ECMO for Respiratory Support in Adults
Darryl Abrams
Daniel Brodie
INTRODUCTION
The role of extracorporeal membrane oxygenation (ECMO) for adults with respiratory failure has been evolving over the last several decades, most prominently in the context of the acute respiratory distress syndrome (ARDS). However, early versions of the technology, with their high rates of complications, had significantly limited the applicability of ECMO. More recent advances in technology, along with improved management strategies, have favorably impacted both the risk-benefit profile and the consequent survival rates in patients with severe forms of respiratory failure. However, there remains a lack of randomized controlled trials definitively demonstrating the benefit of ECMO over conventional standard-of-care ventilator management. Less severe forms of respiratory failure may likewise benefit from ECMO through the use of smaller cannulae and lower blood flow rates than those typically required for severe ARDS. However, these applications also require more rigorous study before they can be recommended. This chapter reviews both the existing role and the future potential for ECMO in acute and chronic respiratory failure.
CONFIGURATION APPROACHES
ECMO refers to an extracorporeal circuit that directly delivers oxygen to and removes carbon dioxide from the blood via an oxygenator, a gas exchange device with a semipermeable membrane, that selectively permits diffusion of gas into and out of the blood. Deoxygenated blood is drained from a central vein via an external pump, passes through the oxygenator, and is returned to the patient through a reinfusion cannula. When blood is returned to a central vein, referred to as venovenous ECMO, the device only provides respiratory support. When blood is returned to an artery, referred to as venoarterial ECMO, the circuit may provide both respiratory and circulatory support. ECMO, in contrast to cardiopulmonary bypass, most often provides partial cardiopulmonary support.
Oxygenation is primarily determined by the amount of circuit blood flow, the fraction of oxygen delivered through the oxygenator (FDO2), the amount of recirculation, and native lung function. Carbon dioxide removal is principally determined by the rate of gas flow through the oxygenator, known as the sweep gas flow rate, by the extracorporeal circuit blood flow rate, and by native lung function (1). Because extracorporeal circuits are very efficient at removing carbon dioxide and can usually do so at lower blood flow rates than those needed to achieve adequate oxygenation (2,3), smaller cannulae can be used for the purpose of extracorporeal carbon dioxide removal (ECCO2R). These smaller cannulae offer the advantage of being easier and safer to insert (4,5), thus potentially improving the risk profile of ECCO2R over ECMO. ECCO2R may be implemented to correct derangements in carbon dioxide and pH in the setting of acute or chronic hypercapnic respiratory failure (5). Alternatively, it may be useful in eliminating carbon dioxide in the setting of hypoxemic respiratory failure when lung-protective ventilatory strategies result in severe respiratory acidosis. However, ECCO2R delivers very little oxygen to the bloodstream.
Venovenous ECMO traditionally involves cannulation of two distinct venous access points for drainage and reinfusion of blood (1), including cannulation of a femoral vein (Fig. 26.1). When the drainage and reinfusion ports are in close proximity, reinfused oxygenated blood may be taken back up into the circuit, a phenomenon known as recirculation (6). Recirculated blood, which does not contribute to systemic oxygenation, may compromise the circuit’s efficiency. With the advent of bicaval dual-lumen cannulae, venovenous ECMO can be performed through a single venous access site (Fig. 26.2). When performed via the internal jugular vein, for instance, this avoids the need for femoral access and potentially reduces the amount of recirculation when properly positioned (6,7,8,9). Placement is best accomplished under imaging guidance (10), potentially limiting the utility of such a strategy in emergency situations when transesophageal echocardiography or fluoroscopy is unavailable. However, for patients in whom mobilization is anticipated, a cannulation strategy that avoids femoral cannulation is often preferable. The choice of cannula size is based on the patient’s physiologic needs. Particular consideration should be given to the patient’s estimated cardiac output. For a given extracorporeal blood flow, changes in cardiac output will alter the percentage of the
patient’s blood volume passing through the oxygenator, which will have a direct impact on systemic oxygenation.
patient’s blood volume passing through the oxygenator, which will have a direct impact on systemic oxygenation.
A less commonly employed configuration, used primarily for carbon dioxide removal, is arteriovenous ECCO2R, which is a pumpless circuit that uses the patient’s native cardiac output to generate blood flow through an oxygenator (11,12). The potential for complications associated with arterial cannulation, along with an inability to control circuit blood flow, makes arteriovenous ECCO2R less desirable than venovenous cannulation for most ECCO2R clinical scenarios (13). Additionally, because the partial pressure of carbon dioxide in arterial blood is lower than that in venous blood, arteriovenous ECCO2R is inherently less efficient than venovenous ECCO2R.
