The epithelium is a type of tissue made up of densely packed cells that rest on a basement membrane (Kurn & Daly, 2020). It is one of the five main types of animal tissues—the other four are nerve tissue, connective tissue, muscle tissue, and vascular tissue—and it serves as a covering or lining of various bodily surfaces and cavities. The epithelium may be classified according to the number of its layers as simple, stratified, or pseudostratified (Kurn & Daly, 2020). The endothelium is a special type of epithelium that lines the blood and lymphatic vessels (Aird, 2013). It consists of a single layer of smooth thin cells that form an interface between circulating blood or lymph in the lumen and the rest of the vessel wall (Aird, 2013) (Figure 14.1). The endothelium is characterized as a spatially distributed organ, with a weight of ~1 kg in the average individual that covers a total surface area of 4000 to 7000 m2 (Aird, 2013). Endothelial cells in contact with blood are called vascular endothelial cells, whereas those in contact with lymph are known as lymphatic endothelial cells. Vascular endothelial cells line the entire circulatory system, from the heart to the smallest capillaries, where they perform several fundamental functions (Aird, 2013). Although traditionally considered an inert, static layer in the circulatory system, the vascular endothelium is now acknowledged as a complex organ with important autocrine and paracrine functions, producing a large number of substances that regulate vasodilation, cell proliferation, angiogenesis, and hemostasis (Table 14.1). First of all, endothelial cells form a semipermeable membrane that mediates transfer of ions, solutes, and fluids between the blood and interstitial compartments (Rosenberg & Aird, 1999). As a general rule, gases pass through the endothelium via simple diffusion, whereas ions, solutes, and fluids require convective flow between endothelial cells (paracellular route) or through the endothelial cell (transcellular route) (Rosenberg & Aird, 1999). However, barrier properties differ significantly between different vascular endothelia. For example, the blood‐brain barrier forms a highly efficient barrier due to its tight junctional complexes and paucity of caveolae, which limits both paracellular and transcellular transport, while the liver sinusoidal endothelium possesses a discontinuous basement membrane that is highly permeable (Rosenberg & Aird, 1999). The endothelium also regulates the traffic of leukocytes between blood and underlying tissue. Under normal conditions, constitutive trafficking of lymphocytes from blood to lymph nodes occurs via specialized blood vessels, called high endothelial venules (Chi et al., 2003). In states of inflammation, endothelial cells in postcapillary venules (in nonlymphoid tissue) mediate the adhesion and trans endothelial migration of leukocytes to the extravascular space (Lacorre et al., 2004). This process involves a highly orchestrated multistep adhesion cascade that begins with initial attachment, rolling, arrest, and ends with diapedesis through the endothelium and migration through tissues (Lacorre et al., 2004). This cascade is mediated by specific molecules, such as E‐selectin, P‐selectin, L‐selectin, and endothelial intercellular adhesion molecule‐1 (ICAM‐1). In addition to regulating leukocyte transfer, the endothelium plays other roles in the innate immune response. For example, activated endothelial cells may express and/or release a multitude of inflammatory mediators, such as tumor necrosis factor alpha (TNF‐α), interleukin 1 (IL‐1), and interferon gamma (IFN‐γ). FIGURE 14.1 The endothelium. The endothelial cells form a one‐cell, thick‐walled layer that lines all blood vessels—including arteries, arterioles, venules, veins, and capillaries. Smooth muscle cells layer beneath endothelial cells to form the blood vessel. Table 14.1 Autocrine and paracrine substances released from the endothelium. Source: (Di Francescomarino et al., 2009). The endothelium plays a key role in mediating vasomotor tone. Endothelial cells express several molecules that