A mechanical ventilator is an automatic machine designed to provide all or part of the work required to move gas into and out of the lungs. The act of moving air into and out of the lungs is called breathing, or ventilation. Devices such as resuscitation bags and T piece resuscitators (for infants) are certainly used to assist breathing, but these devices are not automatic; they require an operator to supply the energy to push the gas into the lungs through the mouth and nose or at least to control the flow direction. Thus, such devices are not considered mechanical ventilators. Automating the ventilator requires three components: There was a time when you could do routine maintenance on your car engine yourself. About that time, a clinician could also completely disassemble and reassemble a mechanical ventilator as a training exercise or to perform repairs. In those days (the late 1970s), textbooks1,2 describing ventilators emphasized individual mechanical components and pneumatic schematics. Today, both cars and ventilators are incredibly complex mechanical devices controlled by multiple microprocessors running sophisticated software. All but the most rudimentary maintenance of ventilators is now the responsibility of specially trained biomedical engineers. Figure 10-1 shows a simplified pneumatic schematic of a current generation intensive care ventilator. FIGURE 10-1 Simplified schematic of a modern intensive care ventilator. High-pressure gas enters the ventilator through the gas inlet connections for oxygen and air (1, 2). Mixing takes place in a reservoir (5) and is controlled by two valves (3, 4). Inspiratory flow from the reservoir is controlled by a separate proportional valve (6). On the inspiratory circuit there is a safety valve (7) and two nonreturn valves (8, 9). In normal operation, the safety valve is closed so that inspiratory flow is supplied to the patient’s lungs. When the safety valve is open, spontaneous inspiration of atmospheric air is possible through the emergency breathing valve (8). The emergency expiratory valve (9) provides a second channel for expiration when the expiratory valve (17) is blocked. Also on the inspiratory circuit are an inspiratory pressure (P) sensor (11) and a pressure sensor calibration valve (10). The exhalation circuit consists of the expiratory valve (17), expiratory pressure sensor (13) with its calibration valve (12), and an expiratory flow (F) sensor (18). The expiratory valve is a proportional valve and is used to adjust the pressure in the patient circuit. Conversion of mass flow to volume (body temperature and pressure, saturated [BTPS]) requires knowledge of ambient pressure, measured by another pressure sensor (not shown). Pressure in the patient circuit is measured with two independent pressure sensors (11, 13). Oxygen flow to the nebulizer port (19) is controlled by a pressure regulator (14) and a solenoid valve (15). Reproduced with permission of Mandu Press, Inc. Our approach to describing ventilator design in this chapter is a major departure from previous equipment books. The focus of this chapter has changed from descriptions of individual components (valves, pistons, circuits, etc.) to a more generalized model of a ventilator as a “black box,” a device for which we supply an input and expect a certain output.3 The reason for the change is that the internal operations of the ventilator are largely unknowable and unimportant to most clinicians. What follows, then, is only a brief overview of the key design features of mechanical ventilators with an emphasis on input power requirements, transfer functions (pneumatic and electronic control systems), and outputs (pressure, volume, and flow waveforms). We will also discuss the interactions between the operator and the ventilator (the operator interface) and various types of ventilator output displays. After this brief introduction to ventilator design, we then change the focus to the interaction between the ventilator and the patient. Ultimately, the application of mechanical ventilation involves selecting a mode of ventilation. The term mode of ventilation means a preset pattern of interaction between the ventilator and the patient. However, the word mode and many other words associated with mechanical ventilation are used without definition and often in illogical ways in both the research literature and ventilator operators’ manuals. Therefore, to understand the technology and terminology associated with modes, in Section 2 we will review 10 basic concepts, starting with how a breath is defined and ending with a classification system for modes of ventilation. The input power for ventilators comes from electricity or compressed gas. Electricity, from either wall outlets (e.g., 110 to 240 volts A/C, at 50/60 Hz) or batteries (e.g., 10 to 30 volts DC), is used to run compressors or blowers of various types. Batteries are a common power source for patient transport or emergency use in hospitals. Alternatively, the power to expand the lungs is supplied by compressed gas from tanks or wall outlets in the hospital (e.g., 30–80 pounds per square inch [psi]). Some transport and emergency ventilators use compressed gas to power both lung inflation and the control circuitry. For these ventilators, knowledge of gas consumption is critical when using cylinders of compressed gas to make sure the source is not depleted before the need for the ventilator (e.g., transport) is over. Ventilators are powered by separate sources of compressed air and compressed oxygen. This permits the delivery of a range of oxygen concentrations to support the needs of sick patients. Hospital wall outlets supply air and oxygen at 50 psi, although most ventilators have internal regulators to reduce this pressure to a lower level (e.g., 20 psi). Because compressed gas has all moisture removed, the gas delivered to the patient must be warmed and humidified to avoid drying out the lung tissue. Input power must be converted to get the desired outputs of pressure, volume, and flow. Electrically powered ventilators use a compressor or blower to generate the required pressure and flow. A compressor is a machine for moving a relatively low flow of gas at ambient pressure to a storage container at a higher level of pressure (e.g., 20 psi). Compressors are generally found on intensive care ventilators. A blower (also called a turbine) is a machine for generating relatively larger flows of gas as the direct ventilator output (rather than filling a storage container) with a relatively moderate increase of pressure (e.g., 2 psi). Blowers are used on home care and transport ventilators. Compressors are typically larger and consume more electrical power than blowers, hence the use of blowers on small, portable devices. Ventilators use different kinds of flow control valves to manipulate the flow of gas from the ventilator to the patient. The simplest valve is a fixed orifice flow resistor that permits setting a constant flow to the external tubing that conducts the gas to the patient, called the “patient circuit.” Manually adjusted variable orifice flowmeters allow the operator to set constant flows in a range of values. This provides a simple means, for example, of adjusting inspired volume during assisted ventilation. Fixed and adjustable flow valves are used in some transport ventilators and resuscitation devices. Inexpensive microprocessors became available in the 1980s and led to development of digital control of flow valves (Figure 10-2). Digital control allows a great deal of flexibility in shaping the ventilator’s output pressure, volume, and flow.4 Such valves are used in most of the current generation of intensive care ventilators.
