiratory failure. There is little evidence that ACS directly causes pulmonary hypertension. Fire kills because of smoke inhalation and severe airway burns. Inhaled toxins, such as chlorine, phosgen, and sulphur dioxide, can cause erythema, oedema, and ulceration of the airways. It should be presumed that all patients with smoke inhalation have been exposed to CO and cyanide, which is formed from the burning of common household compounds, such as nylon, wool, and cotton. Cyanide can kill rapidly by inhibiting aerobic metabolism. Patients who present with suspected smoke inhalation need a thorough assessment of their airway. Clinical features of concern include tachypnoea, erythema, burns to the face and neck and blistering of the oropharynx found at laryngoscopy. Symptoms of cough and wheeze will occur within seconds and respiratory distress will occur within 12–36 hours of exposure. Patients should have serial arterial blood gas measurements of oxyhaemoglobin level and measurement of carboxyhaemoglobin, methaemoglobin, and lactate levels. A toxicology screen should be sent and a CXR obtained, although it is a poor indicator of lung injury. Patients with an unexplained lactic acidosis and a low PaCO2 may have cyanide poisoning. Patients should be given 100% inspired oxygen to reverse the tissue hypoxia and displace the CO and cyanide bound to proteins. The treatment for cyanide poisoning is with sodium thiosulfate and hydroxocobalamin. Nebulised bronchodilators and prophylactic antibiotics are usually prescribed and the patient must be continuously monitored. Patients who suffer with smoke inhalation can develop secondary airway obstruction due to oedema and secretions, develop secondary infections, and can progress to ARDS over days. Patients who develop stridor with worsening airway oedema are at risk of respiratory and cardiac arrest and should be intubated and ventilated. Carbon monoxide (CO) poisoning is common, often undiagnosed, and potentially fatal. It should be considered in all patients presenting with smoke inhalation but is also commoner in the winter months as it often occurs with faulty boilers and poorly ventilated fuel‐burning devices. CO has an affinity for haemoglobin 240 times greater than oxygen, diffuses rapidly across the capillary membrane of the lungs and binds to haemoglobin, forming carboxyhaemoglobin (COHB). CO reduces the amount of oxygen transported to tissues for glycolysis. Patients with CO poisoning present with non‐specific symptoms, including headaches, confusion, nausea, dizziness, and general malaise: the symptoms are often mistakenly thought to have a viral aetiology. Patients are noted to have “cherry red” lips. When severe, patients may present with seizures and a reduced Glasgow Coma Scale (GCS). Doctors should have a high index of suspicion and should take a detailed social history. A neurological examination is essential and evidence of end‐organ damage, which includes cardiac ischaemia, should be sought. Pulse oximetry and blood gas measurements will be normal as these cannot distinguish between oxyhaemoglobin and carboxyhaemoglobin (COHB). COHB measurements can be obtained from a blood gas sample using co‐oximetry. Non‐smokers have CO levels < 3% while smokers may have levels up to 15%. Patients with CO poisoning require continuous monitoring on a HDU or ICU. Management is with 100% inspired oxygen using a non‐breathing face mask. The guidelines recommend hyperbaric oxygen therapy (HBO) if the CO level is >25%, if the CO level is >20% in a pregnant woman, if there is a severe metabolic acidosis with a pH < 7.1, or if there is evidence of cardiac ischaemia on ECG. There is some evidence that hyperbaric oxygen reduces the severity of cognitive defects. Patients who have a reduced GCS should be intubated and ventilated. The local council and fire departments should be alerted to seal off the premises to prevent harm to others. Direct trauma to the airway can occur secondary to any injury to the head, neck, oropharynx, or upper chest. Causes include blunt or penetrating injuries, burns, smoke inhalation, and ingestion of caustic substances. These injuries can result in immediate or delayed airways obstruction. The clinician needs to assess the patient and the airway for signs of obstruction and the likelihood of deterioration. Continuous pulse oximetry monitoring is essential. If oedema or a haematoma are likely to develop, then it is advisable to intubate the patient. Drowning, either in freshwater (90%) or sea water (10%), is a common cause of death worldwide, especially in children. Individuals submerged in water breath‐hold until they exceed the breaking point. Aspiration of water occurs in 85% of cases before laryngospasm and bronchospasm set in. Drowning causes a loss of surfactant in the lung, resulting in atelectasis, and exudative fluid pours into the alveoli. This is exacerbated by the vasoconstriction that occurs in the pulmonary vessels secondary to hypoxia. If the individual is not removed from the water, there is worsening hypoxaemia, hypercapnoea, acidosis, and cardiac arrest. Management is with immediate basic life support, oxygen, and treatment of any hypothermia, Prolonged resuscitation