influence blood vessel diameter and flow dynamics, most notably nitric oxide (NO). The enzyme responsible for endothelial production of NO is endothelial nitric oxide synthase (eNOS), which is modulated by many extracellular signals, including (but not limited to) shear stress and growth factors (Regan & Aird, 2012). Other vasomotor molecules released by the endothelium include carbon monoxide, endothelin 1, epoxyeicosatrienoic acids, and prostaglandins. These molecules regulate the narrowing and enlargement of the blood vessels, usually referred to as vasoconstriction and vasodilation, respectively, and hence the control of blood pressure. Finally, the vascular endothelium has a fundamental role in hemostasis. The endothelium can produce a wide variety of procoagulant and anticoagulant molecules. On the procoagulant side, endothelial cells synthesize plasminogen‐activator inhibitor‐1 (PAI‐1), von Willebrand factor, protease activated receptors, and rarely tissue factor (TF). On the anticoagulant side, endothelial cells express, synthesize, and/or release tissue factor pathway inhibitor (TFPI), thrombomodulin (TM), endothelial protein C receptor (EPCR), tissue‐type plasminogen activator (tPA), and heparan (Aird, 2013). The endothelium normally provides a surface on which blood does not clot due to the enhanced expression of anticoagulant substances; however, in pathological conditions, a shift in the hemostatic balance to one or the other side may result in increased risk of bleeding or thrombosis. Endothelial dysfunction, i.e., the loss of normal endothelial function, is a hallmark of vascular diseases and is recognized as a key early event in the development of atherosclerosis (Hadi, Carr, & Al Suwaidi, 2005; Versari, Daghini, Virdis, Ghiadoni, & Taddei, 2009). Impaired endothelial function is often present in patients with cardio‐metabolic diseases, such as coronary artery disease, diabetes mellitus, chronic kidney disease, and inflammatory diseases (e.g., rheumatoid arthritis and systemic lupus erythematosus) and is predictive of target organ damage, adverse atherosclerotic and thromboembolic cardiovascular events, including myocardial infarction, and cardiovascular mortality (Hadi et al., 2005; Versari et al., 2009). Deleterious alterations of endothelial physiology represent a key step in atherogenesis, and the endothelium is increasingly becoming a surrogate end point of therapeutic approaches to lower cardiovascular risk (Hadi et al., 2005; Versari et al., 2009). Endothelial dysfunction is characterized by reduction of the bioavailability of vasodilators, particularly NO, and/or an increase in endothelium‐derived contracting factors (Lerman & Burnett, 1992). The resulting imbalance leads to an impairment of endothelium‐dependent vasodilation, which is the functional characteristic of endothelial dysfunction. In addition to impaired endothelium‐dependent vasodilation, endothelial dysfunction also comprises a specific state of endothelial activation, which is characterized by a proinflammatory, proliferative, oxidative, and procoagulatory state that favors all stages of atherogenesis (Anderson, 1999). Under normal conditions, the vascular endothelium maintains vascular tone and blood fluidity with no or little expression of proinflammatory factors. However, various traditional and novel cardiovascular risk factors, including smoking, aging, obesity, dyslipidemia, hypertension, hyperglycemia, systemic inflammation, oxidative stress, and genetic predisposition to premature atherosclerotic disease, are all associated with unfavorable alterations in endothelial function. This includes a state of chronic inflammation accompanied by a loss of antithrombotic factors and an increase in vasoconstrictor and prothrombotic products, in addition to abnormal vasoreactivity, therefore elevating the risk of cardiovascular events (Bonetti, Lerman, & Lerman, 2003). Reactive oxygen species (ROS) are generated at sites of inflammation and injury and at low concentrations can function as signaling molecules participating in the regulation of fundamental cell activities, such