CHAPTER 13 Respiratory failure occurs due to inadequate gas exchange resulting in an abnormally low oxygen (O2) level in blood. It is a potentially life‐threatening condition that can lead to respiratory arrest and death if untreated. It can be categorised into type 1 respiratory failure or type 2 respiratory failure which can be acute or chronic at presentation. The diagnosis of type 1 and type 2 respiratory failure can be made by arterial blood gas (ABG) measurement. The mechanisms for developing these two types of respiratory failure are different (Box 13.1). Table 13.1 depicts the results of a normal ABG measurement and ABG in patients with type 1 and type 2 respiratory failure. Table 13.1 Arterial blood gas measurements. The physiology and control of breathing are discussed in Chapter 2. The respiratory centre consists of neurones in the medulla and in the floor of the fourth ventricle which initiate automated breathing activity under the regulation of chemical and physical reflexes. These can be overridden by voluntary cortical control, for example when sighing or breath‐holding. During inspiration, the inspiratory muscles contract, resulting in an increase in the volume of the thoracic cavity. This generates negative pressure in the alveoli and flow of air into the lungs. During expiration, the intra‐alveolar pressure becomes slightly higher than atmospheric pressure and air flows out of the lungs. The relationship between the partial pressure of oxygen in blood (PO2) and the oxygen saturation measured by pulse oximetry (SaO2) is described as the oxyhaemoglobin dissociation curve. Oxygen saturation is closely related to the partial pressure of oxygen in blood only over a short range of about 3–7 kPa. Above this level the dissociation curve begins to plateau and there is only a small increase in oxygen saturation as PO2 rises. See Chapter 2 for details of the oxyhaemoglobin dissociation curve. CO2 is carried in blood dissolved in plasma and is in equilibrium with the bicarbonate anion (HCO3−), which is an important buffer, ensuring acid–base homeostasis. The Henderson‐Hasselbalch equation shows this: In 1868, Pfluger was the first to show that the CO2 content of arterial blood directly affected breathing in animals. In 1905, Haldane and Priestley established that the respiratory centre was extremely sensitive to small changes in alveolar CO2 concentrations. CO2 readily crosses the blood‐brain barrier, rapidly increasing the concentration of H+ in the cerebrospinal fluid. The chemoreceptors on the antero‐lateral surfaces of the medulla are extremely sensitive to hydrogen ions (H+). A small rise in PCO2, therefore, results in an increase in the concentration of H+ in the cerebrospinal fluid and an increase in ventilation. The peripheral chemoreceptors in the carotid and aortic bodies are also sensitive to CO2 and H+ but are activated only when there is a significant fall in the O2 level of arterial blood (< 60 mmHg or 8.0 kPa). These chemoreceptors do not play an important role in the regulation of breathing under normal physiological conditions. Patients with type 2 respiratory failure have chronically elevated CO2 levels in their blood and