is recommended, especially in children and in those with hypothermia. Unconscious patients will need to be intubated and mechanically ventilated using high PEEP. Those who are conscious and appear to have recovered should be monitored carefully for at least six hours as pulmonary oedema can occur up to four hours after the event. Diving to depths greater than 30 m (98 ft) has significant physiological effects on gas exchange. Divers breathe a mixture of gases, including Heliox. The greater density of gas results in increased airway resistance which increases the work of breathing. There is also a reduction in the maximum breathing capacity and a reduction in pulmonary compliance. In addition, there is an increase in the dead space in the lungs which results in hypoventilation and hypercapnoea. Oxygen exchange in the alveoli is compromised when the gas density exceeds 25 g l−1. Decompression stops during the dive are required to minimise this risk. There is also a risk of oxygen toxicity. Asymptomatic lung rupture can occur in normal individuals, even at sea level, with everyday manoeuvres such as coughing, sneezing, and breath‐holding. These are usually small and have no significant adverse effects. When a diver ascends, the volume of air in the lungs expands according to Boyle’s Law. This can cause pulmonary barotrauma which can result in air embolism which then escapes into the arterial circulation. Divers often do “skip breathing” when they inhale, then pause, then exhale and this may predispose to lung rupture as the lungs are stretched to their elastic limit. There is a risk of developing a tension pneumothorax on ascent secondary to pulmonary barotrauma. Individuals at risk of a pneumothorax, for example, those with chronic lung disease, are advised against deep sea diving. A fit and healthy young adult who has had a spontaneous pneumothorax should be safe to dive if they have had bilateral pleurectomies, have not had a pneumothorax for five years and if a CT thorax and lung function with flow volume loops are normal, as the risk of barotrauma is not significantly greater than that for the general population. An individual who has suffered a traumatic or iatrogenic pneumothorax, for example, after non‐invasive ventilation, should be safe to dive so long as there has been complete resolution as assessed by CT thorax and full lung function tests. Pneumothorax is discussed in Chapter 10. Nitrogen narcosis initially causes a feeling of euphoria which can rapidly progress to decreased consciousness and coma. A rapid ascent can result in decompression sickness or “the bends” when nitrogen dissolved in the blood and tissues forms bubbles and causes dysbaric osteonecrosis of bones, especially in the humerus and femur. Acute altitude or mountain sickness is caused by rapid ascent to altitudes above 2400 m (8000 ft). Although the percentage of oxygen at high altitudes remains at 21%, the partial pressure of oxygen decreases, so climbers become progressively more hypoxaemic. Early flu‐like symptoms, which include headaches, dizziness, fatigue, abdominal cramps, nausea, and vomiting, occur approximately eight hours after ascent. The symptoms usually resolve after two days of acclimatisation. Nosebleeds and insomnia are also common symptoms. Ascent to over 3500 m (11 500 ft) without acclimatisation can result in high altitude pulmonary oedema (HAPE) and high altitude cerebral oedema (HACE), which can be fatal. Climbers with HAPE present with severe breathlessness at rest, fever, and a cough which is initially, dry but then productive of pink, frothy sputum. It is postulated to occur due to pulmonary vasoconstriction secondary to ventilation/perfusion (VQ) mismatch. HACE, which may develop secondary to vasodilation of cerebral blood vessels, presents with severe headaches, neurological symptoms, and can progress to coma and death. Factors which determine the development of HAPE and HACE include rate of ascent, the altitude reached, and an individual’s susceptibility. Acute mountain sickness, HAPE, and HACE can be prevented by ascending slowly, not undertaking strenuous physical activities for 24 hours and avoiding alcohol which can exacerbate the dehydration that occurs at high altitudes due to loss of water vapour. Management of acute mountain sickness includes immediate descent as well as supplemental oxygen, which can be given by a Gamow bag. Acetazolamide, given at 125 mg twice a day, relieves symptoms and improves oxygenation by stimulating the respiratory centre to increase the respiratory rate. Dexamethasone can be beneficial in the short term for HACE, but moving the patient to a lower altitude is essential. Chronic mountain sickness (Monge’s disease) occurs in people living at altitudes above 3000 m (10 000 ft), especially in the Andes in South America. It is linked to expression of genes ANP32D and SENP1. Chronic hypoxaemia causes an increase in erythropoiesis, resulting in polycythaemia (HB > 200 g l−1 and haematocrit >65%), hyperviscosity and VQ mismatch. It can progress to pulmonary hypertension and cor pulmonale which may warrant urgent venesection. Descent to a lower altitude improves symptoms and reverses early physiological changes.