as cell growth and cell adaptation responses, whereas at higher concentrations, ROS can cause cellular injury and death. The vascular endothelium, which regulates the passage of macromolecules and circulating cells from blood to tissues, is a major target of oxidative stress, playing a critical role in the pathophysiology of several vascular diseases and disorders. Specifically, oxidative stress increases vascular endothelial permeability and promotes leukocyte adhesion, which is coupled with alterations in endothelial signal transduction and redox‐regulated transcription factors, such as activator protein‐1 (AP‐1) and nuclear factor‐kappa B (NF‐κB) (Lum & Roebuck, 2001). Despite its prominent role in cardiovascular health, endothelial function is not easy to assess. This is because (i) few symptoms are directly referable or specific to the endothelium; (ii) the endothelium is hidden from view and not amenable to traditional physical diagnostic procedures; and (iii) the endothelium is not spatially confined and is therefore difficult to image using anatomic imaging methodologies. Table 14.2 presents an overview of available diagnostic markers/procedures for the endothelium (Aird, 2013). To date, the gold standard for diagnosing endothelial dysfunction is the physiologic assessment of vasomotor tone. This can be carried out invasively (e.g., using angiography) or noninvasively (e.g., using imaging or flow studies). The most commonly used diagnostic assay for endothelial function in clinical practice is the noninvasive flow‐mediated vasodilation (FMD), which measures endothelial‐mediated vasorelaxation of the brachial artery in response to the release of external compression (Al‐Qaisi, Kharbanda, Mittal, & Donald, 2008; Corretti et al., 2002; Edmundowicz, 2002). An increase in flow in the brachial artery is achieved by inflation of a pneumatic cuff (placed on the forearm, distal to the ultrasound imaging site) to suprasystolic pressure for 5 min. Upon deflation of the cuff, the increased flow results in shear stress, which activates eNOS to release NO via the L‐arginine pathway. The NO diffuses to the smooth muscle cells causing them to relax, resulting in vasodilatation, and FMD is measured as the percentage change in brachial artery diameter from baseline in response to the increased flow. Noninvasive ultrasound FMD of the brachial artery is an alternate technique also widely used in the literature. Studies have demonstrated that abnormal FMD correlates with coronary artery disease and predicts future disease progression, including acute vascular events (Patel et al., 2004). Therefore, FMD represents a noninvasive measure of pre‐clinical cardiovascular risk in populations with and without obvious risk factors. Table 14.2 Diagnostic procedures and markers for endothelium. Source: (Aird, 2013). Circulating biomarkers for the endothelium include soluble and cell‐based assays. Soluble mediators consist of endothelial‐derived factors involved in hemostasis, cell adhesion, vasomotor and permeability/angiogenesis. Biomarkers for endothelial dysfunction that are released by other cell types include asymmetric dimethylarginine (ADMA), lipoprotein‐associated phospholipase A2, and C‐reactive protein (CRP). Although there are many studies reporting the association of one or another of these mediators in different patient populations, few if any of these markers can consistently and reliably predict the presence/stage of disease, prognosis, or response to therapy in individual patients (Aird, 2013). With regard to cell‐based assays, several types of cells, including circulating mature endothelial cells (CEC), endothelial microparticles (EMPs) and endothelial progenitor cells (EPCs) have been linked to endothelial function; although these cells hold promising value in detecting endothelial damage and predicting its adverse health effects, there is a need for standardization of their quantification and phenotyping methodology and a better understanding of their correlation with underlying disease state (Goon, Boos, & Lip, 2005; Horstman, Jy, Jimenez, & Ahn, 2004; Werner & Nickenig, 2007). The use of noninvasive molecular imaging to visualize biological processes at the molecular and cellular levels within the intact endothelium is another promising diagnostic procedure that remains at an investigational stage (Kaufmann et al., 2007; Kelly et al., 2005). Management of endothelial dysfunction mainly aims at ameliorating endothelial function, through targeting cellular output (i.e., phenotype) and/or intracellular coupling mechanisms (Aird, 2013). Examples of targeting the output include the use of neutralizing antibodies against adhesion molecules, such as ICAM‐1 or VCAM‐1; inducing the expression/synthesis of anticoagulants, such as TM, EPCR, TFPI, and orheparan sulfate; or increasing barrier function (Aird, 2013). Examples of modulating intracellular coupling mechanisms include neutralizing cell surface receptors (e.g., antibodies against the vascular endothelial growth factor [VEGF] receptor) and administration of antioxidants or inhibitors of NFκB signaling (Aird, 2013). There is increasing evidence that certain approved drugs exert their beneficial effect—at least in part—by attenuating endothelial dysfunction. Experimental and clinical studies have demonstrated that a variety of currently used or investigational drugs, such as angiotensin‐converting enzyme inhibitors, angiotensin AT1 receptors blockers, angiotensin‐(1‐7), antioxidants, beta‐blockers, calcium‐channel blockers, endothelial NO synthase enhancers, phosphodiesterase‐5 inhibitors, sphingosine‐1‐phosphate, and statins exert endothelial protective effects (Su, 2015). Given the remarkable capacity of the endothelium to sense and respond to its extracellular environment, it is likely that most if not all systemically administered drugs will alter endothelial phenotype. Due to the difference in mechanisms of action, these drugs need to be used according to specific mechanisms underlying disease‐specific endothelial dysfunction. Nutrition is widely recognized as a strong determinant of cardiovascular health (Chareonrungrueangchai, Wongkawinwoot, Anothaisintawee, & Reutrakul, 2020; Liyanage et al., 2016; Onvani, Haghighatdoost, Surkan, Larijani, & Azadbakht, 2017; Schwingshackl & Hoffmann, 2015; Studer, Briel, Leimenstoll, Glass, & Bucher, 2005). Although data on the relationship between diet and the endothelium are limited, growing evidence suggests that dietary factors play a role in modulating endothelial function. Obesity is considered an important and modifiable risk factor for endothelial dysfunction. A chronic inflammatory state in obesity causes dysregulation of the endocrine and paracrine actions of adipocyte‐derived factors, which disrupt vascular homeostasis and contribute to endothelial vasodilator dysfunction and subsequent hypertension (Engin, 2017; Schinzari, Tesauro, & Cardillo, 2017). While normal healthy perivascular adipose tissue ensures the dilation of blood vessels, obesity‐associated perivascular adipose tissue leads to a change in profile of the released adipo‐cytokines, resulting in a decreased vaso‐relaxing effect (Engin, 2017; Schinzari et al., 2017). Adipose tissue inflammation, NO bioavailability, insulin resistance, and oxidized low‐density lipoproteins are the main participating factors in endothelial dysfunction of obesity (Engin, 2017; Schinzari et al., 2017). Given the close relationship between obesity and endothelial dysfunction, weight loss, achieved through either diet alone or its combination with aerobic exercise, is linked with improved endothelial function as assessed by FMD in overweight and obese individuals (Ades et al., 2011; Bigornia et al., 2010; Brook et al., 2004; Mavri et al., 2011; Pierce et al., 2008; Skilton et al., 2008). This beneficial effect has been attributed to both the favorable metabolic changes associated with weight loss, including improvements in lipidemic profile indices, glucose metabolism, inhibition of thrombosis, amelioration of inflammation, and the degree of weight reduction (Figure 14.2) (Shankar & Steinberg, 2008). The results of available clinical trials are highly encouraging and indicate that