in the extracellular fluid surrounding the central chemoreceptors in the respiratory centre. These chemoreceptors, therefore, become relatively insensitive to the raised levels of CO2. Under these circumstances the response to hypoxia by the peripheral chemoreceptors becomes the key stimulant to breathing. Correcting the hypoxia by giving uncontrolled oxygen can prevent the response to hypoxia and worsen the hypoventilation, eventually resulting in respiratory arrest (see Chapter 2). Respiratory failure can occur because of a disturbance of gas exchange at the alveolar level (type 1) or due to failure of the ventilatory muscle pump that enables air to enter and leave the lungs (type 2). Type 1 respiratory failure occurs due to ventilation‐perfusion (V‐Q) mismatch in the lungs, resulting in a reduction in gas exchange (Box 13.2). It is necessary to have an adequate surface area and sufficient blood flow through the pulmonary capillaries to maintain oxygenation. Any condition that results in a reduction in blood flow in the pulmonary arteries (such as pulmonary emboli) or a reduction in the surface area for gas exchange (such as emphysema) will result in type 1 respiratory failure. Patients with type 1 respiratory failure will usually present with breathlessness, increased respiratory rate and symptoms and signs of the underlying cause. This may include wheeze in asthma and crackles in patients with pulmonary oedema or pulmonary fibrosis. If the hypoxia is severe, the patient may appear cyanosed. Initial investigations should include a full history, a thorough examination, pulse oximetry, ABG on air, chest X‐ray, full blood count, urea and electrolytes, and blood glucose. Further investigations can be carried out as indicated by the initial findings and may include a high‐resolution CT scan (HRCT) if underlying fibrotic lung disease is suspected, ventilation‐perfusion (VQ) scan or CTPA if a pulmonary embolus is suspected, or echocardiogram if left ventricular failure or pulmonary hypertension is suspected. The alveolar (A)‐arterial (a) oxygen gradient is the difference between the alveolar concentration of oxygen (A) and the arterial concentration of oxygen (a). It is a more sensitive indicator of disturbance of gas exchange (VQ mismatch) than arterial blood gas measurement alone and can be particularly helpful in patients who appear breathless and are hypoxic without having respiratory failure (PaO2 > 8 kPa). Calculation of the A‐a gradient in a patient with acute type 2 respiratory failure may also be helpful in determining the underlying cause of the lung disease. In a young, healthy individual the A‐a gradient will be around 2 or 3, increasing up to 4 with age. A high A‐a gradient suggests a diffusion defect, a VQ mismatch or a right‐to‐left shunt. In routine clinical practice it is not unusual for the extent of the VQ mismatch to be underestimated because too much reliance is placed on simple oximetry and ABG measurement alone. A slightly reduced PaO2, for example between 10 and 12 kPa, is often ignored. Box 13.3 explains how to calculate the A‐a gradient. It is assumed that the PaCO2 is equal to the PACO2 because carbon dioxide crosses