Acute lung injury and acute respiratory distress syndrome
Abbreviations
Acute lung injury (ALI) and acute respiratory distress syndrome (ARDS)
Diagnosis of ALI and ARDS
Incidence and mortality of ALI and ARDS
Pathogenesis of ALI and ARDS
Investigations in patients suspected of ALI and ARDS
Management of ALI and ARDS
Principles of mechanical ventilation in ARDS
Other therapies for ARDS
Morbidity and mortality in ARDS
Transfusion‐Related Acute Lung Injury (TRALI)
Acute chest syndrome with sickle cell disease
Smoke inhalation
Carbon monoxide poisoning
Airway trauma
Near‐drowning
Deep sea diving
Acute altitude sickness
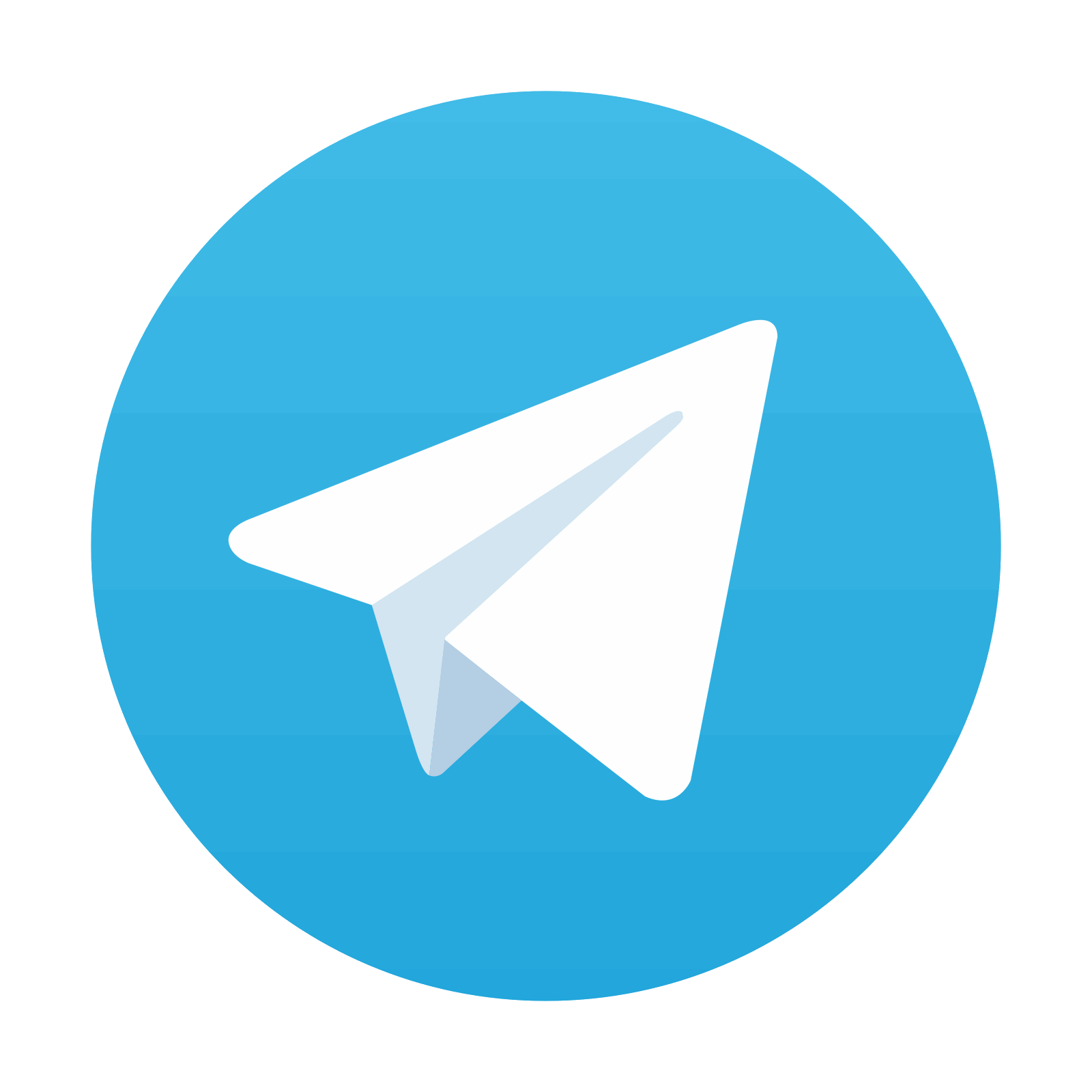
Stay updated, free articles. Join our Telegram channel

Full access? Get Clinical Tree
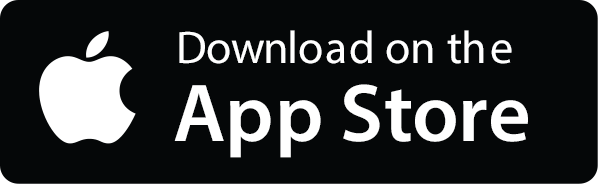
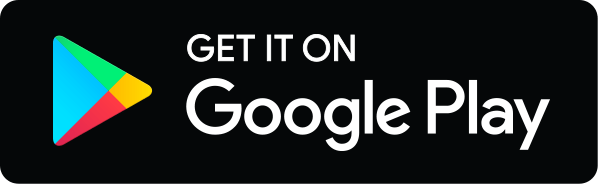