endothelial dysfunction is at least partially reversible by weight loss. Thus, it makes sense that all obese individuals with endothelial dysfunction should be encouraged to lose weight, preferably through lifestyle modification, to improve endothelial function and cardio‐metabolic health in general. However, many questions in this topic remain unanswered. First, the optimal degree of weight loss needed for a clinically meaningful improvement in endothelial function has not been determined. Most, clinical trials of improvement in endothelial function associated with weight reduction suggest that a weight loss of ≥10% may be required before an improvement in endothelial function can be detected (Shankar & Steinberg, 2008); nevertheless, even a smaller degree of weight loss, i.e., <5%, has been shown to result in improvements in cardio‐metabolic risk and can be a more suitable, realistic, and sustainable goal for most obese patients in clinical practice, especially for obese patients with high cardiovascular risk or established obesity‐related disorders (Jensen et al., 2014; Yumuk et al., 2015). Moreover, the optimal rate of weight loss for the endothelium remains unknown. If caloric restriction alone was sufficient to improve endothelial function, one should be able to see an early effect in patients undergoing bariatric surgery. If, however, there is persistence in the generation of various mediators responsible for the endothelial dysfunction, these factors may make it difficult to detect any significant improvements in endothelial function for long periods. This is consistent with persistent elevations in the levels of markers of endothelial activation and inflammation for several months after bariatric surgery (Vazquez et al., 2005). Therefore, it is reasonable to postulate that patients with lesser degrees of overweight/obesity, without other co‐morbidities, will experience a significant improvement in endothelial function over time if they achieve significant weight loss, even if the rate of weight loss is not exceptionally rapid. Finally, the macronutrient composition of a diet may affect endothelial function independent of the effect of the weight loss itself. A clinical trial comparing the effects of a low‐carbohydrate versus a low‐fat diet showed that despite similar degrees of weight loss, the low‐fat diet group exhibited a significant improvement in endothelial function, whereas the low‐carbohydrate diet group showed no change in endothelial dysfunction (Phillips et al., 2008). Furthermore, it is highly likely that sodium intake is also reduced in proportion with the caloric restriction. From a vascular function perspective, a reduction in sodium intake is likely to affect endothelial function, either directly or indirectly, and this factor should be considered when evaluating the effects of dietary interventions on vascular function. FIGURE 14.2 Simplified scheme of processes during weight loss that lead to improved vascular function. It is unknown at what degree of weight loss these processes occur and whether they are independent. FFA = free fatty acids. Source: (Shankar & Steinberg, 2008). Data on the effect of dietary components on the vascular endothelium remain scarce and conflicting. Among the various dietary components, dietary fat appears to affect endothelial function in a complex way. With regard to fat quantity, several short‐term feeding trials have shown a detrimental effect of a high‐fat meal on postprandial endothelial function, but this observation has not been confirmed in all available studies (Cuevas & Germain, 2004; Davis, Katz, & Wylie‐Rosett, 2007). Fat quality might also be important for endothelial function; both in vitro and in vivo studies suggest that trans and saturated fatty acids result in impairments in endothelial function, whereas mono‐unsaturated and omega‐3 poly‐unsaturated fatty acids can contrariwise improve endothelial function (Cuevas & Germain, 2004; Davis et al., 2007). Although more research is needed, this observation might suggest that a diet rich in foods with unsaturated fat, such as vegetable oils, nuts, and