rapidly from the pulmonary vasculature to the alveoli. This equation can be simplified for ease of calculation as follows: Appendix 13.A gives some examples of how to calculate the A‐a gradient. Type 1 respiratory failure can present as an emergency, requiring prompt assessment and treatment. Management of type 1 respiratory failure consists of immediately correcting the hypoxaemia and treating the underlying cause. The aim in type 1 respiratory failure is to maintain the oxygen saturation in the range of 94–98% using a suitable device. These patients are usually not at risk of developing hypercapnoea, so the concentration of oxygen that is required can be safely given, so long as the ABG is monitored regularly. Hypoxaemia (PaO2 < 60 mmHg or 8.0 kPa) must always be corrected to avoid the consequences, which includes respiratory arrest. Oxygen is a drug and must be prescribed, just like any other drug. Oxygen is indicated for all patients with hypoxaemia but is not a panacea for breathlessness in the absence of hypoxaemia except in the palliative care setting. The British Thoracic Society (BTS) Oxygen Guidelines, with agreement from 21 other societies, including the British Association for Emergency Medicine, the British Cardiovascular Society and the Royal College of Anaesthetists, has been disseminated nationally and these should be followed. The BTS oxygen audit is available at http://www.brit‐thoracic.org.uk. It is the responsibility of the doctor to ensure that oxygen has been prescribed, specifying the appropriate target saturation range and the device through which oxygen is to be delivered. Patients who are hypoxic can be given oxygen through a variety of devices. Nasal cannulae are suitable for most patients with type 1 respiratory failure (Figure 13.1). Figure 13.1 Patient using a nasal cannulae. A flow rate of 2–6 L min−1 of oxygen can be delivered, giving a fractional inspired oxygen concentration (FiO2) of between 24 and 50%. The exact FiO2 will depend on the patient’s minute volume, inspiratory flow, and pattern of breathing. Nasal cannulae are cheap, comfortable to wear and well‐tolerated. A simple face mask can also be used for patients with type 1 respiratory failure (Figure 13.2).The flow rate must be set at between 5 and 10 L min−1 to deliver an oxygen concentration of between 35 and 60%. A lower flow rate may result in CO2 build up. A reservoir or re‐breathe mask (Figure 13.3) is used to deliver a higher concentration of oxygen of between 60% and 80% in patients with severe hypoxaemia who are not at risk of retaining CO2. It is often used in patients who are critically ill. Patients who are extremely hypoxic and require very high concentrations of inspired oxygen may benefit from continuous positive airways pressure (CPAP) device which can deliver over 90% of oxygen, often on the high dependency unit (HDU) (Figure 13.4). If oxygenation is inadequate through any of these devices, intubation and ventilation on the intensive care unit (ICU) should be considered. Patients who are at risk of type 2 respiratory failure should be given controlled oxygen via a venturi mask (see section on management of Type 2 respiratory failure). Figure 