In patients with impaired gas exchange and significant cardiac dysfunction, a venoarterial configuration may be appropriate to provide both respiratory and circulatory support. Traditionally, venoarterial ECMO involves femoral venous drainage and femoral arterial reinfusion. However, the reinfusion flow in that configuration travels retrograde up the aorta and may meet resistance from antegrade flow generated by the left ventricle, potentially compromising oxygen delivery to the aortic arch if native gas exchange is significantly impaired (Fig. 26.3). In such circumstances, an additional reinfusion cannula may be added to the circuit via a connection of the femoral arterial reinfusion cannula that is inserted into an internal jugular vein (14), thereby taking advantage of the native cardiac output to circulate reinfused oxygenated blood into the right heart and on to the ascending aorta (Fig. 26.4). Of note, some patients with severe respiratory failure may have concomitant right ventricular dysfunction, in which case, it is theoretically possible that
this approach could induce or exacerbate right ventricular volume overload in a subset of these patients. This combination of venous drainage and arterial and venous reinfusion is referred to as venoarterial-venous ECMO. Combining internal jugular venous drainage and subclavian arterial reinfusion (via an end-to-side graft) constitutes an alternative venoarterial cannulation strategy that optimizes upper-body oxygenation (15), although this configuration requires an open-surgical approach in an operating room and would not be appropriate for emergent bedside cannulation (16).
this approach could induce or exacerbate right ventricular volume overload in a subset of these patients. This combination of venous drainage and arterial and venous reinfusion is referred to as venoarterial-venous ECMO. Combining internal jugular venous drainage and subclavian arterial reinfusion (via an end-to-side graft) constitutes an alternative venoarterial cannulation strategy that optimizes upper-body oxygenation (15), although this configuration requires an open-surgical approach in an operating room and would not be appropriate for emergent bedside cannulation (16).
Venovenous ECMO alone often provides adequate gas exchange support for patients with respiratory failure, because improved oxygenation often decreases pulmonary vascular resistance and increases coronary perfusion and right ventricular function (17). However, in patients with pulmonary hypertension independent of hypoxemic vasoconstriction, venoarterial support may be necessary.
POTENTIAL INDICATIONS FOR ECMO
Acute Respiratory Distress Syndrome
ARDS is the indication for which ECMO has been most rigorously studied (1) (Table 26.1). When ARDS is severe enough to require invasive mechanical ventilation (IMV), positive pressure ventilation perpetuates the underlying lung injury (18). Strategies that minimize tidal volumes and airway pressures have been proven to reduce mortality in ARDS (19). These strategies allow for so-called permissive hypercapnia and its attendant respiratory acidosis. However, severe reductions in respiratory system compliance may limit the ability to apply lung-protective ventilation because of the development of unacceptably severe respiratory acidosis (20,21). ECMO, by removing carbon dioxide, potentially facilitates lung-protective ventilation by correcting unsustainable levels of respiratory acidosis that may accompany low tidal volume
ventilation (4,22). Additionally, ECMO may be used as a rescue therapy for patients with refractory hypoxemia despite maximal ventilatory support (1). Potential indications for ECMO use in ARDS have been proposed (1,23), although there are no universally accepted criteria.
ventilation (4,22). Additionally, ECMO may be used as a rescue therapy for patients with refractory hypoxemia despite maximal ventilatory support (1). Potential indications for ECMO use in ARDS have been proposed (1,23), although there are no universally accepted criteria.