fish, can be beneficial for the endothelium when compared to foods rich in saturated and trans fat, such as red and processed meat, butter, stick margarine, and high‐processed foods. Besides dietary fat, polyphenols, especially flavonoids, have recently emerged as important non‐nutritive substances for the cardiovascular system. Several clinical trials have demonstrated a significant increase in FMD after the consumption of foods rich in polyphenols, such as tea, grape juice, and red wine (Cuevas & Germain, 2004; Davis et al., 2007); however, it is still unclear whether it is through the effect on endothelial function, their antioxidant properties, or potential anti‐inflammatory properties that polyphenols improve cardiovascular risk. Folic acid has also been attributed with beneficial properties for endothelium; some clinical trials using folic acid supplements have shown improvements in endothelium‐dependent vasodilation, whereas others have not, and this discrepancy may be attributed to the dosage and duration of supplementation (Cuevas & Germain, 2004; Davis et al., 2007). Finally, antioxidants, such as vitamins C and E have also been shown to improve endothelial function in some trials, however, data so far are controversial and inadequate to support antioxidant supplementation as a strategy to manage endothelial dysfunction in clinical practice (Cuevas & Germain, 2004; Davis et al., 2007). As indicated in previous chapters, evaluating the impact of a single dietary factor independently of any other changes in the diet is problematic. In fact, as foods are mixtures of different nutrients and other components that show strong synergistic or antagonistic effects, it is not appropriate to attribute the health effects of a food to only one of its components. Moreover, if total energy intake is kept constant, eating less of one macronutrient implies necessarily eating more of others, and the quality of the replacement can also influence the effect observed. All these limitations require caution in interpreting the results of studies in relation to the effect of a single dietary change on cardiovascular risk. In recent years, nutrition research and epidemiology have focused on the relationship between health/disease on the one hand, and foods and dietary patterns—rather than single nutrients—on the other (Hodge & Bassett, 2016; Hu, 2002; Ocke, 2013; Willett & McCullough, 2008). FIGURE 14.3 Effects of the Mediterranean diet on endothelial dysfunction. ↓ = decrease; ↑ = increase; ⇆ = interacts. Source: (Torres‐Pena et al., 2020 / MDPI / CC BY 4.0). Consistent evidence from epidemiological studies indicates that the adoption of “prudent” or “healthy” dietary patterns, characterized by a higher consumption of fruits, vegetables, nuts, legumes, fish, vegetable oils (especially olive oil), and whole grains, along with a lower/modest intake of red and processed meats, foods rich in refined carbohydrates (sugars), salt, and industrialized processed foods high in trans fatty acids, is associated with a lower incidence of cardiovascular disease and morality (Freeman et al., 2018; Freeman et al., 2017; Mach et al., 2020). With regard to the endothelium, there is also some epidemiological evidence that, regardless of traditional risk factors such as age, smoking, and body weight, individuals who consume a prudent diet exhibit lower levels of soluble E‐selectin, a serum marker for endothelial dysfunction; conversely, adoption of a Western diet, rich in red meat, processed meat, refined grains, and French fries correlate positively with E‐selectin, sICAM‐1, and sVCAM‐1, which are indicative of endothelial dysfunction and a significant role in the pathogenesis of cardiovascular disease (Lopez‐Garcia et al., 2004). Among the various healthy dietary patterns, the Mediterranean diet (MD) is well‐known for its various health benefits, mainly in relation to reducing the risk of all‐cause mortality, cardiovascular disease, cancer, neurodegenerative diseases, diabetes mellitus, and hypertension (Kastorini et al., 2011; Sofi, Macchi, Abbate, Gensini, & Casini, 2014). The MD