13.2 Patient using a simple face mask. Figure 13.3 Patient using a reservoir (re‐breathe) mask. Figure 13.4 Patient being fitted with a CPAP device. Nursing staff are responsible for monitoring and documenting the oxygen saturation on the observation chart. In addition, the prescription chart should be signed at each drug round. This is to ensure that the oxygen prescription is being carefully followed and that the amount of oxygen given is adjusted according to the oxygen saturation measurement. It must be remembered that high concentrations of oxygen given over a prolonged period may be harmful, resulting in coronary vasoconstriction, reduced cardiac index, and re‐perfusion injury after a myocardial infarction. It may also have adverse effects in patients who have suffered a stroke. Patients with severe lung disease may be chronically hypoxic and are at risk of developing complications, such as cor pulmonale. They will require long term oxygen therapy (LTOT) at home. These patients should have a formal LTOT assessment, which is usually carried out by a respiratory nurse or physiotherapist. These patients are prescribed the amount of oxygen required (usually 1–4 L min−1) via a concentrator which is installed in their home to use for a specified number of hours. Chapter 3 discusses oxygen as a drug, how it is prescribed and delivered. Type 2 respiratory failure occurs because of failure of ventilation resulting in alveolar hypoventilation. It can be acute or chronic, or present with an acute component overlying the chronic condition. Patients who present with acute type 2 respiratory failure will be symptomatic and unwell. Exacerbation of COPD is the commonest cause of acute type 2 respiratory failure in hospitals in the UK and is associated with significant morbidity and mortality. These patients may develop type 2 respiratory failure in transit to hospital or in the emergency department because they are given uncontrolled oxygen. The uncontrolled oxygen stops the hypoxic drive that the patient is reliant on, resulting in hypoventilation, hypercapnoea, and eventually respiratory arrest. It is therefore essential to be aware of the risk factors for developing type 2 respiratory failure. Box 13.4 lists the common causes of type 2 respiratory failure.
Respiratory failure
Abbreviations
Respiratory failure
Normal
Type 1 respiratory failure
Acute type II respiratory failure
PaO2 mmHg (kPa)
80–100 (10.5–13.3)
<60 (8.0)
< 60 (8.0)
PaCO2 mmHg (kPa)
35–45(4.5–6.0)
35–45 (4.5–6.0)
>48 (6.5)
pH
7.35–7.45
7.35–7.45
<7.35
HCO3− mmol/L
24
22–26
22–26
The regulation of breathing
Mechanisms of respiratory failure
Type 1 respiratory failure
Clinical presentation of type 1 respiratory failure
Investigations in type 1 respiratory failure
Alveolar‐arterial oxygen gradient
Management of type 1 respiratory failure
Oxygen therapy and monitoring in type 1 respiratory failure
Devices for giving oxygen
Chronic type 1 respiratory failure
Type 2 respiratory failure
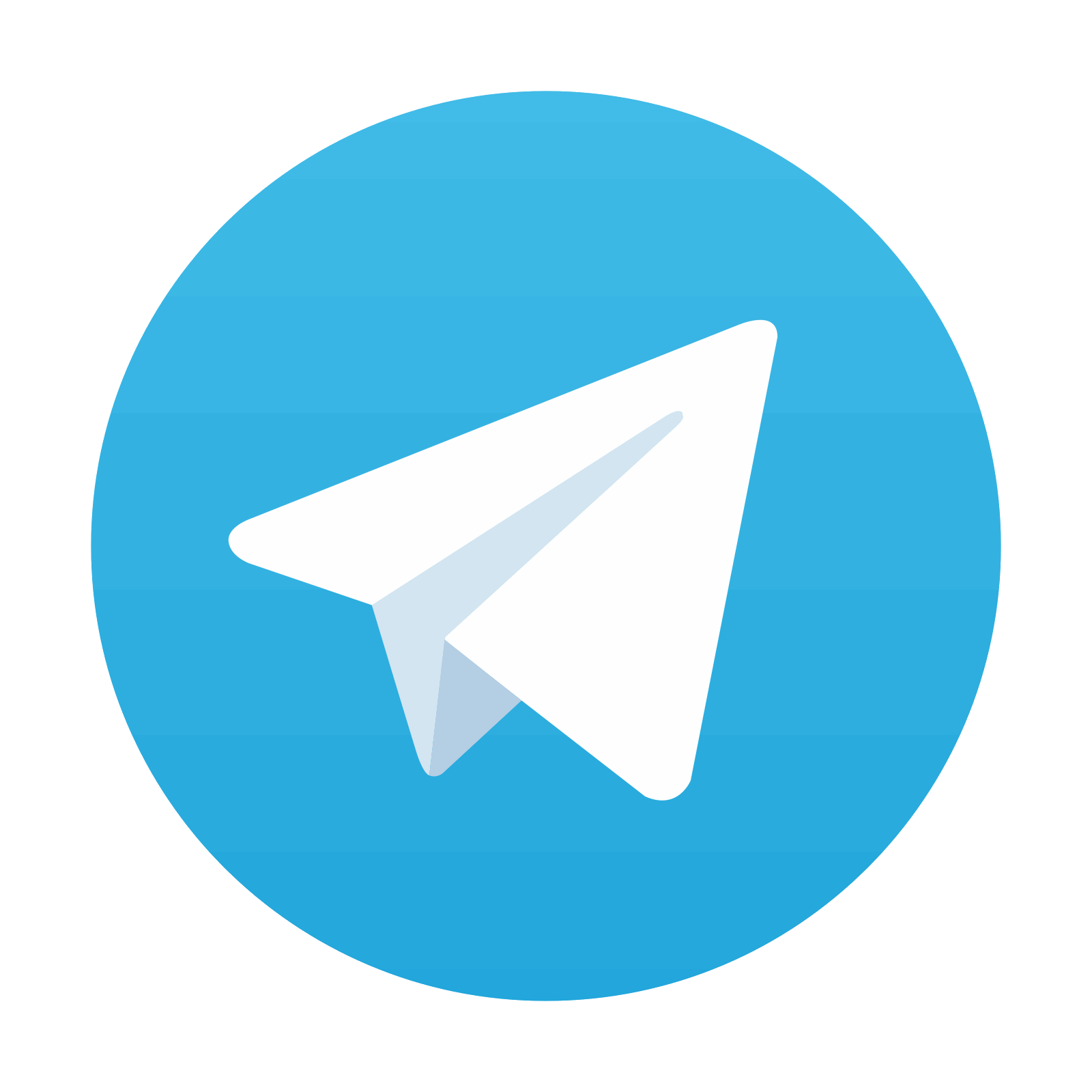
Stay updated, free articles. Join our Telegram channel

Full access? Get Clinical Tree
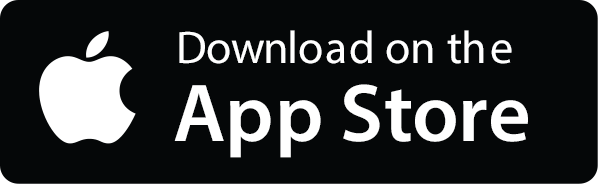
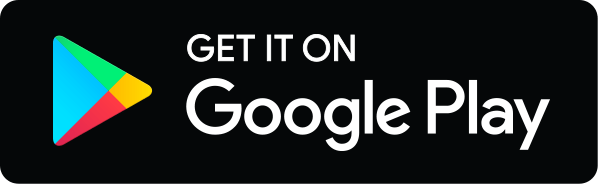