Despite the potential for ECMO to alter outcomes in ARDS, early randomized trials failed to demonstrate an effect of ECMO on mortality in severe ARDS, owing in part to high rates of device-related complications (24,25). In the most recent randomized controlled trial involving ECMO for ARDS (Conventional Ventilation or ECMO for Severe Adult Respiratory Failure, CESAR), 180 subjects with the equivalent of severe ARDS were randomly assigned to referral to a specialized center for consideration of ECMO or to ongoing conventional mechanical ventilation, primarily at the originating hospital (26). Those referred for consideration of ECMO had a significantly lower rate of the combined endpoint of death or severe disability at 6 months (37% vs. 53%, relative risk 0.69, p = 0.03). Because this study was pragmatic in design, there are significant limitations to the interpretation of these results, including the fact that only 70% of the control group received a lung-protective ventilation strategy at any time during the study. Additionally, 24% of those in the ECMO-referred group were managed without ECMO, making it difficult to evaluate the effect of ECMO itself on survival. Other nonrandomized observational studies, particularly during the influenza A (H1N1) pandemic in 2009, have shown conflicting results of the impact of ECMO on survival in severe ARDS (27,28). Such discrepancies call attention to the need for prospective randomized data evaluating the effect of ECMO on survival in severe ARDS. The ongoing ECMO to rescue lung injury in severe ARDS (EOLIA) trial prospectively randomizes