Section 1: Introduction to Ventilators
Power Inputs
Power Conversion Subsystems
Flow Control Valves
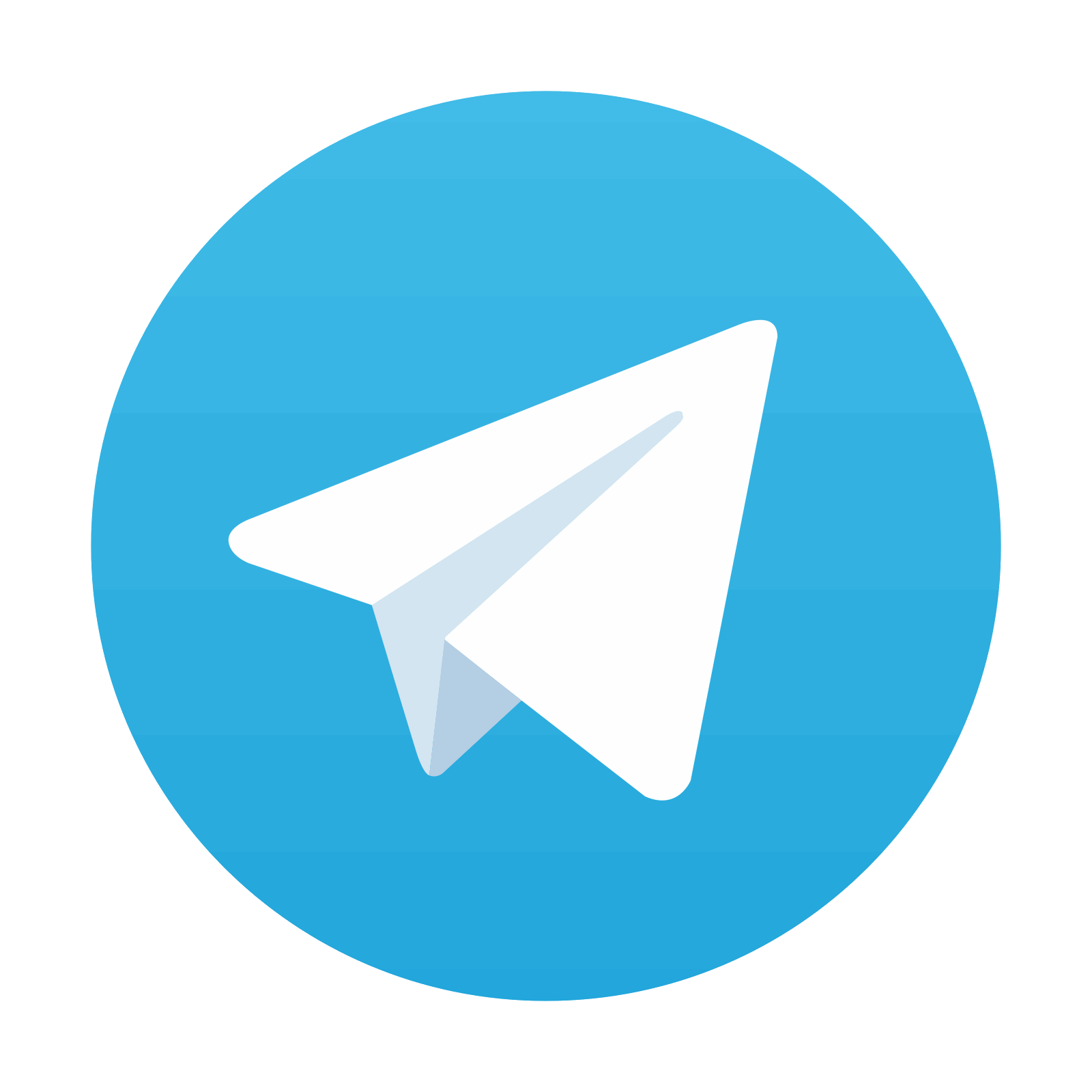
Stay updated, free articles. Join our Telegram channel

Full access? Get Clinical Tree
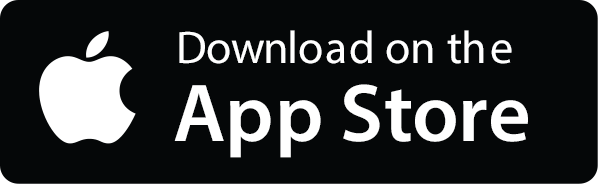
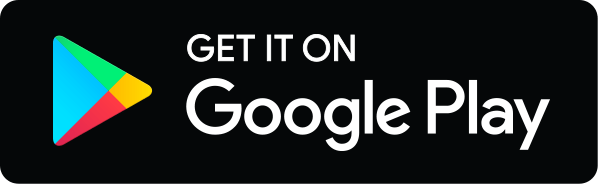