can be described as a dietary pattern characterized by high consumption of olive oil (as the main edible fat), vegetables, legumes, whole grains, fruits, and nuts, a moderate consumption of poultry and fish, a low consumption of full fat dairy products and red meat, and a low‐to‐moderate consumption of wine as the main source of alcohol accompanying meals (Sofi, 2009). The remarkable health benefits of the MD have been attributed to its strong anti‐inflammatory and antioxidant properties, deriving from the complex synergistic effects of the combination of its individual foods and their nutrients (Bullo, Lamuela‐Raventos, & Salas‐Salvado, 2011; Kontogianni, Zampelas, & Tsigos, 2006). Moreover, a long‐term adherence to the MD is associated with a reduced risk of obesity and better weight control, compared to other dietary patterns (such as a low‐fat diet), when combined with energy restriction (more in Unit 5) (Buckland, Bach, & Serra‐Majem, 2008; Esposito, Kastorini, Panagiotakos, & Giugliano, 2011). Given that obesity, inflammation, and oxidative stress are implicated in the pathogenesis of endothelial dysfunction, the MD could constitute the first‐line dietary pattern for enhancing endothelial function (Figure 14.3) (Torres‐Pena, Rangel‐Zuniga, Alcala‐Diaz, Lopez‐Miranda, & Delgado‐Lista, 2020). This is supported by clinical trials showing significant improvements in FMD by approximately 2% after a dietary intervention based on the MD both in healthy individuals and patients with high cardiovascular risk (e.g., those with dyslipidemia, metabolic syndrome, and diabetes) (Esposito et al., 2004; Fuentes et al., 2001; Shannon et al., 2020).
CHAPTER 14
Endothelial Function
INTRODUCTION
Vasodilators
Vasoconstrictors
Nitric oxide, prostacyclin, endothelium‐derived hyperpolarizing factor, bradykinin, adrenomedullin, C‐natriuretic peptide
Endothelin‐1, angiotensin‐II, thromboxane A2, oxidant radicals, prostaglandin H2
Antiproliferative
Pro‐proliferative
Nitric oxide, prostacyclin, transforming growth factor‐b, heparan sulfate
Endothelin‐1, angiotensin‐II, oxidant radicals, platelet‐derived growth factor, basic fibroblast growth factor, insulin‐like growth factor, interleukins
Antithrombotic
Prothrombotic
Nitric oxide, prostacyclin, plasminogen‐activator, protein C, tissue factor inhibitor, von Willebrand factor
Endothelin‐1, oxidant radicals, plasminogen‐activator inhibitor‐ 1, thromboxane A2, fibrinogen, tissue factor
Angiogenesis
Inflammatory markers
Vascular endothelial growth factor
Cell adhesion molecules (P‐ and E‐selectin, intercellular adhesion molecule, vascular cell adhesion molecule), chemokines, nuclear factor‐kB
ENDOTHELIAL DYSFUNCTION
DESCRIPTION
ASSESSMENT
Procedure/marker
Comments
Pathology
Not readily available except for skin biopsies; results from skin biopsies may not extrapolate to other vascular beds
Soluble mediators
Intercellular adhesion molecule 1
Not specific to endothelial cells
Vascular cell adhesion molecule 1
Not specific to endothelial cells
P‐selectin
Not specific to endothelial cells
E‐selectin
Specific to endothelial cells
Thrombomodulin
Specific to endothelial cells
Tissue plasminogen activator
Not specific to endothelial cells
Plasminogen‐activator inhibitor 1
Not specific to endothelial cells
von Willebrand factor
Not specific to endothelial cells
Endothelial protein C receptor
Specific to endothelial cells
Cell‐based mediators
Circulating endothelial cells
May be quantified and phenotyped; limited by small numbers in blood
Microparticles
May be quantified and phenotyped
Endothelial progenitor cells
May be quantified and phenotyped; hematopoietic in origin
Molecular imaging
Holds promise for vascular bed‐specific diagnosis
Flow studies
Gold standard method in clinical mainstream
MANAGEMENT
NUTRITION AND ENDOTHELIAL FUNCTION
OBESITY AND WEIGHT LOSS
NUTRIENTS AND FOODS
DIETARY PATTERNS
PHYSICAL ACTIVITY AND ENDOTHELIAL FUNCTION
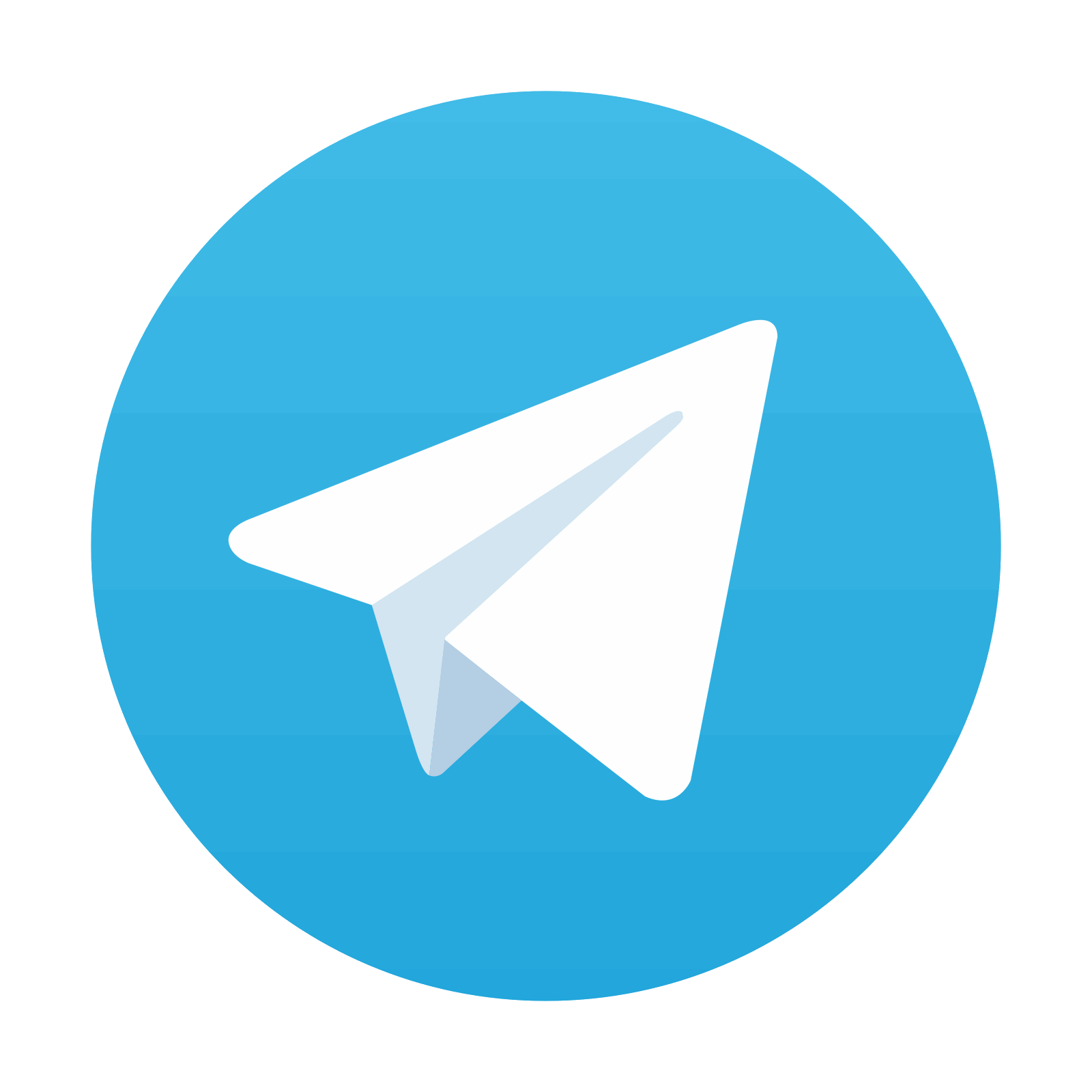
Stay updated, free articles. Join our Telegram channel

Full access? Get Clinical Tree
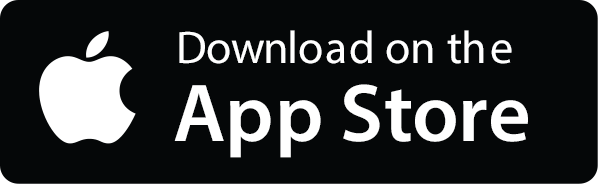
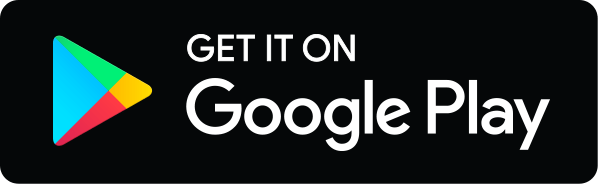