patients with severe, refractory ARDS to either ECMO or to optimal conventional management, including the use of neuromuscular blockade and prone positioning (29). Risk stratification models have been proposed to help identify ARDS patients who will derive the greatest benefit from venovenous extracorporeal support (30,31,32,33). Data regarding the neurocognitive, psychiatric, and functional sequelae for those who recover from ARDS after having received ECMO are limited (28,34,35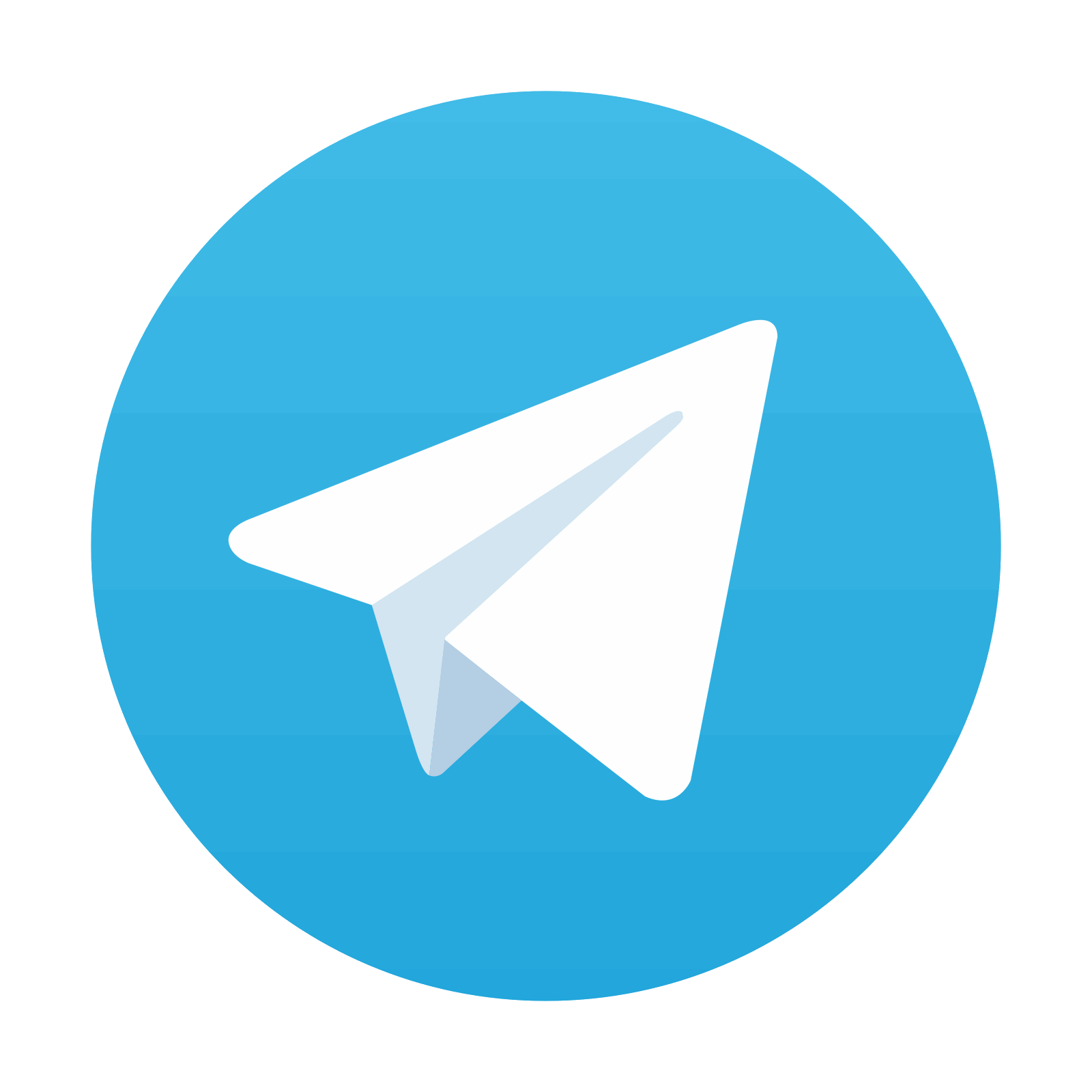
patients with severe, refractory ARDS to either ECMO or to optimal conventional management, including the use of neuromuscular blockade and prone positioning (29). Risk stratification models have been proposed to help identify ARDS patients who will derive the greatest benefit from venovenous extracorporeal support (30,31,32,33). Data regarding the neurocognitive, psychiatric, and functional sequelae for those who recover from ARDS after having received ECMO are limited (28,34,35
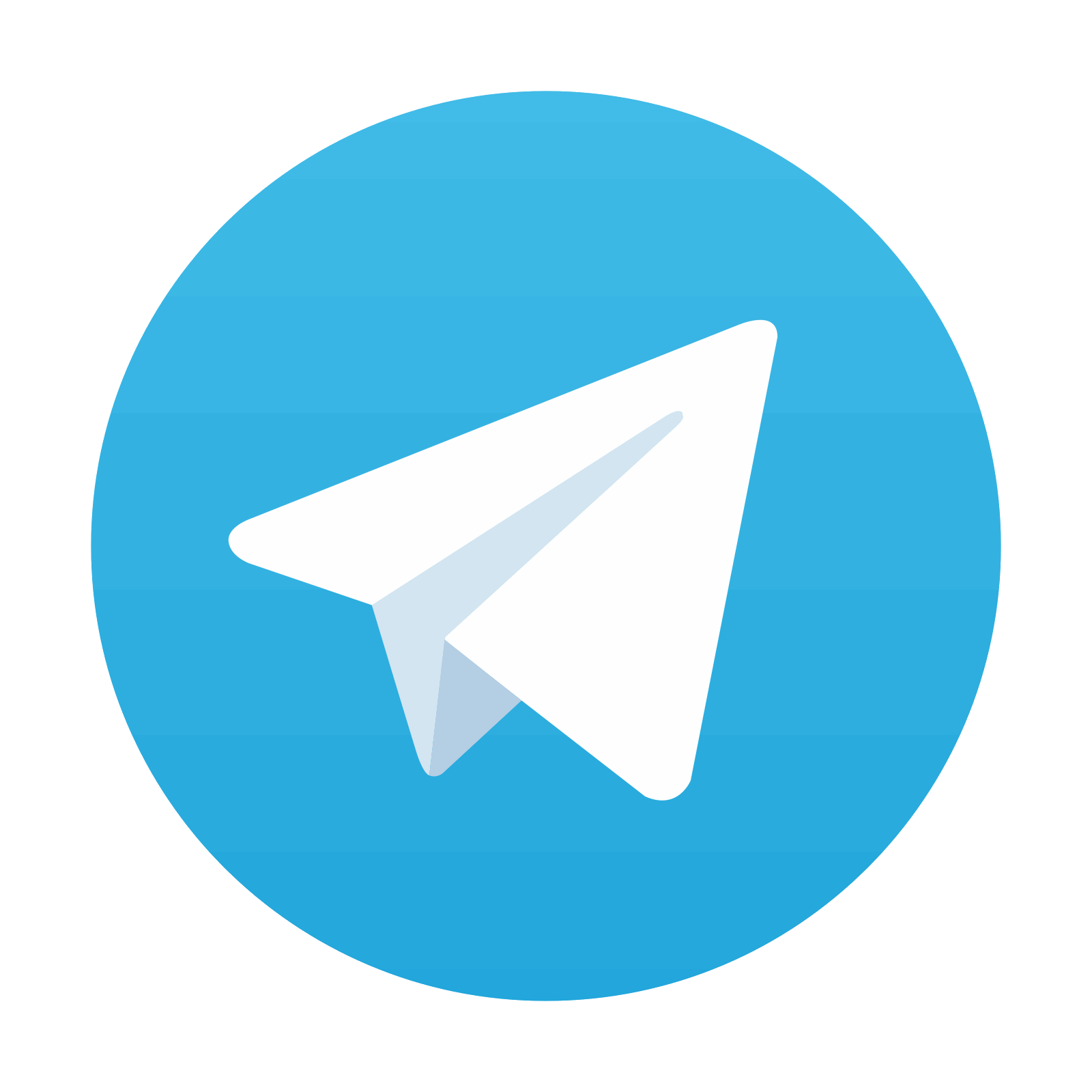
Stay updated, free articles. Join our Telegram channel

Full access? Get Clinical Tree
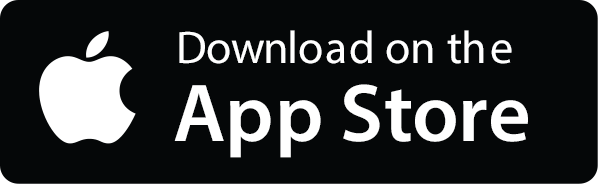
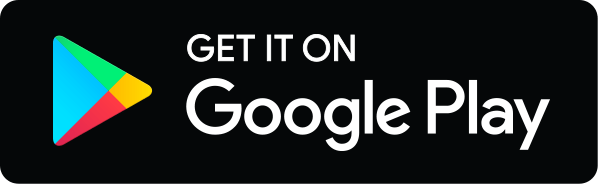
